Medical Imaging Systems Part 1 - X-ray Production PDF
Document Details
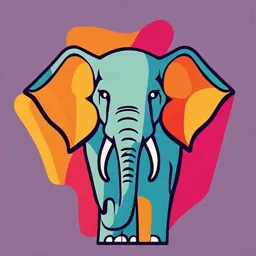
Uploaded by FeistyNebula8189
Yarmouk University
Tags
Summary
This document details medical imaging systems, focusing on the first part that discusses X-ray production, including X-ray tubes, Bremsstrahlung radiation, and Characteristic X-ray Spectrum. It covers key aspects of X-ray technologies.
Full Transcript
Medical Imaging Systems Part 1 X-ray prod., tubes & generators X-Ray tubes Bremsstrahlung radiation (braking radiation) Bremsstrahlung Energy Spectrum What does kVp mean ? x-ray production efficiency Major factors that affect x-ray production efficiency include the...
Medical Imaging Systems Part 1 X-ray prod., tubes & generators X-Ray tubes Bremsstrahlung radiation (braking radiation) Bremsstrahlung Energy Spectrum What does kVp mean ? x-ray production efficiency Major factors that affect x-ray production efficiency include the atomic number of the target material and the kinetic energy of the incident electrons. The approximate ratio of radiative energy loss caused by bremsstrahlung production to collisional (excitation and ionization) energy loss within the diagnostic x-ray energy range (potential difference of 20 to 150 kV) is expressed as follows: where EK is the kinetic energy of the incident electrons in keV, and Z is the atomic number of the target electrode material. The most common target material is tungsten (W, Z = 74); in mammography, molybdenum (Mo, Z = 42) and rhodium (Rh, Z = 45) are also used. For 100-keV electrons impinging on tungsten, the approximate ratio of radiative to collisional losses is (100 x 74)/820,000 = 0.009 = 0.9%; therefore, more than 99% of the incident electron energy on the target electrode is converted to heat and nonuseful low-energy electromagnetic radiation. Characteristic x-ray Spectrum Characteristic x-rays are designated by the shell in which the electron vacancy is filled, and a subscript of a or b indicates whether the electron transition is from an adjacent shell (α) or nonadjacent shell (β). Characteristic radiation spectrum Atomic levels involved in copper Kα and Kβ emission Bremsstrahlung & Characteristic radiation spectrum Modern x-ray tubes Modern x-ray tubes The Envelope The envelope is the glass housing that protects the tube. It is also used to help protect from excessive exposure to x-rays. The envelope is the first part of the filtration system. Vacuum: The removal of the air permits electrons to flow from cathode to anode without encountering the gas atoms of air. Protective Housing - The housing controls leakage and scatter radiation, isolates the high voltages, and provides a means to cool the tube. - Leakage Radiation: Any photons that escape from the housing except at the port. Leakage radiation must not exceed 100 mR/hr at 1 meter. X-Ray Tube Heating There are three main types of heat generation in an x-ray tube. The first is by convection. – Convection: The transfer of thermal energy by actual physical movement from one location to another of a substance in which thermal energy is stored. Also known as thermal convection. The air is what is moving the heat energy around in the tube. If the tube has oil in it, then the oil is the convection material. The second is by conduction. – Conduction: The flow of thermal energy through a substance from a higher-to a lower-temperature region. This is from the stator axel to the anode. The third is by radiation. – Radiation: The energy radiated by solids, liquids, and gases in the form of electromagnetic waves as a result of their temperature. Also known as thermal radiation. This occurs when the electrons hit the anode target and heat up the anode. The x-ray radiation is given off which heats up the tube. Cathode filaments When energized, the filament circuit heats the filament through electrical resistance, and the process of thermionic emission releases electrons from the filament surface at a rate determined by the filament current and corresponding filament temperature. Electron beam focusing Tube Current vs Filament Current Anode Configurations Copper serves a dual role: it mechanically supports the insert and efficiently conducts heat from the tungsten target. However, the small area of the focal spot on the stationary anode limits the tube current and x-ray output that can be sustained without damage from excessive temperature. Dental x-ray units and some low-output mobile x-ray machines and mobile fluoroscopy systems use fixed anode x-ray tubes. Anode Geometry ❑ The