Physiology 209 Shrier 2024 Lecture Notes PDF
Document Details
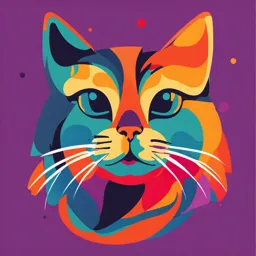
Uploaded by CongenialCarnelian9331
Montreal Neurological Institute
2024
Shrier
Tags
Summary
These lecture notes cover essential concepts in cellular physiology by examining fundamental mechanisms of transport across cell membranes. The various processes including diffusion, osmosis, and active transport are outlined in detail.
Full Transcript
Milieu intérieur or Internal Environment was used by Claude Bernard in 1854 to describe the role of the body in maintaining the uniformity of the conditions of life. So that the external variations are at each instant compensated for and equilibrated. Homeostasis, from the Greek words for "same" an...
Milieu intérieur or Internal Environment was used by Claude Bernard in 1854 to describe the role of the body in maintaining the uniformity of the conditions of life. So that the external variations are at each instant compensated for and equilibrated. Homeostasis, from the Greek words for "same" and "steady," Refers to any process that living things use to actively maintain fairly stable conditions necessary for survival. The term was coined in 1930 by the physician Walter Cannon. In order to preserve the constancy of the Milieu Intérieur and the homeostasis we need to exchange nutrients, salts, gases, and waste in and out of the body. Exchange between Compartments GI tract plasma skin lungs kidneys Interstitial Fluid ISF capillary wall cell membrane (plasma membrane) Intracellular Fluid ICF H2O-60% body wt ICF 28L, 11L ISF, plasma 3L Permeability Characteristics of Cell Membrane Highly Permeable to: H 2O Lipid-soluble substances Dissolved Gases (O2, CO2) Small uncharged molecules Less Permeable to: Larger molecules Charged particles Impermeable to : Very Large molecules Cell Membrane Bimolecular Phospholipid Layer ( Phosholipid Bilayer) polar Cell (plasma) membrane 6-10nM thick nonpolar Amphipathic Polar and Nonpolar ends Phospholipids comprise 40-50% of the plasma membrane by weight. Cholesterol, inserted into the phospholipid bilayer functions as a buffer preventing lower temperatures from inhibiting fluidity and higher temperatures increasing fluidity too much. Cholesterol is also involved in the formation of vesicles that pinch off the plasma membrane and in lipid rafts. Slightly amphipatic. Integral Proteins: Most diverse macromolecules, 25-75% membrane by weight Integral – closely associated with phospholipids, mostly cross the membrane (Trans- membrane) Peripheral – more loosely associated, mostly on the cytoplasmic side Peripheral Glycocalyx is a fuzzy coating that sourrounds the cell membrane formed of glycans, glycoproteins and glycolipids. The glycocalyx contributes to cell-cell recognition, communication, adhesion and protection. It helps to control vascular pemeability. glycoproteins, and glycolipids. Fluid Mosaic Model of Cell Membrane Amino acid transport G-protein receptor Na-K pump Insulin receptor ACh receptor Channels and Transporters Actin Microtubules Septins CD4 T Lymphocytes CD45 Leucocytes CD68 Monocytes CAMs Cadherins Integrins Transmembrane Pathways a. via phospholipid bilayer Channel b. via interaction with a transmembrane protein Carrier Exchanger Transporter Cell Membrane Transport Mechanisms PASSIVE ACTIVE (energy independent) (energy dependent) 1. Diffusion 1. Active Transport a) Primary 2. Facilitated Diffusion b) Secondary 3. Osmosis 2. Pino/Phagocytosis Diffusion Simple diffusion is the movement molecules from one location to another as a result of random thermal motion. Lump of sugar Sugar molecules Flux is the amount of particles crossing a surface per unit time Net flux is from high concentration to lower concentration. At equilibrium, diffusion fluxes are equal and net flux is zero. Diffusion occurs even in the presence of