Origin and evolution of life on Earth PDF
Document Details
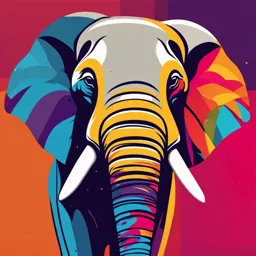
Uploaded by ResplendentPanFlute
University of Ruhuna
Tags
Summary
This document discusses the origin and evolution of life on Earth, covering topics like the pre-biotic Earth, hypotheses on the beginnings of life, including the Oparin-Haldane hypothesis. It also explores the development of cells, from prokaryotes to eukaryotes, and the formation of organelles like mitochondria and chloroplasts through endosymbiosis.
Full Transcript
B.Sc. General Degree- Level I-Semester I BOT1112 Plant Diversity, Unity and Evolution Prof. Shirani Widana Gamage Department of Botany, Faculty of Science University of Ruhuna September 2024 Plant Diversity, Unity and Evolution Course content (...
B.Sc. General Degree- Level I-Semester I BOT1112 Plant Diversity, Unity and Evolution Prof. Shirani Widana Gamage Department of Botany, Faculty of Science University of Ruhuna September 2024 Plant Diversity, Unity and Evolution Course content (30 h) Evolution of life on earth History of taxonomy Six-kingdom and three-domain system of classification Structural and reproductive diversity in domain bacteria Vegetative diversity of blue green bacteria (Cyanobacteria) Domain Archaea; diversity and interesting features Structural and reproductive diversity in fungi General characters, thallus organization, nutrition, and reproduction of Lichens Vegetative and reproductive diversity in algae Vegetative and reproductive diversity in Bryophyta Structure, life cycle and biological importance of Pteridophyta Gymnospermae Angiospermae Evaluation: End semester examination : 70% and Continuous assessments : 30% The Biosphere: Life on Earth Life! It's everywhere on Earth poles to the equator bottom of the sea to several miles in the air undersea thermal vents freezing waters to dry valleys groundwater thousands of feet below the Earth's surface Over the last 3.8 billion years, living organisms on Earth have first appeared and adapted to almost every environment imaginable The diversity of life is truly amazing, but all living organisms do share certain similarities All living organisms can replicate (self-replicating) and the replicator molecule is DNA DNA transfer genetic information from one generation to the next They are self-sustaining Timeline of Earth’s history and life on Earth The pre-biotic Earth Earth is about 4.6 billion years old and it has been changing all the time About 1 billion years thereafter, it started to cool and an atmosphere consisting of hydrogen and helium, and continental crust was formed Then about 3.8 billion years ago the life stated to begin At this stage, the Earth was constantly subjected to intense electrical storms, meteoric impacts and volcanic eruptions Also subjected to ultra-violet (UV) and gamma radiation because the Earth was not shielded by the oxygen-rich atmosphere that we have now There was a constant input of molecules/elements from the volcanic eruptions and complex organic molecules from meteorites Energy of radiation and storms and volcanic eruptions forced those chemical elements to combine or compounds to break apart As a result simple molecules like sugars, amino acids, nucleotides, fatty acids were formed spontaneously These chemical reactions happened again and again so that the products of particular reactions became more and more abundant on Earth It was still hot because of high levels of carbon dioxide and methane produced by volcanic activity in the atmosphere Hydrogen, hydrogen sulfide, hydrogen cyanide and formaldehyde were also present Together with other gases such as ammonia and methane, water formed in the atmosphere, and began to fill the pre-biotic ocean basins But this was not yet life! Pre-biotic ocean basin How might life have arisen? We do not know for sure There are several hypothesis Oparin-Haldane hypothesis In the 1920s, Russian scientist Aleksandr Oparin and English scientist J. B. S. Haldane both separately proposed that life on Earth could have arisen step-by- step from non-living matter through a process of “gradual chemical evolution” Oparin and Haldane thought that the early Earth had a reducing atmosphere (oxygen-poor atmosphere) in which molecules tend to donate electrons Simple inorganic molecules could have reacted (with energy from lightning or the sun) to form building blocks like amino acids and nucleotides, which could have accumulated in the oceans, making a "primordial soup" These building blocks might have combined in further reactions, forming larger, more complex molecules (polymers) like proteins and nucleic acids The polymers could have assembled into units or structures that were capable of self-sustaining and replicating Oparin thought these might have been “colonies” of proteins clustered together to carry out metabolism Haldane suggested that macromolecules became enclosed in membranes to make cell-like structures The details of this hypothesis are probably not quite correct Present day geologists think the early atmosphere was not reducing But