Drought-Induced Mosquito Outbreaks in Wetlands (2003) - PDF
Document Details
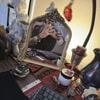
Uploaded by rafawar1000
Florida Atlantic University
2003
Jonathan M. Chase and Tiffany M. Knight
Tags
Summary
This scientific paper investigates the link between drought and mosquito population outbreaks in wetlands. It explores how biotic interactions (like competition and predation) and abiotic factors (like precipitation) influence mosquito abundance. The research suggests that drought events can cause considerable mosquito outbreaks in wetlands, particularly semi-permanent ones.
Full Transcript
Ecology Letters, (2003) 6: 1017–1024 doi: 10.1046/j.1461-0248.2003.00533.x REPORT Drought-induced mosquito outbreaks in wetlands Abstract Jonathan M. Chase1* and M...
Ecology Letters, (2003) 6: 1017–1024 doi: 10.1046/j.1461-0248.2003.00533.x REPORT Drought-induced mosquito outbreaks in wetlands Abstract Jonathan M. Chase1* and Mosquitoes are not only a nuisance, but also vector many important human and animal Tiffany M. Knight2 diseases. Here, in opposition with the dogma that increased precipitation predicts 1 Department of Biology, mosquito abundance, we hypothesize that mosquitoes should show population Washington University, Box No. outbreaks after drought years. Specifically, we suggest that in wetlands that never dry 1137, St Louis, MO 63130, USA (permanent), predators limit mosquito abundance, whereas in wetlands that dry yearly 2 Department of Zoology, (temporary), competitors that are well adapted to predictable drying, limit mosquito University of Florida, abundance. However, in wetlands that dry only during drought years (semi-permanent), Gainesville, FL 32611, USA *Correspondence: E-mail: mosquito predators and competitors are eliminated and must recolonize following a [email protected] drought, and the abundance of wetland mosquitoes can skyrocket. We present supportive evidence for this hypothesis from surveys of natural wetlands and from a controlled mesocosm experiment. We conclude that this framework may provide a reliable way to predict and prepare for year-to-year variation in mosquito abundances at large spatial scales. Keywords Competition, drought, food web, mosquito, outbreak, precipitation, predation. Ecology Letters (2003) 6: 1017–1024 limited to very short time scales (e.g. Aniedu 1992; Vandyk INTRODUCTION & Rowley 1995; Dhileepan 1996; Lindblade et al. 1999; Mosquitoes. ÔNo animal on earth has touched so directly and Webb & Russell 1999; Gleiser et al. 2000; Wegbreit & profoundly the lives of so many human beingsÕ (Spielman & Reisen 2000). Currently, no reliable information exists to D’Antonio 2001). In addition to being a general nuisance to predict year-to-year variation in mosquito abundance, humans, mosquitoes are vectors for several important human although it would allow for more informed management diseases, such as malaria, dengue fever, yellow fever and West strategies, including judicious use of pesticides, which can Nile virus, as well as diseases of domesticated animals, such often have adverse effects on ecosystems, and preparations as equine encephalitis and canine heartworm (e.g. Mulla for the protection against and treatment of mosquito- 1994; Maguire et al. 1999; Rose 2001; Hay et al. 2002). vectored diseases. Although a great deal of research is focused on understand- In this article, we use an emerging conceptual framework ing the molecular biology of mosquitoes and their role as from the community ecology of aquatic food webs to disease vectors, basic mosquito ecology is often poorly produce and test a novel hypothesis regarding year-to-year integrated into these studies (Curtis 2002; Enserink 2002). variation in mosquito abundance. Specifically, we argue that The vast majority of species show highly variable because larval mosquitoes are components of a much larger population densities through time (Fagan et al. 2001), and metacommunity of interacting species, the interaction mosquitoes are no exception (Spielman & D’Antonio 2001). between biotic interactions (competitors and predators) It is widely thought that abiotic factors, particularly and abiotic constraints (habitat drying) is essential for precipitation, limit mosquito abundance and thus mosquito understanding the controls on mosquito abundance. We populations should positively covary with precipitation. The further suggest that by placing mosquitoes into a broader reasoning for this supposition is that precipitation creates community context, a much more predictive framework can habitats for mosquitoes to lay their eggs and for their aquatic be developed for understanding and predicting year-to-year larvae to grow. Surprisingly, however, precipitation almost variation in mosquito abundances. never correlates with mosquito abundance in the long-term; Many species of mosquitoes are habitat generalists which instead, our ability to predict mosquito abundance has been breed, grow as larvae and emerge from a wide variety