Part 4 [4.0].pdf
Document Details
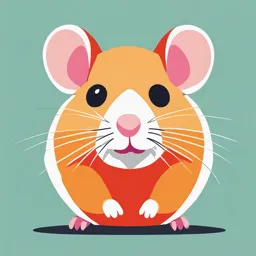
Uploaded by LikedRhodonite
Tags
Full Transcript
Y1B4M1L4 ARTICULATIONS, JOINT MOTION & MUSCLE CONTRACTION A. RECRUITMENT D. FORCE SUMMATION ● During a contraction, small motor units are recruited first, and then as forces are increased larger motor units are recruited. ● The size principle of motor unit recruitment – an energy-saving mechanism...
Y1B4M1L4 ARTICULATIONS, JOINT MOTION & MUSCLE CONTRACTION A. RECRUITMENT D. FORCE SUMMATION ● During a contraction, small motor units are recruited first, and then as forces are increased larger motor units are recruited. ● The size principle of motor unit recruitment – an energy-saving mechanism. ● Small motor units generate less tension thus require less energy expenditure (energy conserving). ● Large motor units are unnecessary if the task can be done by the small motor units unless the small motor units cannot accomplish the task. B. MUSCLE TONE ● The relaxed state of the muscle provides nearly consistent and low-level tension and resistance to stretch. ● Muscle tone (firmness of muscle) is maintained by spinal cord activity. ● No normal muscle is flaccid. Healthy muscles are never truly flaccid. ● If the nerve is damaged, the muscle loses muscle tone and becomes flaccid. C. MUSCLE TWITCH ● When a muscle is stimulated, it will twitch. LATENT PHASE ● Sarcolemma and T-tubules depolarize. ● Calcium is released in the cytosol. ● Cross-bridges begin to cycle but there is no visible shortening of muscles. Figure 56. Temporal Summation of Two Stimuli ● Summation – addition of individual twitch contractions to increase the intensity of overall muscle contraction. ● There are two ways in which summation can occur: ○ Multiple fiber summation – increasing the number of motor units contracting simultaneously. ○ Frequency summation – increasing the frequency of contraction, which can lead to tetanus. ● Mechanism of tetanization: When the frequency reaches a critical level, the successive contractions eventually become so rapid that they fuse together and the whole muscle contraction appears to be completely smooth and continuous. ● Tetany occurs because enough calcium ions are maintained in the muscle sarcoplasm, even between action potentials, so that a full contractile state is sustained without allowing any relaxation between the action potentials. CONTRACTION PHASE ● Sarcomeres shorten as a result of myosin cross-bridge cycling. ● Speed depends on weight being lifted and fiber type (slow-twitch fibers or fast-twitch fibers). RELAXATION PHASE ● The ends of the actin filaments extending from two successive Z discs barely overlap one another. ● Calcium is actively transported back into terminal cisternae. ● Cross-bridge cycling decreases and ends. ● Tension is reduced, and muscle returns to its original length. ● ATP is dephosphorylated to ADP. Figure 57. Frequency Summation and Tetanization Figure 58. Tetanus ● Tetanus – isometric tension of a single muscle fiber during continuously increasing and decreasing stimulation frequency. ○ Dots at the top are intervals of 0.2 s. Figure 55. Muscle Twitch Maximum Strength of Contraction: ● Maximum strength of tetanic contraction of a muscle operating at a normal muscle length is 3 and 4 kg/cm² of muscle or 50 psi (pounds per square inch). ● A quadriceps muscle can have up to 16 square inches of muscle belly, as much as 800 pounds of tension may be applied to the patellar tendon. ○ This shows how it is possible for muscles to pull their tendons out of their insertion in bone. BARBON, CALIZO, DAQUIL, DULANA, LAYGAN, RODRIGUEZ, SOMBONG, TILOS, TUMACOLE | MG 4 16 of 22 Y1B4M1L4 ARTICULATIONS, JOINT MOTION & MUSCLE CONTRACTION TREPPE (“STAIRCASE” EFFECT ● Treppe (staircase effect) – occurs as a consequence of changes in muscle strength at the onset of contraction. ● Mechanism of treppe: When a muscle begins to contract after a long period of rest, its initial strength of contraction may be as little as one-half its strength 10 to 50 muscle twitches later. ● The strength of contraction increases to a plateau. ● This is believed to be caused primarily by increasing calcium ions in the cytosol with each successive muscle action potential and failure of the sarcoplasm to recapture the ions immediately. TEMPORAL SUMMATION Figure 59. Temporal Summation ● Occurs after the first five stimuli. ● There is an increase in tension. INCOMPLETE TETANUS FATIGUE Figure 62. Fatigue ● Factors that contribute to muscle fatigue are: ○ Ionic imbalances ○ Inadequate ATP ○ Accumulation of by-products ● Effects of prolonged and strong muscle contraction are as follows: ○ Inability