picture shows what can happen to an anode when the anode stops turning. The anode actually melts. Anode Configurations: Stationary and Rotating ❑ The focal track area of the rotating anode is approximately equal to the product of the circumferential track length (2πr) and the track width (Δr), where r is the radial distance from the axis of the x-ray tube to the center of the track. ❑The allowable instantaneous heat loading depends on the anode rotation speed and the focal spot area. Faster rotation distributes the heat load over a greater portion of the focal track area for short exposure times. A larger focal spot allows a greater x-ray beam intensity but causes a loss of spatial resolution that increases with distance of the imaged object from the image receptor. Anode Geometry ❖ Anode angles in diagnostic x-ray tubes typically range from 7 to FIGURE 6-13 The anode (target) 20 degrees, with 12- to 15- angle, θ, is defined as the angle of degree angles being most the target surface in relation to the common. central ray. The focal spot length, as projected down the central axis, is foreshortened, according to the line focus principle ❑ The effective and actual focal spot lengths are geometrically related as: Effective focal length= Actual focal length x sin θ where θ is the anode angle. Foreshortening of the focal spot length at the central ray is called the line focus principle, as described by the above equation. An ability to have a smaller effective focal spot size for a large actual focal spot increases the power loadings for smaller effective focal spot sizes. The X-Ray Tube: -Target Area Target, focus, focal point, focal spot mean the same thing. This is where the high-voltage electrons hit the anode. Actual focal spot: The physical area of the focal track that is impacted. Focal Track: The portion of the anode where the high-voltage electron stream will impact. When discussing a rotating anode this describes the circular path that will be impacted by the electron beam. Effective focal spot: The area of the focal spot that is projected out of the tube toward the object being radiographed. In the diagram below there are two anode with different size focal spots. The one on the left has a larger actual focal spot than the one on the right. The X-Ray Tube: The angle of the focal spot is one way to control the size of the effective (apparent) focal spot. The larger the angle the larger the effective focal spot, as illustrated in the next two diagrams. Anode Angle, Field Coverage, and Focal Spot Size Measurement and verification of focal spot size FIGURE 6-16 Various tools allow measurement of the focal spot size, either directly or indirectly. A and E: Pinhole camera and images. B and F: Slit camera and images. C and G: Star pattern and images. D and H: Resolution bar pattern and images. For E–H, the top row of images represents the measurements of the large focal spot (1.2 mm x 1.2 mm), and the bottom row the small focal spot (0.6 mm x 0.6 mm). The star and bar patterns provide an “equivalent” focal spot dimension based upon the resolvability of the equivalent spatial frequencies. Anode Heel Effect Due to the geometry of the angled anode target, the radiation intensity is greater on the cathode side. As the figure below indicates the intensity of the x-ray beam is greater towards the cathode (filament) end of the tube. The reason that the Heel Effect occurs is illustrated below. The letters represent interactions with electrons from the cathode and the lines coming from the interactions are x-rays. More x-rays will be able to get out of the anode on the right side (the angle side) than on the left side because of all the material the x-rays must pass through to get out of the anode. Off-Focus Radiation Off-focal radiation results from electrons that scatter from the anode, and are re-accelerated back to the anode, outside of the focal spot area. Increasing patient exposure, Causing geometric blurring, Reducing image contrast, Increasing random noise. The image shows an extreme effect of off-focus radiation. You can see the image of the nose from the off-focus radiation. x-ray Tube Housing The x-ray tube housing supports, ❑ Federal regulations (21 CFR insulates, and protects the x-ray tube 1020.30) require manufacturers insert from the environment. to provide sufficient shielding to limit the leakage radiation exposure rate to 0.88 mGy air kerma per hour (equivalent to 100 mR/h) at 1 m from the focal spot when the x-ray tube is operated at the leakage technique factors for the x-ray tube. Leakage