of 2003 Blackwell Publishing Ltd/CNRS 14610248, 2003, 11, Downloaded from https://onlinelibrary.wiley.com/doi/10.1046/j.1461-0248.2003.00533.x by Florida Atlantic University Libraries, Wiley Online Library on [30/08/2023]. See the Terms and Conditions (https://onlinelibrary.wiley.com/terms-and-conditions) on Wiley Online Library for rules of use; OA articles are governed by the applicable Creative Commons License 1018 J. M. Chase and T. M. Knight aquatic habitats (Carpenter & LaCasse 1955). These species, In years prior to a drying event, we predict that predators including many members of the genera Anopheles, Culex and will be common, as in permanent wetlands, because the Culiseta, are also some of the most important human pests habitat has retained standing water for several seasons, and disease vectors. In eastern North America, some of the allowing sufficient time for predator colonization. As a most common mosquito species are members of the result, in most years, mosquito densities will be low in semi- Anopheles quadrimaculatus species complex and Culex pipiens. permanent wetlands. In years following a drying event, Although these species have somewhat distinct habitat however, we predict that both efficient mosquito predators preferences, they readily lay eggs in, and emerge from and mosquito competitors will be rare, as neither group of wetlands of all types (Carpenter & LaCasse 1955). Further, species are well adapted to drying events. Furthermore, although C. pipiens and to a lesser degree A. quadrimaculatus, because mosquitoes have extremely rapid generation times will also breed in smaller water-filled habitats (e.g. contain- (weeks) relative to their predators and can readily disperse ers, ditches), which are often associated with humans, among habitats, we predict that mosquitoes should show wetlands provide a much greater area for potential larval rapid population increases in semi-permanent wetlands in habitats, and often produce many more adult mosquitoes, years following a drought event. than the smaller habitats traditionally associated with In this study, we evaluate this hypothesis by using two mosquito control. As such, we suggest that understanding complimentary approaches. First, we censused larval mos- the dynamics of mosquitoes in these wetland habitats will quitoes, and their potential predators and competitors in provide a much better understanding of yearly variation in natural wetlands that varied in their probability of retaining the abundances of mosquitoes in the landscape. permanent standing water during normal and drought years. In wetlands, the abundances of mosquito larvae are often Second, we established a long-term experiment in artificial limited by biotic factors, such as predators and competitors wetland mesocosms where we manipulated the permanence (Blaustein & Karban 1990; Blaustein & Margalit 1996; of wetlands experimentally so that they dried every year, one Blaustein 1998; Stav et al. 2000; Mokany & Shine 2003). In of every 3 years, or never, and then measured the response addition, the importance of these biotic interactions varies of mosquitoes, their competitors and their predators. depending on the type of wetland. Wetlands can be divided into three classes – temporary, permanent and semi- permanent – based on their probability of retaining standing METHODS water throughout the year; this in turn determines the types Natural history and species involved of species that can live in those habitats and their interspecific interactions (Schneider & Frost 1996; Wellborn Larvae of the two most common mosquito species encoun- et al. 1996; Williams 1996). Permanent wetlands always tered in the natural and artificial wetlands, A. quadrimaculatus retain standing water. In these habitats, predators, including and C. pipiens (>95% of the larval mosquito community), and fish and many insects, can complete their life cycles and other types of mosquito larvae, utilize different feeding reach very high densities. Thus, we predict that mosquito behaviours and have slightly different diets (e.g. Merritt et al. densities will be low in permanent wetlands as a result of 1992). They are both generalists, however, and readily predation. Temporary wetlands are those that fill and dry consume detritus, microbes and algae, both from the every year. Although some predators subsist in these benthos and the water column. As such, they are likely to temporary habitats (Spencer et al. 1999), most of the more compete for resources with several other co-occurring efficient mosquito predators (e.g. fish, large insects) cannot species. Potential mosquito competitors in these wetlands live in these habitats because of their drying. Alternatively, included several insects (Chironomidae, some Corixidae), mosquito competitors, which can adapt to the predictable molluscs (Pulmonate snails), anuran larave (Ranidae, yearly drying of these wetlands (e.g. zooplankton with Hylidae), amphipods, and cladoceran and copepod