the basic idea – a stepwise, spontaneous formation of simple, then more complex, then self- sustaining biological molecules or assemblies – is still the most acceptable origins-of-life hypotheses today Miller and Urey hypothesis In 1953, Stanley Miller and Harold Urey did an experiment to test Oparin and Haldane’s ideas They found that organic molecules could be spontaneously produced under reducing conditions similar to what happened in the pre-biotic earth Miller and Urey built a closed system containing a heated pool of water and a mixture of gases that were thought to be abundant in the atmosphere of early earth (H2O, NH3,CH4, H2) To simulate the lightning that might have provided energy for chemical reactions in Earth’s early atmosphere, Miller and Urey sent sparks of electricity through their experimental system After letting the experiment to run for a week, Miller and Urey found that various types of amino acids, sugars, lipids and other organic molecules had formed But large, complex molecules like DNA and protein were missing But the Miller-Urey experiment showed that at least some of the building blocks for these molecules could form spontaneously from simple compounds Scientists now think that the atmosphere of early Earth was different than in Miller and Urey's setup (that is, not reducing, and not rich in ammonia and methane) So, it's doubtful that Miller and Urey did an accurate simulation of conditions on early Earth However, a variety of experiments done later have shown that organic building blocks (especially amino acids) can form from inorganic precursors From these experiments, it seems reasonable to imagine that at least some of life's building blocks could have formed abiotically on early Earth However, exactly how (and under what conditions) remains an open question How could monomers (building blocks) like amino acids or nucleotides have assembled into polymers, or actual biological macromolecules, on early Earth? In cells today, polymers are put together by enzymes But, since the enzymes themselves are polymers, this is kind of a chicken-and-egg problem! In the 1950s, biochemist Sidney Fox and his colleagues suggested that, on early Earth, ocean water carrying amino acids could have splashed onto a hot surface like a lava flow, boiling away the water and formed protein If we imagine that polymers were able to form on early Earth, still there is a question, How the polymers would have become self-replicating or self-sustaining, meeting the most basic criteria for life? This is an area in which there are many ideas, but little certainty about the correct answer RNA world hypothesis One possibility is that the first life forms were self- replicating nucleic acids, such as RNA or DNA, and that other elements were later add-on to this basic system Many scientists who believe in this hypothesis think that RNA, not DNA, was the first genetic material This is known as the RNA world hypothesis Scientists favor RNA over DNA as the first genetic molecule for several reasons The most important reason is that RNA can, in addition to carrying information, act as a catalyst In contrast, we don’t know of any naturally occurring catalytic DNA molecules RNA catalysts are called ribozymes, and they could have played key roles in the RNA world A catalytic RNA may have catalyzed a chemical reaction to copy itself Such a self-replicating RNA could pass genetic material from generation to generation, fulfilling the most basic criteria for life and, potentially, undergoing evolution Interactions between RNA and amino acids then evolved into the present-day genetic code Later, DNA eventually replaced RNA as the genetic material Metabolism-first hypothesis It suggests that self-sustaining networks of metabolic reactions may have been the first simple life These networks might have formed, near undersea hydrothermal vents that provided a continual supply of chemical precursors, and might have been self- sustaining and persistent (meeting the basic criteria for life) Initially, simple pathways might have produced molecules that acted as catalysts for the formation of more complex molecules Eventually, the metabolic networks might have been able to build large molecules such as proteins and nucleic acids Later "individuals" enclosed by membranes would have been formed Origin and Evolution of Cells ▪ Cells are divided into two main classes, defined by whether they contain a nucleus ▪ Prokaryotic cells lack a nucleus surrounded by an envelope ▪ Eukaryotic cells have a nucleus in which the genetic material is separated from the cytoplasm Characteristic Prokaryote Eukaryote Nucleus Absent Present Cell diameter ≈1μm 10–100 μm Cytoskeleton Absent Present Cytoplasmic Absent Present organelles DNA content 1 × 106 to 5 × 106 1.5 × 107 to 5 × 109 Chromosomes Single circular DNA Multiple linear molecule DNA molecules In spite of these differences, the same basic molecular mechanisms govern the lives of both prokaryotes and eukaryotes This indicates that all present-day cells are descended from a single primordial