of the contractile and metabolic processes of the muscle fibers to continue supplying the same work output. ○ Transmission of the nerve signal through the neuromuscular junction can diminish at least a small amount after intense prolonged muscle activity, thus further diminishing muscle contraction. ○ Interruption of blood flow through a contracting muscle leads to almost complete muscle fatigue within 1 or 2 minutes because of the loss of nutrient supply, especially loss of oxygen. XI. LENGTH TENSION RELATIONSHIP ● The degree of stretch indicates how the forcefulness of muscle contraction depends on the length of the sarcomeres within a muscle before contraction begins. Figure 60. Incomplete Tetanus ● Continuous contraction with incomplete relaxation (shorter contraction relaxation cycle). Figure 63. Length-Tension diagram for a single fully contracted sarcomere Guyton & Hall, 13th Ed) ● This length-tension diagram shows the maximum strength of contraction when the sarcomere is 2.0 to 2.2 micrometers in length. To the upper right are the relative positions of the actin and myosin filaments at different sarcomere lengths from point A to point D. COMPLETE TETANUS Figure 61. Complete Tetanus ● Smooth continuous contractions with no complete relaxation Figure 64. Relation of muscle length to tension in the muscle both before and during muscle contraction Guyton & Hall, 13th Ed.) BARBON, CALIZO, DAQUIL, DULANA, LAYGAN, RODRIGUEZ, SOMBONG, TILOS, TUMACOLE | MG 4 17 of 22 Y1B4M1L4 ARTICULATIONS, JOINT MOTION & MUSCLE CONTRACTION A. UNSTRETCHED XII. WHOLE MUSCLE CONTRACTION A. ISOMETRIC ● Mechanism of isometric contraction: ○ Muscle does not shorten during contraction (no change in muscle length) ● There is no movement of joints. ● An isometric contraction occurs when the load is greater than the force of muscle contraction. ● Muscle creates tension when it contracts, but the overall length of the muscle does not change. Figure 65. Unstretched Muscle ● There is less tension in unstretched muscle that is stimulated. ● Relatively weak contraction results when an unstretched muscle is stimulated ○ Due to overlapping thin filaments conflicting with each other, restricting cross bridge binding. Thus, less tension develops. ● Tension decreases as sarcomere length decreases because thick filaments crumple. ○ This results in fewer myosin heads making contact with thin filaments. B. MODERATELY STRETCHED ● There is maximal tension in moderately stretched muscle that is stimulated. ● Due to optimum overlapping of thick and thin filaments, all cross bridges can participate in the contraction. ● Maximum tension 100% occurs when the zone of overlap between a thick and thin filament extends from the edge of the H zone to one end of a thick filament. ● All cross-bridges participate. C. OVERSTRETCHED Figure 67. Isometric Systems for Recording Muscle Contraction B. ISOTONIC ● Mechanism of isotonic contraction: Muscle shortens but the tension on the muscle remains constant throughout the contraction. ● Isotonic contraction occurs when the force of the muscle contraction is greater than the load and the tension of the muscle remains constant during the contraction. ● When the muscle contracts, it shortens and moves the load. ● Two types of isotonic contraction are: ○ Concentric – the muscle shortens [e.g. carrying a load (flexion)]. ○ Eccentric – the muscle lengthens (e.g. attempting to carry a heavy load which is beyond strength). Figure 66. Overstretched Muscle ● When an overstretched muscle is stimulated, the resulting contraction is very weak. ● Thin filaments are pulled almost to the ends of the thick filaments. Thus, only minimal tension develops. ● Rarely occurs in skeletal muscle because of bony attachments ● There is minimal tension in overstretched muscle that is stimulated. ● Zone of overlap shortens as muscle fibers lengthen. ● Fewer myosin heads can make contact with thin filaments, and the tension the fiber can produce decreases. ● When a skeletal muscle fiber is stretched to 170% of its optimal length, there is no overlap between the thick and thin filaments. ● Because none of the myosin heads can bind to thin filaments, the muscle fiber cannot contract, and tension is zero. Figure 68. Isotonic Systems for Recording Muscle Contraction XIII. MUSCLE REMODELING ● All muscles are constantly remodeled to match the functions required of them. A. HYPERTROPHY ● Increase in total muscle mass ● Results mostly from an increase in the number of myofilaments (fiber hypertrophy). ● Response to maximal contractions ● Mechanisms: ○ Increased rate of synthesis of contractile proteins ○ Increase in enzymatic systems ○ Addition of