resting eggs), are often quite dense in the absence of zooplankton. Common mosquito predators included small predators. Thus, we predict that the number of mosquitoes fishes (mainly Cyprinidae and Gasterosteiridae), larval emerging from temporary wetlands will be low in temporary salamanders (Ambystomatidae, Salamandridae), and several habitats as a result of strong competitive interactions. This types of insects, including Hemiptera (e.g. Notonectidae, effect could either be because of lower rates of emergence if Naucoridae, other Corixidae), Coleoptera (Dyticidae) and high competitor density slows the rate of larval develop- Odonata (Libillulidae, Aeshnidae, Coenegrionidae, Lestidae). ment, or because of higher larval mortality and/or There are certain complexities associated with grouping avoidance of oviposition by females in wetlands with high species only as competitors and predators, which we do not competitor density. Semi-permanent wetlands are those that explicitly consider here because of space limitations and the retain standing water in most years, but periodically dry coarse level of predictions that we are trying to test here. For when precipitation and the water table are particularly low. example, C. pipiens and A. quadrimaculatus vary in their 2003 Blackwell Publishing Ltd/CNRS 14610248, 2003, 11, Downloaded from https://onlinelibrary.wiley.com/doi/10.1046/j.1461-0248.2003.00533.x by Florida Atlantic University Libraries, Wiley Online Library on [30/08/2023]. See the Terms and Conditions (https://onlinelibrary.wiley.com/terms-and-conditions) on Wiley Online Library for rules of use; OA articles are governed by the applicable Creative Commons License Drought-induced mosquito outbreaks in wetlands 1019 relative preferences for grazing on surfaces vs. in the water Pymatuning Laboratory of Ecology, University of Pitts- column, and thus are more likely to compete differentially burgh (Crawford County, PA, USA). Each tank was filled with grazers (e.g. snails and tadpoles) or filter feeders (e.g. with 4–6 cm of topsoil and nutrient poor well water so that zooplankton). As a result, surface grazers may actually they were two-thirds full. Further, each tank was inoculated facilitate filter-feeding mosquitoes and filter feeders may with a wide variety of species (e.g. macrophytes, plankton, facilitate grazing mosquitoes indirectly. However, when macroinvertebrates and larval amphibians) that occurred in both groups of competitors are present, their overall effect the regional species pool, and left open so that natural on mosquitoes should be negative, and so we only consider colonization of mosquitoes and other species could occur. their negative effects here. In addition, some species which Permanence (three levels) and nutrients (three levels) were we call competitors may also eat mosquitoes to a limited manipulated in a factorial design with four replicates for each degree. Nevertheless, our groupings provide a first approxi- treatment. There were no main or interactive effects of the mation of how biotic interactions limit mosquito densities. nutrient treatments (ANOVA; P > 0.2) on any of the response variables of interest here, and thus we do not discuss them further here. To manipulate permanence we connected a Wetland surveys 5 cm diameter PVC pipe to the bottom drain of each Wetlands were identified in north-west Pennsylvania mesocosm, so that we could slowly dry them over the course (Crawford County, USA), and were followed from 1998 of the summer (from June to September); wetlands naturally to 2001. The year 1999 was a particularly dry year, in which refilled over the winter with precipitation and were topped- several wetlands only dried in that year, but not in 1998, off with well water in early spring when necessary. 2000 or 2001. Wetlands that dried only in 1999 were Each mesocosm was assigned to one of three perma- classified as semi-permanent, those that dried every year nence treatments: (1) permanent (never dry), (2) temporary were classified as temporary, and those that never dried were (dry every year), (3) semi-permanent (dry in the second year classified as permanent. We chose three wetlands from each of the 3 years). In April–June 2001, we censused mosqui- permanence category that were similar in total surface area toes, potential mosquito competitors and potential mos- and water chemistry for sampling and analyses. Mosquito quito predators, in each mesocosm biweekly. As we were larvae, predators and competitors were sampled during May primarily interested in how many mosquitoes emerged from of each year. Macroinvertebrates were sampled in 5–10, 1 m each different larval environment to become reproductive sweeps (depending on the size of the wetland) using a 0.1 m adults, we censused pupal exuviae that were left behind width D-net with 0.5 mm mesh. Zooplankton were sampled upon successful metamorphosis of a pupae into an adult using 5–10 vertical pulls of a 10 cm diameter 48 lm mesh mosquito. Exuviae were