a mechanical partition (membrane) as long as it is permeable to diffusing particles At equilibrium, net movement = 0 Gradient Equilibrium Fig. 04.02 Fig. 04.03 Co = extracellular concentration Concentration C Ci = Co Ci = intracellular concentration 0 0 Time Consider the case of a single cell perfused extracellularly with a physiological solution. At time 0 the physiological solution is changed by adding a molecule at a concentration C0 ,which remains constant. The intracellular concentration Ci is zero at time 0 and increases over time until Ci = C0. FICK’s LAW of DIFFUSION J = PA (C0 – Ci) J Flux: moles of solute crossing a unit area per unit time - rate of diffusion P Permeability (or diffusion) coefficient: a constant based on the ease with which a molecule moves through a membrane A Surface area of the membrane Co – Ci Concentration gradient of the diffusing molecule across the membrane Diffusion time increases in proportion to the square of the distance travelled by the solute molecules. Diffusion is an effective transport process only over short distances. (Einstein's approximation equation) Time for Glucose Diffusion 1 um = 1 msec 10 um = 100 msec 100 um = 10,000 msec Cell Membrane Diffusion 1.Mass of the molecule 2.Concentration gradient 3.Lipid Solubility 4.Electrical charge 5.Ion channels 6.Membrane carriers Diffusion a) Diiffusion of non-polar molecules and gases across the lipid bilayer b) Diffusion of ions through channels Diffusion is related to the concentration gradient Ion Channels Ion channels are transmembrane proteins that show ion selectivity The movement of ions is also affected by the presence of an electrical gradient. The simultaneous existence of an electrical and a concentration gradient for a particular ion is known as an electrochemical gradient. K+ Concentration Gradient - K+ K+ K+ - K+ K+ K+ K+ Intracellular - Extracellular K+ milieu K+ K+ K+ - milieu - K+ K K K + + + K+ - - - 90 mV Electrical Gradient 0 mV The simultaneous existence of an electrical and a concentration gradient for a particular ion is known as the electrochemical gradient. Ion channels can exist in open or closed state as they undergo conformational changes. This is known as gating. Channels may be gated in 3 ways: a. Ligand-gated Cooper b. Voltage-gated Hanrahan, Lukacs Shrier, c. Mechanically-gated Sharif Voltage Gated Ion Channels Na+ channels K+ channels Ca+ channels Cl- channels Current flow through single ion channels depends upon: 1. Channel conductance 2. Channel open time 3. Frequency of channel opening Patch Clamp Experiment Voltage step Evoked single K+ channels Averaged Evoked Single K+ Channels Whole cell K+ Current Mediated Transport Mediated transport is the movement of ions and other molecules (glucose, amino acids) by integral membrane proteins called transporters or carriers. Ion movement across membranes via transporters is much slower than through ion channels. 1. Facilitated Diffusion (Passive) 2. Active Transport 1. Primary Active Transport 2. Secondary Active Transport Characteristics of Mediated Transport a. Specificity – system usually transports one particular type of molecule only b. Saturation – rate of transport reaches a maximum when all binding sites on all transporters are occupied. Thus, a limit – the Tm transport maximum (Tm) – exists for a given substance across a given membrane c. Competition – occurs when structurally similar substances compete for the same binding site on a membrane carrier Factors that Determine Mediated Transport 1. Solute concentration 2. Affinity of transporter for the solute 3. Numbers of transporters 4. Rate of transporter conformational change Mediated Transport Systems 1. Facilitated Diffusion 2. Active Transport a. Primary Active Transport b. Secondary Active Transport Faciltated Diffusion FACILITATED DIFFUSION involves the presence