techniques are the maximal operable kV (kVmax, typically 125 to 150 kV) at the highest possible continuous current (typically 3 to 5 mA at kVmax for most diagnostic tubes). Collimators Collimators adjust the size and shape of the x-ray field emerging from the tube port. ❑ Federal regulations (21 CFR 1020.31) require that the light field and x-ray field be aligned so that the sum of the misalignments, along either the length or the width of the field, is within 2% of the SID. For example, at a typical SID of 100 cm (40 inches), the sum of the misalignments between the light field and the x-ray field at the left and right edges must not exceed 2 cm, and the sum of the misalignments at the other two edges also must not exceed 2 cm. Filtration Filtration is the removal of x-rays as the beam passes through a layer of material. Filtration includes both the inherent filtration of the x-ray tube and added filtration. Inherent filtration includes the thickness (1 to 2 mm) of the glass or metal insert at the x-ray tube port and effectively attenuate all x-rays in the spectrum below about 15 keV. Added filtration refers to sheets of metal intentionally placed in the beam to change its effective energy. Aluminum (Al) is the most commonly used added filter material. Compensation (equalization) filters are used to change the spatial pattern of the x-ray intensity incident on the patient, so as to deliver a more uniform x-ray exposure to the detector. Wedge filters are useful for lateral projections in cervical-thoracic spine imaging, where the incident fluence is increased to match the increased tissue thickness encountered (e.g., to provide a low incident flux to the thin neck area and a high incident flux to the thick shoulders). “Bow-tie” filters are used in CT to reduce dose to the periphery of the patient, where x-ray paths are shorter and fewer x-rays are required. Heel Effect Wedge filter compensation filter Bow-tie filters X-RAY GENERATOR FUNCTION AND COMPONENTS Transformers: Transformers: Autotransformer Modular system schematic Rectifier Circuit: Current and voltage supplies for x-ray tube Three-phase electric power Three-phase transformer with four wire output one wire for neutral, others for A, B and C phases. A neutral wire allows the three-phase system to use a higher voltage while still supporting lower-voltage single-phase appliances. FIGURE 6-25 In a high-frequency inverter generator, a single- or three phase AC input voltage is rectified and smoothed to create a DC waveform. An inverter circuit produces a high frequency AC waveform as input to the high-voltage transformer. Rectification and capacitance smoothing provide the resultant high- voltage output waveform, with properties similar to those of a three-phase system. High Frequency generator schematic Phototimers operate by measuring the actual amount of radiation incident on the image receptor and terminating x-ray production when the proper amount is obtained. In the event of a phototimer detector or circuit failure, a "backup timer" safety device terminates the x-ray exposure after a preset time. Radiation Glossary Half Value Layer (HVL): the thickness of material required to reduce the intensity of an x- or gamma-ray beam to one-half of its initial value. Tenth Value Layer (TVL): the thickness of material necessary to reduce the intensity of the beam to a tenth of its initial value. Fluence: the number of photons (or particles) passing through a unit cross-sectional area: photon = ( cm−2 ) Area Flux: the fluence rate (e.g., the rate at which photons or particles pass through a unit area per unit time) photon = ( cm−2 sec −1 ) Area.Time Energy Fluence: the amount of energy passing through a unit cross- sectional area photon Energy = = E ( keV / cm2 ) Area photon Radiation Glossary Mass Energy Absorption Coefficient: the mass attenuation coefficient multiplied by the fraction of the energy of the interacting photons that is absorbed to charged particles as kinetic energy: en ( cm 2 / kg ) o Absorbed Dose: the energy (ΔE) deposited by ionizing radiation per unit mass of material (Δm): E en Dose = = ( J / kg = gray( Gy )) m o E ❖ rad = 10 mGy = 0.01 J/kg Exposure: the amount of electrical charge (ΔQ) produced by ionizing electromagnetic radiation per mass (Δm) of air: Q X= ( C / kg or R ) m ❖ Radiation fields are often expressed as an exposure rate (R/hr or mR/min). Radiation Glossary W: the ratio of the dose (D) to the exposure (X) in air: – W; the average energy deposited per ion pair in air, is approximately constant as a function