counted and removed from the net. All animals were counted and classified according to mesocosm on each sampling date. Macroscopic mosquito three categories – mosquitoes, potential competitors or predators and large competitors were censused in two ways: potential predators – and converted to biomass using (1) visually by counting organisms on the sides of the species and size-specific dry-weight conversions. mesocosm and the surface of the vegetation and ground, Our methods were chosen so as to standardize sampling and (2) with a 10 cm · 10 cm net with 0.33 mm mesh net as much as possible across these highly divergent wetland 10 cm; we swept the net through the vegetation 10 times types. However, because the wetland types differ markedly and counted all organisms captured. The numbers were in several factors, notably their geomorphic structure and converted to dry-weight estimates as above. Zooplankton vegetation, this could have created sampling biases when were censused by collecting 5 L of water in an integrated trying to compare among the wetland types. We have used a tube sampler, and were preserved and counted as above. variety of sampling methods in these types of wetlands, and find our estimates relatively robust, at least qualitatively, to each other (J. M. Chase, unpublished data). Nevertheless, we RESULTS cannot say for certain whether such a bias was introduced; if Wetland surveys it was, it could have either increased or decreased any observed differences among trophic groups within the Repeated measures ANOVA showed a significant effect of natural wetlands. wetland permanence type (F2,6 ¼ 20.7, P < 0.002), year (F2,4 ¼ 17.93, P < 0.001) and their interaction (F2,4 ¼ 17.0, P < 0.001) on larval mosquito biomass. We found a Experimental mesocosms significant effect of wetland permanence type In 1999, as part of a larger experiment, we established thirty (F2,6 ¼ 25.56, P < 0.001), a marginally significant effect of six 1140 litre mesocosms (1.5-m diameter Rubbermaid year (F2,4 ¼ 3.39, P ¼ 0.07) and a marginally significant cattle tanks) in full sunlight in a large old field near the wetland permanence type by year interaction (F2,4 ¼ 3.05, 2003 Blackwell Publishing Ltd/CNRS 14610248, 2003, 11, Downloaded from https://onlinelibrary.wiley.com/doi/10.1046/j.1461-0248.2003.00533.x by Florida Atlantic University Libraries, Wiley Online Library on [30/08/2023]. See the Terms and Conditions (https://onlinelibrary.wiley.com/terms-and-conditions) on Wiley Online Library for rules of use; OA articles are governed by the applicable Creative Commons License 1020 J. M. Chase and T. M. Knight P ¼ 0.06) on competitor biomass. Finally, we found a (a) 15 significant effect of wetland permanence type (F2,6 ¼ 22.43, P < 0.002), year (F2,4 ¼ 15.62, P < 0.001) and their Mosquito biomass (g m–2) interaction (F2,4 ¼ 21.63, P < 0.001) on predator biomass. 10 Following the significant main effects of wetland perma- nence type, we used Tukey’s HSD for pairwise comparisons. In 1999 and 2001, mosquito abundance was not different among any wetland type (Tukey’s HSD; P > 0.3). However, 5 in 2000, following the drought event, mosquito abundance was highest in semi-permanent wetlands (Tukey’s HSD; P < 0.001), showing a 15-fold increase, but equally low in 0 temporary and permanent wetlands (Tukey’s HSD; P > 0.4) (Fig. 1a). Competitor biomass was highest in temporary 20 wetlands in all 3 years compared with both the permanent (b) and semi-permanent wetlands (Tukey’s HSD; P < 0.05). Competitor biomass (g m–2) However, competitor biomass was not different between 15 permanent and semi-permanent habitats in 1999 and 2001 (Tukey’s HSD; P < 0.4), but was much lower in semi- 10 permanent habitats in 2000, following the drought year (Tukey’s HSD; P < 0.01) (Fig. 1b). Predator biomass was much higher in permanent and semi-permanent wetlands 5 than in temporary wetlands in 1999 and 2001 (Tukey’s HSD; P < 0.05), and there was no difference between the permanent and semi-permanent wetlands (Tukey’s HSD; 0 P > 0.4). However, in 2000 (following the drought), predator biomass was highest only in the permanent (c) 10 wetlands (Tukey’s HSD; P < 0.01), and equally low in both Predator biomass (g m–2) the semi-permanent and temporary habitats (Tukey’s HSD; P > 0.5) (Fig. 1c). 