ancestor How did this first cell develop? And how did the complexity and diversity exhibited by present-day cells evolve? Life first emerged at least 3.8 billion years ago, approximately 750 million years after Earth was formed How life originated and how the first cell came into existence are matters of speculation, since these events cannot be reproduced in the laboratory Evolution of cell; formation of cell The first cell is presumed to have arisen by enclosing self-replicating RNA in a membrane composed of phospholipids Phospholipids are the basic components of all present-day biological membranes, including the plasma membranes of both prokaryotic and eukaryotic cells The key characteristic of the phospholipids is that they are amphipathic One portion of the molecule is soluble in water and another portion is not Phospholipids have long, water-insoluble (hydrophobic) hydrocarbon chains joined to water- soluble (hydrophilic) head groups that contain phosphate When placed in water, phospholipids spontaneously aggregate into a bilayer with their phosphate-containing head groups on the outside in contact with water and their hydrocarbon tails in the interior in contact with each other Such a phospholipid bilayer forms a stable barrier between two aqueous compartments—for example, separating the interior of the cell from its external environment Enclosure of self-replicating RNA and associated molecules in a phospholipid membrane would thus have maintained them as a unit, capable of self-reproduction and further evolution RNA-directed protein synthesis may already have evolved by this time This could be the first cell consisted of self- replicating RNA and proteins Evolution of cell; evolution of metabolism Because cells originated in ocean containing a variety of organic molecules, they were able to obtain food and energy directly from their environment But such a situation is self-limiting, so cells needed to evolve their own mechanisms for generating energy and synthesizing the molecules necessary for their replication Generation and controlled utilization of metabolic energy is important to all cell activities What is this energy source and how it is synthesized? All cells use adenosine 5′-triphosphate (ATP) as their source of metabolic energy for the synthesis of cell constituents and carry out other energy- requiring activities, such as movement Mechanisms used by cells for generating ATP are thought to have evolved in three stages during evolution; glycolysis, photosynthesis, and oxidative metabolism Development of these metabolic pathways changed Earth's atmosphere, thereby altered the course of further evolution In primitive Earth, first energy-generating reactions may be breaking down of organic molecules in the absence of oxygen These reactions may be similar to the glycolysis that we know today which is anaerobic breakdown of glucose to lactic acid, with the net energy gain of two molecules of ATP Energy produced by glycolysis could then be used as a source of energy to drive other metabolic reactions Development of photosynthesis is generally thought to have been the next major evolutionary step The first photosynthetic bacteria evolved more than 3 billion years ago Before that, early cells may have utilized H2S to convert CO2 to organic molecules; This pathway is still used by some bacteria [eg. Sulfur bacteria] Later, organic compounds were synthesized using H2O as a donor of electrons and convert CO2 to organic compounds; photosynthesis Photosynthesis produced free-O2 as the by-product Photosynthesis is thought to have been responsible for making O2 abundant in Earth's atmosphere In the presence of O2, cells evolved and led to the development of oxidative metabolism In oxidative metabolism O2 break down organic compounds to synthesize chemical energy Oxidative metabolism generate energy from organic molecules much more efficient than anaerobic glycolysis Complete oxidative breakdown of glucose to CO2 and H2O yields energy equivalent to that of 36 to 38 molecules of ATP, in contrast to the 2 ATP molecules formed by anaerobic glycolysis Present-day cells use oxidative reactions as their principal source of energy Present-Day Prokaryotes Present-day prokaryotes include all archaebacteria and eubacteria The largest and most complex prokaryotes are the cyanobacteria, in which photosynthesis evolved Prokaryotic cells are surrounded by a rigid cell wall composed of polysaccharides and peptides Within the cell wall is the plasma membrane, which is a bilayer of phospholipids and associated proteins Cell wall is porous and through which a variety of molecules can pass through Plasma membrane provides the functional separation between the inside of the cell and its external environment DNA is a single circular molecule in the nucleoid, which is not surrounded by a membrane separating it from the cytoplasm Cytoplasm contains approximately 30,000 ribosomes (the sites of protein synthesis) Evolution of eukaryotic cell Eukaryotic cell developed at least 2.7 billion years ago, which was 1-1.5 billion