new sarcomeres BARBON, CALIZO, DAQUIL, DULANA, LAYGAN, RODRIGUEZ, SOMBONG, TILOS, TUMACOLE | MG 4 18 of 22 Y1B4M1L4 ARTICULATIONS, JOINT MOTION & MUSCLE CONTRACTION B. ATROPHY D. BIPENNATE ● Reduction in the number of individual muscle fibers. ● Due to a decrease in quantity of actin and myosin filaments. ● Also results in loss of muscle strength. ● Can occur from misuse (i.e., not using the muscle) & nerve damage. ● The tendon lies in the center of the muscle, and the muscle fibers pass to it from two sides (e.g., rectus femoris, gastrocnemius). XIV. FUNCTIONAL TERMINOLOGY OF MUSCLE ACTIVITY A. AGONIST ● a contracting muscle/muscle group that is the principal muscle producing a joint motion or maintaining a posture (primary flexor). B. ANTAGONIST ● a muscle or muscle group that possesses the opposite anatomic action of the agonist. ● Usually, a non-contracting muscle that passively elongates or shortens to permit the action to occur. ● Example: In flexion of the elbow joint, the triceps are antagonists. E. MULTIPENNATE ● The oblique fascicles converge on several tendons (e.g. soleus and subscapularis muscle, deltoid). Additional notes: ● The oblique angle of the muscle fibers in a pennate muscle disrupts the direct relationship between the length of the muscle fiber and the distance at which the total muscle can move a bony part. ● It also decreases the amount of force that is directed along the long axis of the muscle. ● Only a portion of the pennate muscle goes toward producing motion of the bony lever. ● The more oblique a fiber lies to the long axis of the muscle, the lesser force a muscle can exert to a tendon. C. SYNERGIST ● a muscle/muscle group that contracts at the same time as the agonist. ● Action identical to that of the agonist. ● Example: Brachialis and brachioradialis are synergists in the flexion of the elbow joint. ● Scenario: When the elbow is flexed, the primary flexor/agonist is the biceps brachii, the antagonist is the triceps and the synergists are the brachioradialis & brachialis muscles XV. MUSCLE CLASSIFICATION AS TO FIBER ARRANGEMENT A. STRAP/FUSIFORM ● Has a parallel fiber arrangement. ● Capable of greater shortening and ROM. ● Muscles with a parallel fiber arrangement will produce a greater range of motion with a bony lever than a pennate fiber arrangement. ● Fusiform muscles have longer fiber length than pennated muscles. ● Example of strap arrangement: Sternocleidomastoid muscle. Fascicles are long and extended throughout the muscle. Most, but not all fusiform fibers extend throughout the muscle. B. PENNATE ● Has an oblique arrangement. Fiber arrangement resembles that found in a feather. ● Fibers that make up the fascicles in a pennate muscle are usually shorter and more numerous. Figure 69. Muscle Classification As To Fiber Arrangement XVI. SPURT & SHUNT MUSCLES Table 5. Spurt & Shunt Muscle SPURT MUSCLES SHUNT MUSCLES Proximal attachment Far from joint axis Close to joint axis Distal attachment Close to joint axis Far from joint axis Function Mobility (rotatory) Stability (translatory) C. UNIPENNATE ● The tendon lies along one side of the muscle, and the muscle fibers pass obliquely to it (e.g., extensor digitorum longus, palmar interosseous). ○ (e.g. flexor ____ longus) obliquely set fascicles fan/pan out on only one side of the central tendon. BARBON, CALIZO, DAQUIL, DULANA, LAYGAN, RODRIGUEZ, SOMBONG, TILOS, TUMACOLE | MG 4 19 of 22 Y1B4M1L4 ARTICULATIONS, JOINT MOTION & MUSCLE CONTRACTION XVII. TONIC & PHASIC MUSCLES Table 6. Tonic & Phasic Muscle TONIC MUSCLES PHASIC MUSCLES Fiber type High proportion of SO fibers High proportion of FG fibers Fiber arrangement Pennate parallel Location Deep; cross 1 joint Superficial; cross 1 joint Function stability mobility Action ext/abd/ER fix/add/IR XVIII. MUSCLE ARCHITECTURE Additional notes: ● These structural variations affect not only the overall shape and size of the muscles but also the function of the skeletal muscle. ● Five architectural characteristics that affect muscle function: size, arrangement, fiber length, muscle length, & physiologic cross-sectional area ● The two most important architectural characteristics that affect muscle function are the fiber length and PCSA. A. FIBER LENGTH ● Number of sarcomeres along the fiber directly determines the amount of shortening or lengthening of the fiber. ● A long muscle fiber with more sarcomeres in series is capable of shortening over a greater distance than a short muscle fiber. ● For example, if muscle fibers are capable of shortening about 50% of their resting length. A long muscle fiber of 6 cm in length is capable of shortening to 3 cm; while a short muscle fiber