5 Experimental mesocosms Only two species of mosquito, A. quadrimaculatus and C. pipiens, were encountered in the majority of these mesocosms throughout the growing season. Some other species, notably Aedes spp. and Culiseta spp., were 0 1999 2000 2001 observed in 1% of the larvae encountered (b) and their predators (c) over the 3-year period (1999–2001) in these mesocosms, and thus we do not include them in within natural wetlands that varied in permanence. Squares the following analyses. represent temporary wetlands (dried every year), circles represent As a result of the large responses of all three groups of permanent wetlands (never dried) and triangles represent semi- species (mosquitoes, their competitors and their predators) permanent wetlands (dried during the 1999 drought year). to the treatments, variances were not equal among treatments for each response variable (Bartletts–Box F-test; P > 0.05). Thus, rather than parametric ANOVA, we used species (Kruskall–Wallis; A. quadrimaculatus d.f. ¼ 2, nonparametric Kruskall–Wallis tests (ANOVA gives qualita- H ¼ 24.54; C. pipiens d.f. ¼ 2, H ¼ 15.95, P < 0.0001), tively identical results in all cases). In the third year of the competitor biomass (Kruskall–Wallis; d.f. ¼ 2, H ¼ 29.38, experiment (2001), following the experimentally induced P < 0.0001), and predator biomass (Kruskall–Wallis; drought, the wetland permanence treatment (temporary, d.f. ¼ 2, H ¼ 27.37, P < 0.0001). permanent and semi-permanent) significantly affected the The number of adult mosquitoes that emerged from number of emerging adults of both common mosquitoes these wetlands was over 20-fold higher in the semi- 2003 Blackwell Publishing Ltd/CNRS 14610248, 2003, 11, Downloaded from https://onlinelibrary.wiley.com/doi/10.1046/j.1461-0248.2003.00533.x by Florida Atlantic University Libraries, Wiley Online Library on [30/08/2023]. See the Terms and Conditions (https://onlinelibrary.wiley.com/terms-and-conditions) on Wiley Online Library for rules of use; OA articles are governed by the applicable Creative Commons License Drought-induced mosquito outbreaks in wetlands 1021 (a) 200 P < 0.05) (Fig. 2b). Predator biomass was highest in the permanent treatments (Tukey’s HSD; P < 0.01), but equally Number of adult mosquitoes low in both the temporary and semi-permanent treatments 150 (Tukey’s HSD; P > 0.2) (Fig. 2c). Thus, this experiment provides evidence that these wetland mosquitoes show 100 population outbreaks following a drought event in semi- permanent habitats, and that this is associated with lower 50 predator and competitor biomass. 0 DISCUSSION (b) 10 We found that mosquito density increased dramatically following a natural drought event in a survey of wetlands, Competitor biomass (g m–2) and after an experimental drought event in outdoor mesocosms intended to mimic many features of natural wetlands. These patterns are consistent with our hypothesis 5 that predators and competitors limit mosquito populations in permanent and temporary wetlands, respectively, whereas in semi-permanent wetlands, mosquito densities can out- break following drought years because of the loss of both 0 these groups of interactors. The reason this occurs is most likely because these mosquitoes are habitat generalists and (c) 4 opportunists, and following a drought event, they can Predator biomass (g m–2) quickly re-colonize these wetlands. Although predators can 3 also recolonize these semi-permanent habitats following drought, particularly when many individuals are in their 2 terrestrial adult stage (e.g. dragonflies), mosquitoes typically have much more rapid population dynamics (on the order of weeks) than predators (on the order of months to years), 1 allowing them to escape control by predators and achieve population outbreaks in a very short period of time. 0 As an alternative to the predator and competitor ary t m por nen per rma mi- limitation hypothesis that we propose, it is possible that Tem Pe Se Wetland permanence type the patterns we observed may have resulted from different mechanisms. For example, if the act of wetland drying itself Figure 2 Number (±1SE) of emerged adult mosquitoes (a) and induced some sort of difference in larval food supply or biomass (± 1SE) of their competitors (b) and predators (c) within related factor, then we would expect the same pattern of mesocosms where permanence was experimentally manipulated. mosquito density increases following wetland drying. How- Data are only presented for 2001, which was the year immediately ever, we feel that this scenario is unlikely as mosquitoes following the experimental drought event in the semi-permanent were rare in both natural and experimental temporary mesocosms. The number of emerging adult mosquitoes is shown for wetlands, which dried in the same manner as the semi- the two most common species, Anopheles quadrimaculatus (dark bars) and Culex pipiens (white bars); other mosquito species represented permanent wetlands, but did not show similar increases in >1% of the mosquitoes encountered in these wetlands. mosquito abundance. From our results, we cannot discern whether the differences in mosquito abundance are the result of permanent treatments than in either the temporary or differential oviposition by adult mosquitoes or differential permanent treatments (Tukey’s HSD; P < 0.01), whereas mortality of larval mosquitoes. Several species of mosquito the number of mosquitoes that emerged from the have been observed to preferentially oviposit eggs in permanent and temporary treatments were uniformly low, habitats without predators (Chesson 1984; Petranka & and not different from one another (Tukey’s HSD; P > 0.3) Fakhoury 1991; Angelon & Petranka 