of a “transporter” or “carrier” molecule, which enables a solute to penetrate more readily than it would be expected to by simple diffusion. a) Solute binds transporter b) Transporter changes configuration c) Solute is delivered to other side of membrane d) Transporter resumes original configuration Facilitated Diffusion - transporter (carrier) mediated - passive (no-energy) - net flux from high to low concentration Hormones may increase the number and/or affinity of transporters in some membranes Glut-4 transports glucose in muscle that is increased by insulin Active Transport 1. Transporter-mediated 2. Requires supply of chemical energy (usually derived form enzymatic hydrolysis of ATP) 3. Susceptible to metabolic inhibitors 4. Can transport solute against its concentration gradient (i.e., “uphill transport”) Primary Active Transport Active transport involves the hydrolysis of ATP by a transporter (carrier). Phosphorylation of the transporter changes the conformation of the transporter and its solute binding affinity. Na+/K+-ATPase Na+/K+-ATPase phosphorylation dephosphorylation Changes in the binding site affinity for a transported solute are produced by phosphorylation and dephosphorylation of the Na+/K+-ATPase. Other active transporters Ca2+-ATPase Maintain low intracellular Ca2+ levels H+-ATPase Maintain low lysosomal pH H+/ K+- ATPase Acidification of the stomach Secondary Active Transport In secondary active transport the movement of Na+ down its concentration gradient is coupled to the transport of another solute molecule (ion, glucose, amino acid) uphill against its concentration gradient. Secondary active transport uses the energy stored of the electrochemical gradient to move both the Na+ and the transported solute. The creation and maintenance of the electrochemical gradient depends on primary active transport. 1. Na+ binds to a transporter outside the cell (where the Na+ concentration is high) allowing glucose or amino acid to bind to the same carrier. 2. Through a change in configuration, the transporter “delivers” both Na+ and glucose or amino acid into the cell. 3. The transporter then reverts to its original configuration, and the Na+ is extruded from the cell by the Na+/K+ -ATPase. Secondary Active Transport Mechanisms Symport or Cotransport is when solute X is transported in the same direction as Na+. Antiport, Countertransport or Exchange is when solute X is transported in the opposite direction to Na+. Symport Antiport Na+/HCO3− cotransporter Na+/H+ exchanger (NBC) (NHE) Na+/amino acid cotransporter Na+/Ca2+ exchanger (NAcT) (NCX) Na+/glucose cotransporter (SGLT) Summary of Transport Mechanisms Fig. 04.15 Endocytosis and Exocytosis Active transport mechanisms (energy-dependent) involving participation of the cell membrane itself. Endocytosis – the cell membrane invaginates and pinches off to form a vesicle. Exocytosis – an intracellular vesicle fuses with the cell membrane, and its contents are released into the ECF. Endocytosis Exocytosis Exocytosis Excocytosis is the process of moving material from the inside to the outside of the cell. Types: 1) Constituitive Exocytosis Non-regulated. Functions to replace plasma membrane, deliver membrane proteins to the cell membrane and to get rid of substances from the cell. 2) Regulated Exocytosis Tends to triggered by extracellular signals and the increase of cytosolic Ca2+. Responsible for the secretion of hormones, digestive enzymes, and neurotransmitters. Endocytosis 1. Pinocytosis also known as fluid endocytosis, involves an endocytotic vesicle that engulfs the extracellular fluid including whatever solutes are present. It is nonspecific and constituitive. The vesicles travel into the cytoplasm and fuse with other vesicles such as endosomes or lysosomes. 