of 4 cm is only capable of shortening to 2 cm. ● Thus, a hypothetical muscle with long muscle fibers is able to move a bony lever ( to which it is attached) to a greater distance, than a muscle with short muscle fibers. A. FIBER ARCHITECTURE ● Physiologic cross-section transects each fasciculus at a right angle. ● This is contributory to absolute muscle strength (3 to 4 kg/cm2). ● Pennate muscle fibers have greater strength and are more capable of producing tension than parallel muscle fibers. B. AGE AND GENDER ● Strength increases from birth to adolescence, peaks at age 20 to 30 years, and gradually declines thereafter. ● Males exhibit greater strength after puberty. ● This is related to greater muscle mass. C. LENGTH TENSION RELATIONSHIP ● Optimal length is 1.2 times the resting muscle length. ● Lengthened state is related to moderate tension. ○ Moderately stretched state will generate maximum tension. ○ Overly stretched state will result in weak tension. ● Shortened state is related to minimal tension. D. SPEED OF CONTRACTION ● The maximum number of cross-bridges can be formed at slower speeds of contraction. ● For isometric and concentric contractions, there is a decrease in contractile force with increasing speed of shortening. XX. LEVER SYSTEM A. 3 FORCES OF THE MECHANICAL LEVER ● Axis A ● Weight/Resistance W ● Moving force F B. PHYSIOLOGIC CROSS SECTIONAL AREA PCSA ● Measure of the cross-sectional area of a muscle perpendicular to the orientation of the muscle fibers. ○ Muscle force is directly proportional to the number of sarcomeres aligned side by side/parallel. ○ Therefore, if there is a large number of fibers packed into a muscle (e.g. pennate muscle), or if the fibers increase in size due to addition of myofibrils, the ability to produce force increases. XIX. MUSCLE STRENGTH ● ● ● ● The ability of a muscle to generate active tension The capacity of a muscle to produce force neurologic/metabolic/endocrine/psychological factors Other factors that affect muscle strength: fiber architecture, age & gender, muscle size, length-tension relationship, speed of contraction, and leverage of the muscle. Figure 70. Lever B. IN THE BODY ● Counterparts of the three forces of the mechanical lever in the human body are: ○ Bone - rigid arm/ lever ○ Joint - axis ○ Muscle contraction – moving force ● Weight of the body/applied resistance – weight or resistance ● Weight arm WA ○ the perpendicular distance from the axis of the line of action of the weight. ○ Area between fulcrum and weight. ● Force arm FA ○ the perpendicular distance from the moving force to the axis. ○ Area between fulcrum and force. BARBON, CALIZO, DAQUIL, DULANA, LAYGAN, RODRIGUEZ, SOMBONG, TILOS, TUMACOLE | MG 4 20 of 22 Y1B4M1L4 ARTICULATIONS, JOINT MOTION & MUSCLE CONTRACTION ● Formula for mechanical advantage: 𝐹𝐴 𝑀𝐴 = 𝑊𝐴 Figure 71. Lever System C. CLASSES OF LEVER SYSTEMS Figure 74. Second Class Lever 1ST CLASS LEVEL ● In a first-class lever, two resultant forces are applied on either side of an axis, creating rotation in opposite directions. ● Thus, FA = WA ; MA 1. ● A real-life example is the see-saw or balance scale. ● In the body, an example is the atlanto-occipital joint. Figure 75. Second Class Lever in the Human Body Figure 72. First Class Lever Figure 73. First Class Lever in Human Body 3RD CLASS LEVEL ● In a third-class lever, the moving force lies closer to the axis than the weight or resistance. ● Thus, FA < WA ; MA 1 (about 0.5 . ○ Smaller mechanical advantage will give speed/mobility. ● Examples: ○ deltoid acting on the glenohumeral joint. ○ Biceps flexing the elbow against resistance. ● AF - contracting biceps pulling radius by its tendonal attachment ● Axis A - in the middle ● Load/Resistance R - weight of the anterior part of the face ● Applied Force AF - muscles of the neck apply force to lift the head up. ● Conversely, the neck flexor muscles on the anterior aspect may also be the AF to cause neck flexion or bending of the neck. 2ND CLASS LEVER ● In a second-class lever, two resultant forces are applied so that the resistance lies between the moving force and the axis. ● Thus, FA > WA ; MA 1 (about 2 . ○ Bigger mechanical advantage will provide a force advantage so that large things can be moved by a small force. ● An everyday example is the wheelbarrow/crowbar. ● Calf muscles lifting the body around the axis of the toes (tiptoeing) are one of the human bodyʼs second-class levers. ● AF - contraction of the gastroc soleus muscle to plantar flex the ankle. Figure 76. Third Class Lever Figure 77. Third Class Lever in the Human Body BARBON, CALIZO, DAQUIL, DULANA, LAYGAN, RODRIGUEZ, SOMBONG, TILOS, TUMACOLE | MG 4 21 of 22