2002; Spencer et al. (Fig. 2a). Competitor biomass was highest in the temporary 2002; Kilfawi et al. 2003a); furthermore, some evidence also treatments, intermediate in the semi-permanent treatments suggests that mosquitoes can similarly detect and respond to and lowest in the permanent treatments (all Tukey’s HSD; habitats with different densities of competitors (Blaustein & 2003 Blackwell Publishing Ltd/CNRS 14610248, 2003, 11, Downloaded from https://onlinelibrary.wiley.com/doi/10.1046/j.1461-0248.2003.00533.x by Florida Atlantic University Libraries, Wiley Online Library on [30/08/2023]. See the Terms and Conditions (https://onlinelibrary.wiley.com/terms-and-conditions) on Wiley Online Library for rules of use; OA articles are governed by the applicable Creative Commons License 1022 J. M. Chase and T. M. Knight Kotler 1993; Edgerly et al. 1998; Kiflawi et al. 2003b; Mokany utilize wetlands for larval habitats, (3) predators and & Shine 2003). A recent study has suggested that whether competitors are negatively affected by drought and have mosquitoes can behaviourally avoid oviposition in habitats slower generation times than mosquitoes, and (4) semi- with predators, or whether predators simply reduce larval permanent wetland habitats represent a significant propor- mosquito abundance through direct mortality, can have tion of potential larval mosquito habitats. quantitative effects on the overall response of mosquito In conclusion, we predict that mosquito densities are populations to the presence of predators (Spencer et al. controlled by an interaction between abiotic (habitat 2002). However, whether the effect of predators and availability and precipitation) and biotic (competition and competitors observed in our study was primarily because predation) factors, and that outbreaks are likely to occur on of direct mortality or indirect behavioural decisions (ovipo- a landscape level following drought years. Our hypotheses sition habitat selection) is not likely to alter the qualitative and observations are based on species that are able utilize predictions of spatial and temporal variation in mosquito wetlands as larval habitats, but similar patterns have been abundances in food webs that we have discussed here. In suggested for species that specialize on a limited set of addition, although oviposition behaviour may have played a container habitats (Lounibos 1983, 1985; Bradshaw & role in the patterns we observed, in a separate study, we Holzapfel 1988). Further, our observations may lend insight found that differential mortality because of the presence of into understanding patterns of mosquito-borne diseases, competitors and predators, while all else was equal, had a and the potential for global climate change to influence strong negative effect on the number of emerging adult mosquito population dynamics and disease incidence. mosquitoes similar to that observed here (T. Knight, Although some statistical correlations between disease J. Chase, C. Goss & J. Knight, unpublished data). incidence and precipitation have been found, there is little Our main prediction and results are in opposition to the predictability or mechanistic understanding of these patterns widely recognized hypothesis that mosquito densities as of yet (Hay et al. 2000). If our model for mosquito should be highest when precipitation is highest. Although outbreaks translates to mosquito abundances at the land- precipitation sometimes correlates with mosquito densities scape level, then we would predict that the likelihood of in the short-term, it does not predict year-to-year variation disease outbreaks might increase in years following drought in mosquito abundance (e.g. Aniedu 1992; Vandyk & years; a pattern that seems to occur at least in Saint Louis Rowley 1995; Dhileepan 1996; Lindblade et al. 1999; Webb encephalitis outbreaks in North America (e.g. Shaman et al. & Russell 1999; Gleiser et al. 2000; Wegbreit & Reisen 2002; although these authors present a more complex 2000). Other hypotheses to explain yearly variation in hypothesis for the underlying mechanism of this pattern), mosquito abundance similarly have little quantitative and perhaps several other diseases. However, a variety of support. Some mosquito ecologists suggest that all other factors associated with the epidemiology of disease mosquito species respond to the environment differently, transmission will also vary from year to year, such as and that no single factor could reliably predict the variation changes in the stress experienced by the host populations in mosquito densities (Spielman & D’Antonio 2001). We (Lafferty & Holt 2003), or changes in host behaviour and agree that mosquito species have very different natural immunity (Shaman et al. 2002). Finally, although global histories, and likely respond to environmental variation in climate change is controversial, there is evidence that different ways. However, we suggest that some of the climatic variability and periodic drought