years of prokaryotic evolution Phylogenetics studies showed that archaebacteria, eubacteria, and eukaryotes descended from a common ancestor Interestingly, many archaebacterial genes are more similar to those of eukaryotes than to those of eubacteria This indicates that archaebacteria and eukaryotes share a common line of evolutionary descent and are more closely related to each other than either is to the eubacteria Like prokaryotic cells, all eukaryotic cells are surrounded by plasma membranes and contain ribosomes Eukaryotic cells are much more complex and contain a nucleus, a variety of cytoplasmic organelles, and a cytoskeleton The largest and most prominent organelle of eukaryotic cells is the nucleus Nucleus contains the genetic information of the cell, which in eukaryotes is organized as linear rather than circular DNA molecules Nucleus is the site of DNA replication and of RNA synthesis Protein synthesis takes place on ribosomes in the cytoplasm In addition to a nucleus, eukaryotic cells contain a variety of membrane-enclosed organelles in cytoplasm These organelles provide compartments in which different metabolic activities occur Compartmentalization allows eukaryotic cells to function efficiently Mitochondria and chloroplasts, play critical roles in energy metabolism Mitochondria are found in all eukaryotic cells, are the sites of oxidative metabolism Chloroplasts are the sites of photosynthesis and are found only in the cells of plants and green algae Lysosomes and peroxisomes also provide specialized metabolic compartments for the digestion of macromolecules and for various oxidative reactions Plant cells contain large vacuoles that perform a variety of functions, digestion of macromolecules storage of both waste products and nutrients Because of the size and complexity of eukaryotic cells, transport of proteins to their correct destinations within the cell is a challenging task Two cytoplasmic organelles, endoplasmic reticulum (ER) and the Golgi apparatus, are specifically devoted to the sorting and transport of proteins ER is an extensive network of intracellular membranes, extending from the nuclear membrane throughout the cytoplasm ER functions not only in the processing and transport of proteins, but also in the synthesis of lipids From ER, proteins are transported to the Golgi apparatus, where they are further processed and sorted for transport to their final destinations In addition to this role in protein transport, the Golgi apparatus serves as a site of lipid and some of the polysaccharide synthesis in plant cells Eukaryotic cells have another level of internal organization: the cytoskeleton, a network of protein filaments extending throughout the cytoplasm Cytoskeleton provides the structural framework of the cell, determining cell shape and the general organization of the cytoplasm Cytoskeleton is responsible for, movements of entire cells intracellular transport positioning of organelles and other structures, including movements of chromosomes during cell division How organelles were formed in eukaryotic cells A critical step in the evolution of eukaryotic cells is the acquisition of membrane-enclosed organelles such as mitochondria and chloroplast These organelles are thought to have been acquired as a result of the association of prokaryotic cells with the ancestor of eukaryotes; endosymbiosis Mitochondria and chloroplasts are thought to have evolved from bacteria living in large cells Evidences: Both mitochondria and chloroplasts are similar to bacteria in size Like bacteria, they reproduce by dividing in two Both mitochondria and chloroplasts contain their own DNA Mitochondrial and chloroplast DNAs are replicated each time the organelle divides Their genes are translated on organelle ribosomes to synthesize proteins Structure of mitochondria Mitochondria and chloroplasts contain their own genetic systems, which are different from the nuclear genome of the cell Ribosomes and ribosomal RNAs of these organelles are more closely related to those of bacteria than to those encoded by the nuclear genomes of eukaryotes. An endosymbiotic origin for these organelles is now generally accepted Mitochondria thought to have evolved from aerobic bacteria Chloroplasts from photosynthetic bacteria, such as the cyanobacteria Development of multicellular organisms Eukaryotes are either unicellular or multicellular The simplest eukaryotes are the unicellular yeasts Yeasts are more complex than bacteria, but much smaller and simpler than the cells of animals or plants Multicellular organisms evolved from unicellular eukaryotes at least 1.7 billion years ago Some unicellular eukaryotes form multicellular aggregates (colonies) May be they are in the transition stage of the evolution to form multicellular organisms from single cells Cells of many algae (e.g., the green alga Volvox) associate with each other to form multicellular colonies During evolution, cells in the colonies specialized to do certain function and led to form multicellular organisms Continuing cell specialization would