2.Phagocytosis is the process by which cells bind and internalize particulate matter (>0.75 µm) such as small-sized dust particles, cell debris and microorganiisms. It is specific and triggered. Extensions of the cell membrane called pseudopodia fold around the particle and fully engulf it. The pseudopodia fuse to form large vesicles, called phagosomes, that pinch off the membrane. Phagosomes migrate to and fuse with lysosomes where the contents of the phagosome are degraded. Defend against infection and scavenge senescent and dead cells. Golgi Nonspecific Nucleus apparatus uptake of Ligand solutes Receptor Nucleus and H2O Solutes Vesicle Clathrin proteins forming a clathrin- Unbound Plasma coated pit ligand membrane Vesicle Lysosome Extracellular fluid Clathrin proteins (a) Fluid endocytosis being released from vesicle Bacterium Receptor Nucleus Lysosome Endosome Receptor recycled Cytosol to membrane Vesicle formation Pseudopodia (c) Receptor-mediated endocytosis Phagosome Extracellular fluid (b) Phagocytosis Involves macrophages, neutrophils and dendrtitic cells Receptor-mediated Endocytosis In receptor-mediated endocyosis molecules in the extrtacellular fluid (ligands) bind with high affinity to specific protein receptors on the plasma membrane. a. Clathrin-dependent receptor-mediated endocytosis. When the ligand binds the receptor undergoes conformational change and clathrin is recruited to the plasma membrane. Adaptor proteins link the ligand-receptor to the clathrin. The complex forms a cagelike structure that leads to the aggregation of ligand bound receptors. A clathrin coated pit is formed which then invaginates and forms a “clathrin-coated vesicle”. Once the vesicle pinches off it sheds the clathrin coat and vesicles can fuse with the membrane of cellular organelles such as endosomes and lysosomes. Or, they can sometimes fuse with the membrane on another side of the cell (transcytosis). Receptors and clathrin protein are recycled back to the cell membrane. An example is the LDL receptor. Copyright © McGraw-Hill Education. Permission required for reproduction or display. Golgi Nonspecific Nucleus apparatus uptake of Ligand solutes Receptor Cholesterol is transported in the and H2O Nucleus bloodSolutes as lipid-proteinVesicle particles Clathrin proteins known as low-density lipoproteins forming a clathrin- Unbound Plasma coated pit ligand (LDL). The lipoprotein membrane is recognized Vesicle by PM LDL receptors and Lysosome endocytosis Extracellular fluid follows. Clathrin proteins (a) Fluid endocytosis being released from vesicle Bacterium Receptor Nucleus Lysosome Endosome Receptor recycled Cytosol to membrane Vesicle formation Pseudopodia (c) Receptor-mediated endocytosis Phagosome Extracellular fluid (b) Phagocytosis b. Potocytosis is the process by which molecules are sequestered and transported by tiny vesicles called caveolae. These vesicles are clathrin-independent. Caveolae can deliver their contents directly into the cell cytoplasm as well as to the endoplasmic reticulum (ER) or other organelles and to the plasma membrane on the opposite side of the cell (transcytosis). Potocytosis has been implicated in uptake of low molecular wieght molecules such as vitamins. DIFFUSION of WATER Water diffuses freely across most cell membranes This is facilitated by groups of proteins (aquaporins) that form water permeable channels (Peter Agre) Osmosis Osmosis – the net diffusion of H2O across a semipermeable membrane (i.e., permeable to solvent, but not to all solute) 1L 1L M.W. H2O = 18g/mol 2M 53.5 M 55.5 M 1 L H2O = 1000 g Glucose H2O H 2O 1000/18 = 55.5 M Osmosis 53.5 M 55.5 M H2O H 2O Osmotic Pressure The pressure required to prevent the movement of water across a semi-permeable membrane is referred to as the osmotic pressure. This pressure is equal to the difference in the hydrostatic pressures of the two solutions. In an ideal solution, the osmotic pressure is related to temperature in the same way as the pressure of a gas P = nRT/V Van’t Hoff Equation for osmotic pressure n= # of particles, R = gas constant, T = abs., temp, V = volume Thus the osmotic pressure is proportional to the number of particles in solution/unit volume and not to their size, configuration or charge. Osmolarity Osmolarity (Osm) is the total solute concentration of a solution. 