conditions, such as most extensive potential larval habitats are wetlands, and that associated with the El Nino-Southern Oscillation, has that many species of mosquitoes are habitat generalists increased as a result of anthropogenic influences (Tudhope able to take advantage of year-to-year variation in the et al. 2001). We suggest that such variability will increase the suitability of these habitats, and thus are likely to fit into frequency and possibly the magnitude, by which mosquito our predictions. population outbreaks, and possibly their associated health Despite our supportive data at relatively small spatial problems, occur. and short temporal scales, other information will be necessary in order to determine how well our hypothesis, ACKNOWLEDGEMENTS which is based on local biotic interactions among mosquito larvae imbedded within food webs, scales up to longer We thank the NSF (DEB 0108118) for funding, along with time series across large spatial scales. We are currently the University of Pittsburgh and the Pymatuning Laboratory amassing such data. For now, however, we suggest that of Ecology for financial and logistical support. We thank mosquito outbreaks are likely to occur following drought C. Goss, J. Butlzer, and E. Bond for help with fieldwork and years so long as: (1) suitable larval habitats, and the larval for valuable discussions. We also thank L. Blaustein, J. Losos, stage in general, are limiting to overall mosquito popula- R. Ricklefs, and anonymous reviewers for comments. This is tion dynamics, (2) some mosquito species can potentially Pymatuning Laboratory of Ecology Publication no. 143. 2003 Blackwell Publishing Ltd/CNRS 14610248, 2003, 11, Downloaded from https://onlinelibrary.wiley.com/doi/10.1046/j.1461-0248.2003.00533.x by Florida Atlantic University Libraries, Wiley Online Library on [30/08/2023]. See the Terms and Conditions (https://onlinelibrary.wiley.com/terms-and-conditions) on Wiley Online Library for rules of use; OA articles are governed by the applicable Creative Commons License Drought-induced mosquito outbreaks in wetlands 1023 Kiflawi, M., Blaustein, L. & Mangel, M. (2003b). Oviposition REFERENCES habitat selection by the mosquito Culiseta longiareolata in response Angelon, K.A. & Petranka, J.W. (2002). Chemicals of predatory to risk of predation and conspecific larval density. Ecol. Entomol., mosquitofish (Gambusia affinis) influence selection of oviposition 28, 168–173. site by Culex mosquitoes. J. Chem. Ecol., 28, 797–806. Lafferty, K.D. & Holt, R.D. (2003). How should environmental Aniedu, I. (1992). A comparative study of the distribution and stress affect the population dynamics of disease? Ecol. Lett., 6, seasonal abundance of malaria vectors in 3 ecologically distinct 654–664. habitats in Baringo District, Kenya. J. Appl. Entomol., 114, Lindblade, K.A., Walker, E.D., Onapa, A.W., Katungu, J. & 268–274. Wilson, M.L. (1999). Highland malaria in Uganda: prospective Blaustein, L. (1998). Influence of the predatory backswimmer, analysis of an epidemic associated with El Nino. Trans. Roy. Soc. Notonecta maculata, on invertebrate community structure. Ecol. Trop. Med. Hyg., 93, 480–487. Entomol., 23, 246–252. Lounibos, L.P. (1983). The mosquito communities of treeholes in Blaustein, L. & Karban, R. (1990). Indirect effects of the mos- subtropical Florida. In: Phytotelmata: Terrestrial Plants As Host for quitofish Gambusia affinis on the mosquito Culex tarsalis. Limol. Aquatic Insect Communities (eds Frank, J.H. & Lounibos, L.P.). Oceanogr., 35, 767–771. Plexus, Medford, NJ, pp. 223–246. Blaustein, L. & Kotler, B.P. (1993). Oviposition habitat selection Lounibos, L.P. (1985). Interactions influencing production of by the mosquito Culiseta longiareolata: effects of conspecifics, treehole mosquitoes in South Florida. In: Ecology of Mosquitoes: food and green frog tadpoles. Ecol. Entomol., 18, 104–108. Proceedings of a Workshop (eds Lounibos, L.P., Rey J.R. & Frank Blaustein, L. & Margalit, J. (1996). Priority effects in temporary J.H.). Florida Medical Entomology Laboratory, Vero Beach, FL, pools: nature and outcome of mosquito larva toad tadpole pp. 65–67. interactions depend on order of entrance. J. Anim. Ecol., 65, Maguire, M., Skelly, C., Weinstein, P. & Moloney, J. (1999). 77–84. Simulation modelling of Aedes aegypti prevalence, an environ- Bradshaw, W.J. & Holzapfel, C. (1988). Drought and the mental hazard surveillance tool for the control of dengue organization of tree-hole mosquito communities. Oecologia, 74, epidemics. Int. J. Env. Health Res., 9, 253–259. 507–514. Merritt, R.W., Dadd, R.H. & Walker, E.D. (1992). Feeding beha- Carpenter, S.J. & LaCasse, W.J. (1955). Mosquitoes of North America vior, natural food, and nutritional relationships of larval (North of Mexico). University of California Press, Berkeley and mosquitoes. Annu. Rev. Entomol., 37, 349–376. Los Angeles, CA, 360 pp. Mokany, A. & Shine, R. (2003). Oviposition site selection by Chesson, J. (1984). Effect of Notonecta (Hemiptera: Notonectidae) mosquitoes is affected by cues from conspecific larvae and on mosquitoes (Diptera:Culicidae): predation or selective anuran tadpoles. Austral. Ecol., 28, 33–37. oviposition?. Environ. Entomol., 13, 531–538. Mulla, M.S. (1994). Mosquito control then, now, and in the future. Curtis, C. (2002). Molecular medical entomology and the Ôso what?