of energy. The value of W is 33.97 J/C. The roentgen-to-rad conversion factor versus photon energy for water, muscle, and bone. Radiation Glossary Equivalent Dose (H): The product of the absorbed dose (D) and the radiation weighing factor wR: H = D wR (rem or sievert (Sv)) 1 sievert = 100 rem Radiation Glossary Effective Dose (E(Sv)): The sum of the products of the equivalent dose to each organ or tissue irradiated (HT) and the corresponding weighting factor (wT) for that organ or tissue: E( Sv ) = wT H T ( Sv ) Descriptors of X-ray beams X-ray production efficiency, exposure, quality, and quantity are determined by six major factors: 1. x-ray tube target material, 2. voltage (kVp), 3. current (mA), 4. exposure time (s), 5. beam filtration, and 6. generator waveform. - Quality – penetrability of an x-ray beam, which is determined by the kVp, the target material and the filtration of the beam. (higher energy has higher HVL and higher penetration) - Quantity – number of photons which is proportional to tube current times the exposure time. (the higher the mAs, the higher the quantity) the x-ray quantity is approximately proportional to: Ztarget * kVp2 * mAs. - Exposure – proportional to the energy fluence (mR/min). Energy fluence: the amount of energy passing through a unit cross-sectional area: photon Energy = = E ( keV / cm2 ) Area photon Factors affecting X-ray emission 1) Target material - affects quality and quantity of radiation emitted 2) Tube voltage – kVp - determines maximum energy of photons emitted in the bremsstrahlung spectrum, thus affecting quality of the beam and the overall exposure. -Rule of thumb 1 : general Entrance Skin Exposure (ESE) α mAs∗ (kVp)2 -Rule of thumb 2: for the attenuated exposure (kVp1/kVp2)5 = mAs2/ mAs1 3) Tube current (mA) 4) Exposure time (s)– duration of x-ray production. Often the current and exposure time are expressed together as a product, in mAs. 5) Beam filtration – modifies the x-ray energy spectrum and the overall number of photons in the beam. 6) Generator waveform – affects the spectrum and quantity of photons emitted. Single phase system provides lower average energy and number of photons than does a three phase system. 7) Focal spot size – affects the number of photons being produced. Factors affecting X-ray emission FIGURE 6-30 x-Ray tube output intensity varies as the square of tube voltage (kV). In this example, the same tube current and exposure times (mAs) are compared for 60 to 120 kV. The relative area under each spectrum roughly follows a squared dependence (characteristic radiation is ignored). Factors affecting X-ray emission Heat Unit: a quantity related to the heat storage capacity of an X-ray tube. Constant Generator Type Single-phase 1.00 Three-Phase, Six 1.35 Pulse Three-Phase, 1.41 Twelve Pulse High-Frequency 1.45 Example 4 Calculate the heat units generated for the following exposures. Single-phase, rectified unit: 250 mA, 0.7 seconds, and 200 kVp. Example 5 Calculate the heat units generated for the following exposures. Three-phase, six pulse, rectified unit: 300 mA, 0.5 seconds, and 110 kVp. Example 6 Calculate the heat units generated for the following exposures. High Frequency unit: 500 mA, 0.9 seconds, and 300 kVp. Anode Cooling Charts: Permits the calculation of the time necessary for the anode to cool enough for additional exposure to be taken. When you need to find out how long it will take to cool the anode so that you can make more exposures you need to follow the instructions below. To use the Anode Cooling Chart: 1. Find the total heat units applied on the vertical scale. 2. Read from the heat units over to the cooling curve and then down to read the corresponding time. 3. Calculate the time necessary for the anode to cool to any desired level and subtract the corresponding time of the initial exposure. Example 8 For a High Frequency unit, calculate the length of time necessary for the anode to cool to 50000 HU after 5 exposures of 500 mA for 0.7 seconds at 120 kVp. - First find the heat units for one exposure. - Then find the heat units for 5 exposures. - Now use the chart and find the times. From the chart I you go to just over 300000 HU which is about 1 minute. Then you go to 50000 HU and this is about 6.25 minutes. The difference between these times is the amount of time to cool. It will take about 5.25 minutes for the anode to cool so that we can take more exposures. Summary: Housing Cooling Chart - Permits the calculation of the time necessary for the housing to cool enough for additional exposures to be made.