1 osmol = 1 mol of solute particles 1 mol glucose = 1 osmol of solute 1 mol NaCl = 1 mol Na+ + 1 mol Cl- = 2 osmol 1 mol MgCl2 = 1 mol Mg2+ + 2 mol Cl- = 3 osmol Osm = osmol/liter 1 mol glucose/L = 1 osmol/L = 1 Osm 1 mol NaCl/L = 2 osmol/L = 2 Osm 1 mol MgCl2/L = 3 osmol/L = 3 Osm Osmotic pressure is proportional to osmolarity (Osm) Osmotic Pressure of Physiological Saline 1.Determine Molarity 0.9% saline = 0.9 NaCl /100g H2O = 9.0 g NaCl/L H2O (L=1000g) Moles = 9g NaCl x 1 mole /58.5 g NaCl = 0.15 moles NaCl Molarity = moles/L = 0.15 moles NaCl/L = 0.15 M NaCl solution 2. Determine Osmolarity 0.15 M NaCl solution = 0.15 moles Na+ + 0.15 moles Cl- = 0.15 osmol + 0.15 osmol = 0.30 osmol = 0.30 osmol/L H2O = 0.30 Osm = 300 mOsm 3. Calculate Osmotic Pressure Osmotic pressure = 0.30 Osm x 22.4 atm/Osm = 6.7 atm = 6.7 atm x 760 mmHg/atm = 5092 mm Hg When ISF is 3 mOsm> ICF osmotic pressure gradient is 50.92 mm Hg Osmolarity Solutions which have the same osmolarity (concentration of osmotically active particles) as normal extracellular (or intracellular) solution (300 mOsm) are called ISOSMOTIC Solutions which have an osmolarity lower than 300 mOsm are called HYPOOSMOTIC Solutions which have an osmolarity greater than 300 mOsm are called HYPEROSMOTIC To be effective in exerting a sustained osmotic pressure, particles must not be able to cross the membrane and are referred to as nonpenetrating. Extracellular Na+ behaves as a nonpenetrating solute because the Na+ that moves into the cell is pumped out by the Na-K ATPase. Tonicity a. If the solution has a concentration of 300 mOsm of nonpenetrating solute particles, there will be no net shift of water. ISOTONIC solution b. If the solution has a concentration of nonpenetrating solute particles less than 300 mOsm, water will enter the cell and the cell will swell. HYPOTONIC solution c. If the solution has a concentration of nonpenetrating solute particles greater than 300 mOsm, water will leave the cell and the cell will shrink. HYPERTONIC solution Intracellular Extracellular Fluid (ICF) Fluid (ECF) Normal cell 20 mM urea penetrating 140 mM NaCl nonpenetrating Initial 20 mOsm urea 280 mOsm Na+ + Cl- 300 mOsm 300 mOsm Isoosmotic penetrating 20 mOsm urea 20 mOsm urea Equilibrium/ Final 300 mOsm 280 mOsm Na+ + Cl- 280 mOsm H 2O Cell Swelling Hypotonic Total 300 mOsm 300 mOsm CONDITION ECF ECF Vol. ICF Osm. ICF Vol Osm. Excessive H2O intake iv infusion of 0.9% NaCl = = = Hemorrhage = = = Drinking sea water Severe sweating Capillaries An adult has ~40 km of capillaries Capillaries contain ~5% of total circulating blood Each capillary is ~1mm long, inner diameter ~8 µm CAPILLARY WALL A single layer of flattened endothelial cells and a supporting basement membrane. CAPILLARY STRUCTURE and PERMEABILITY 4. Bulk flow 2. 1. 3. TRANSPORT ACROSS CAPILLARY WALL 1, 2- DIFFUSION through across cell membrane is the most important means of transport. Diffusion also occurs through water filled channels (intercellular clefts and fused vesical channels). 3. Transcytosis– Endocytosis on the luminal side followed migration of the vesicle across the cell and then exocytosis on the interstitial side. 4. BULK FLOW distributes the extracellular fluid volume between the plamsa and ISF. Magnitude of bulk flow is proportional to the hydrostatic pressure difference between the plasma and the ISF. Caplillary wall acts as a filter that permits protein free plasma to move from caplillaries to the ISF.