Õ J. Am. Mosq. Control, 190, 574–584. test. Trends Ecol. Evol., 17, 102. Petranka, J.W. & Fakhoury, K. (1991). Evidence of chemically Dhileepan, K. (1996). Mosquito seasonality and arboviral disease mediated avoidance response of ovipositing insects to blue-gills incidence in Murray valley, southeast Australia. Med. Vet. and green frog tadpoles. Copeia, 1991, 234–239. Entomol., 10, 375–384. Rose, R.I. (2001). Pesticides and public health: integrated methods Edgerly, J.S., McFarland, M., Morgan, P. & Livdahl, T. (1998). A of mosquito management. Emerg. Infect. Diseases, 7, 17–23. seasonal shift in egg-laying behaviour in response to cues of Schneider, D.W. & Frost, T.M. (1996). Habitat duration and future competition in a treehole mosquito. J. Anim. Ecol., 67, community structure in temporary ponds. J. N. Am. Benth. Soc., 805–818. 15, 64–86. Enserink, M. (2002). Malaria: ecologists see flaws in transgenic Shaman, J., Day, J.F. & Stieglitz, M. (2002). Drought induced mosquito. Science, 297, 30–31. amplification of Saint Louis encephalitis virus in Florida. Emerg. Fagan, W.F., Meir, E., Prendergast, J., Folarin, A. & Karieva, P. Infect. Dis., 8, 575–580. (2001). Characterizing population vulnerability for 758 species. Spencer, M., Blaustein, L., Schwartz, S.S. & Cohen, J.E. (1999). Ecol. Lett., 4, 132–138. Species richness and the proportion of predatory animal species Gleiser, R.M., Gorla, D.E. & Schelotto, G. (2000). Population in temporary freshwater pools: relationships with habitat size dynamics of Aedes albifasciatus (Diptera: Culicidae) South of and permanence. Ecol. Lett., 2, 157–166. Mar Chiquita Lake, Central Argentina. J. Med. Entomol., 37, Spencer, M., Blaustein, L. & Cohen, J.E. (2002). Oviposition 21–26. habitat selection by mosquitoes (Culiseta longiareolata) and Hay, S.I., Myers, M.F., Burke, D.S., Vaughn, D.W., Endy, T., consequences for population size. Ecology, 83, 669–679. Ananda, N. et al. (2000). Etiology of interepidemic periods Spielman, A. & D’Antonio, M. (2001). Mosquito: A Natural History of of mosquito-borne disease. Proc. Nat. Acad. Sci. (USA), 97, Our Most Persistent and Deadly Foe. Hyperion, New York, NY. 9335–9339. Stav, G., Blaustein, L. & Margalit, Y. (2000). Influence of nymphal Hay, S.I., Cox, J., Rogers, D.J., Randolph, S.E., Stern, D.I., Shanks, Anax imperator (Odonata: Aeshnidae) on oviposition by the D.W. et al. (2002). Climate change and the resurgence of malaria mosquito Culiseta longiareolata (Diptera: Culicidae) and commu- in the East African highlands. Nature, 415, 905–909. nity structure in temporary pools. J. Vector Ecol., 25, 190–202. Kilfawi, M., Blaustein, L. & Mangel, M. (2003a). Predation- Tudhope, A.W., Chilcott, C.P., McCulloch, M.T., Cook, E.R., dependant oviposition habitat selection by the mosquito Chappell, J., Ellam, R.M. et al. (2001). Variability in the El Nino- Culiseta longiareolata: a test of competing hypotheses. Ecol. Lett., 6, Southern oscillation through a glacial–interglacial cycle. Science, 35–40. 291, 1511–1517. 2003 Blackwell Publishing Ltd/CNRS 14610248, 2003, 11, Downloaded from https://onlinelibrary.wiley.com/doi/10.1046/j.1461-0248.2003.00533.x by Florida Atlantic University Libraries, Wiley Online Library on [30/08/2023]. See the Terms and Conditions (https://onlinelibrary.wiley.com/terms-and-conditions) on Wiley Online Library for rules of use; OA articles are governed by the applicable Creative Commons License 1024 J. M. Chase and T. M. Knight Vandyk, J.K. & Rowley, W.A. (1995). Response of Iowa mosquito Williams, D.D. (1996). Environmental constraints in temporary populations to unusual precipitation patterns as measured by New fresh waters and their consequences for the insect fauna. J. N. Jersey light trap collections. J. Am. Mosq. Control, 11, 200–205. Am. Benth. Soc., 15, 634–650. Webb, C.E. & Russell, R.C. (1999). Towards management of mosquitoes at Homebush Bay, Sydney, Australia. I. Seasonal activity and relative abundance of adults of Aedes vigilax, Culex sitiens, and other salt-marsh species, 1993-94 through 1997–98. Editor, L. Blaustein J. Am. Mosq. Control, 15, 242–249. Manuscript received 19 August 2003 Wegbreit, J. & Reisen, W.K. (2000). Relationships among weather, mosquito abundance, and encephalitis virus activity in First decision made 21 August 2003 California: Kern County 1990–98. J. Am. Mosq. Control, 16, Manuscript accepted 8 September 2003 22–27. Wellborn, G.A., Skelly, D.K. & Werner, E.E. (1996). Mechanisms Fast track submitted and reviewed creating community structure across a freshwater habitat gra- dient. Ann. Rev. Ecol. Syst., 27, 337–363. 2003 Blackwell Publishing Ltd/CNRS