have formed present-day plants and animals, including human beings Evolution is a continuing process, where it leads to? Classification of living organisms For easy identification and understanding organisms are classified into different groups Carolus Linnaeus is the father of classification He was the pioneer of modern biological nomenclature In 1758, he proposed a Two Kingdom Classification He classified all living things into two kingdoms, they are Animal kingdom and Plant kingdom This two kingdom classification laid the foundation for modern classification In his classification, he has distinguished clearly animals from plants The plants synthesize their own food and it is fixed in the soil. There is no movement in the plants On the other hand, animals are depending on other plants and animals for their food. Animals are freely movable The two-kingdom classification lasted for a very long time but was replaced because it did not take into account many major parameters while classifying There was no differentiation of the eukaryotes and prokaryotes; neither unicellular and multicellular; nor photosynthetic and the non-photosynthetic There were a lot of organisms which could not be classified as either plants or animals Five-kingdom classification As a result, R. H. Whittaker in 1969 came up with the concept of the five- kingdom classification He considered cell structure, presence of cell wall, mode of reproduction and mode of nutrition in this classification Those five-kingdom were, Monera Protista Fungi Plantae Animalia Kingdom Monera The bacteria/propkaryotes were categorized into the Kingdom Monera They possess the following important features: Bacteria occur everywhere and they are microscopic in nature They possess a cell wall and are prokaryotic The cell wall is formed of amino acids and polysaccharides They can be heterotrophic and autotrophic The heterotrophic bacteria can be parasitic or saprophytic. The autotrophic bacteria can be chemosynthetic or photosynthetic Kingdom Protista Protista has the following important features: They are unicellular and eukaryotic organisms Some of them have cilia or flagella for mobility Sexual reproduction is by a process of cell fusion and zygote formation They include desmids, diatoms, dinoflagellates, euglenoids, slim molds and protozoans Kingdom Fungi The kingdom fungi include moulds, mushroom, yeast etc. Fungi have following important features: The fungi are filamentous, excluding yeast (single-celled) Comprises slender, long thread-like constructions called hyphae. The web of hyphae is called mycelium Some of the hyphae are unbroken tubes which are jam-packed with multinucleated cytoplasm called coenocytic hyphae The other type of hyphae has cross-walls or septae The cell wall of fungi is composed of polysaccharides and chitin Most of the fungi are saprophytes and are heterotrophic Some of the fungi also survive as symbionts. Some are parasites Kingdom Plantae Features of Kingdom Plantae: All eukaryotes which have chloroplast Most of them are autotrophic in nature, but some are heterotrophic as well The Cell wall mainly comprises cellulose Plants have two distinct phases in their lifecycle These phases alternate with each other The diploid saprophytic and the haploid gametophytic phase The lengths of the diploid and haploid phases vary among dissimilar groups of plants Kingdom Animalia Features of Kingdom Animalia: All multicellular eukaryotes which are heterotrophs and lack cell wall The animals are directly or indirectly dependent on plants for food. Their mode of nutrition is holozoic. Holozoic nutrition encompasses ingestion of food and then the use of an internal cavity for digestion of food Many of the animals are adept for locomotion They reproduce by sexual mode of reproduction Six kingdom Three-domain system of biological classification The five kingdom classification does not mention about how organisms within Kingdoms or between kingdoms may be related to each other via evolutionary relationships Therefore, in 1990, Carl Woese proposed Six Kingdom and Three Domain Classification based on the genetic characteristics (rather than phenotypic ones) with respect to evolutionary relatedness (phylogeny) of organisms He believed that all cells came from a common ancestor cell termed the universal common ancestor This universal common ancestor eventually evolved into three different cell types, each representing a domain He separated the monera into archaebacteria and eubacteria based on the ribosomal RNA structure This led to the division of organisms into three domains, archaea, bacteria, and eukarya The key difference from earlier classifications is the separation of of archaea from bacteria This system adds a level of classification (the domains) "above" the kingdoms present in the previously used five- kingdom systems Archaea appear to be more closely related to Eukaryotes than they are to other prokaryotes Hierarchy of biological classification Domain Archaea Kingdom Bacteria Bacteria Eukarya Archaebacteria Protista Fungi Plantae Animalia