Organelle Organization (PDF)
Document Details
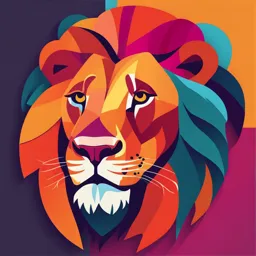
Uploaded by KindlyElegy
Tags
Related
Summary
This document discusses the intracellular organization and transport of proteins in eukaryotic cells. It details the various mechanisms of protein movement between different compartments, including translocation, gated transport, vesicular transport, and engulfment. The mechanisms are described with a focus on the role of sorting signals and sorting receptors in guiding proteins to their correct cellular locations.
Full Transcript
Chapter 12-13 Intracellular Organization and trafficking Copyright © 2022 W. W. Norton & Company, Inc. THE COMPARTMENTALIZATION OF CELLS All Eukaryotic Cells Have the Same Basic Set of Membrane- enclosed Organelles Major intracellular membrane-enclosed compartments of an animal...
Chapter 12-13 Intracellular Organization and trafficking Copyright © 2022 W. W. Norton & Company, Inc. THE COMPARTMENTALIZATION OF CELLS All Eukaryotic Cells Have the Same Basic Set of Membrane- enclosed Organelles Major intracellular membrane-enclosed compartments of an animal cell Membrane enclosures allow these compartments to have an environment inside that is different from the surroundings Remember that a lot happens at the membranes too, not just inside the organelles!! Major intracellular membrane-enclosed compartments of an animal cell The secretory pathway has an environment inside its membrane bound lumens that is very similar to the outside. In addition to organelles, cells also have BIOMOLECULE CONDENSATES, or places with high concentrations of certain nucleic acids + proteins, serving as biochemical factories BIOMOLECULE CONDENSATES Contain a series of macromolecules (nucleic acids and/or proteins) that serve as a scaffold for the whole condensate These scaffolds attract and recruit other proteins and/or nucleic acids that will perform a specific task This helps concentrate all components required for an specific task in a small place, further facilitating it For the nucleolus, more than 400 proteins and RNAs are recruited to help process pre-rRNA and assemble ribosomes. The pre-rRNA transcript acts as a scaffold to recruit other proteins like ribosomal proteins or other RNAs line snoRNA (small nucleolar RNA) that are required for ribosome creation NUCLEOLUS: EXAMPLE OF BIOMOLECULE CONDENSATES https://mmegias.webs.uvigo.es/02-english/5-celulas/4-nucleolo.php https://mmegias.webs.uvigo.es/02-english/5-celulas/4-nucleolo.php BIOMOLECULE CONDENSATES are BIOCHEMICAL FACTORIES https://mmegias.webs.uvigo.es/02-english/5-celulas/4-nucleolo.php THE COMPARTMENTALIZATION OF CELLS Proteins Can Move Between Compartments in Different Ways A simplified “road map” of protein traffic within a eukaryotic cell Proteins can move from one compartment to another by protein translocation (blue), gated transport (red), vesicular transport (green), or engulfment (gray) The sorting signals that direct a given protein’s movement through the system, and thereby determine its eventual location in the cell, are contained in each protein’s amino acid sequence The journey begins with the synthesis of a protein on a ribosome in the cytosol and, for many proteins, terminates when the protein reaches its final destination Other proteins shuttle back and forth between the nucleus and cytosol A simplified “road map” of protein traffic within a eukaryotic cell At each intermediate station (boxes), a decision is made as to whether the protein is to be retained in that compartment or transported further. A sorting signal may direct either retention in or exit from a compartment A special transport process termed “engulfment” is used to move proteins from the cytosol into the lysosome in autophagy or used to enclose chromosomes inside the nucleus during nuclear envelope re-formation after mitosis TRANSLOCATION Transmembrane proteins called protein translocators directly transport protein from the cytosol to the organelle. Entry into the secretory pathway through the ER is an example of this, just like entry into mitochondria and peroxisomes Protein is either co-translationally translocated (ER) or it is first synthesized in the cytosol and it is translocated unfolded inside the organelle GATED TRANSPORT Exclusive for the Nucleus-cytosol trafficking Nuclear pore function as gates that regulate the passage between the two spaces It is so big that proteins can pass fully folded and even bound to others in complexes VESICLE TRANSPORT Membrane-enclosed transport intermediates. Often spherical vesicles but they can also be elongated tubules or irregularly shaped fragments of organelles Vesicle budding and fusion during vesicular transport Transport vesicles bud from one compartment (donor) and fuse with another topologically equivalent (target) compartment. Vesicle budding and fusion during vesicular transport In the process, a subset of soluble components (red dots) are transferred from lumen to lumen. Vesicle budding and fusion during vesicular transport Note that membrane is also transferred and that the original orientation of both proteins and lipids in the donor compartment membrane is preserved in the target compartment membrane. Vesicle budding and fusion during vesicular transport Membrane proteins retain their asymmetrical orientation, with the same domains always facing the cytosol. ENGULFMENT Double-membrane sheets wrap around portions of the cytoplasm ENGULFMENT Double-membrane sheets wrap around portions of the cytoplasm ENGULFMENT Three examples: 1. Autophagy 2. Lysosome 3. During the end of mitosis, portions of the ER membrane engulf decondensing chromosomes to restore the nucleus THE COMPARTMENTALIZATION OF CELLS Sorting Signals Direct Proteins to the Correct Cell Address Sorting signals are recognized by Sorting Receptors present at specific organelles Examples of signal sequences that direct proteins to different intracellular locations Negatively charged amino acids Positively charged amino acids Hydrophobic amino acids Important uncharged polar amino acids For ER Resident proteins (at the C- Terminus) THE ENDOPLASMIC RETICULUM Important role in biosynthesis of both lipids and proteins It also stores Ca2+ that will be used in many cell processes Sarcoplasmic storing and releasing calcium Usually, half of the total membrane in a cell Organized as an interconnected network of tubules and flattened sacs that are continuous and the membrane is connected to the outer nuclear membrane The ER extends as a network of tubules and sheets throughout the entire cytosol, so that all regions of the cytosol are close to some portion of the ER membrane Site of production of (1) proteins that need to be secreted, (2) Resident proteins of the ER, Golgi and organelles derive from the ER (3) many transmembrane proteins and lipids of the ER, Golgi, mitochondria, lysosomes, endosomes, transport vesicles, peroxisome, plasma membrane. THE ENDOPLASMIC RETICULUM The ER Is Structurally and Functionally Diverse: Rough ER: with ribosomes: Secretory Pathway Smooth ER: without ribosomes: Lipid biosynthesis and metabolism The proportion between them varies from cell to cell: Exocrine cell in the pancreas: 60% of cell membrane is rough ER Similar % for insulin or antibody secreting cells Cells that made steroid hormones have large amounts of smooth ER Muscle cells have special smooth ER called sarcoplasmic reticulum involved in release/uptake of Ca2+for muscle contraction The smooth ER can extend and make contact sites with mitochondria and the plasma membrane These contacts facilitate the transfer of lipids synthesized at the ER into mitochondria and plasma membrane THE ENDOPLASMIC RETICULUM The ER Assembles Most Lipid Bilayers The synthesis of phospholipids at the ER membrane fatty acids delivered to the ER by a cytosolic fatty acid binding protein are linked to glycerol 3-phosphate to produce phosphatidic acid, which serves as a precursor to make other phospholipids that differ in the structures of their polar head groups. Notice how the lipids are only formed in one of the two layers of the bilayer ER membrane, the one facing the cytosol THE ENDOPLASMIC RETICULUM Membrane Contact Sites Between the ER and Other Organelles Facilitate Selective Lipid Transfer PROTEINS PRESENT IN THE ER Proteins that we can find in the ER Proteins in the lumen that need to be secreted Proteins residents on the ER lumen Proteins in the membrane that need to be located in the plasma membrane Proteins in the membrane that are resident of the ER membrane PROTEINS IN THE ER LUMEN TO BE SECRETED CONTAIN AN ER SIGNAL SEQUENCE AND UNDERGO CO-TRANSLATIONAL TRANSLOCATION PROTEINS PRESENT IN THE ER Translocation Across the ER Membrane Does Not Always Require Ongoing Polypeptide Chain Elongation SOME PROTEINS ARE TRANSPORTED TO THE LUMEN AFTER TRANSLATION WITH THE HELP OF CHAPERONS: POST-TRANSLATION LOCALIZATION Keep the protein unfolded SOME PROTEINS ARE TRANSPORTED TO THE LUMEN AFTER TRANSLATION WITH THE HELP OF CHAPERONS: POST-TRANSLATION LOCALIZATION Notice how they still have the ER signal PROTEINS PRESENT IN THE ER Transmembrane Proteins Contain Hydrophobic Segments That Are Recognized Like Signal Sequences MEMBRANE PROTEINS CONTAIN HYDROPHOBIC TRANSMEMBRANE DOMAINS THAT EMBED THEM IN THE ER MEMBRANE IN SOME CASES, IN A SINGLE –PASS MEMBRANE PROTEIN THE TRANSMEMBRANE DOMAIN CAN ACT AS AN ER SIGNAL (it is also hydrophobic) The transmembrane segment is recognized by SRP (not shown) and delivered via the SRP receptor to the translocator at the ER membrane DEPENDING ON THE ORIENTATION THAT IT ENTERS THE TRANSLOCON, IT CAN HAVE THE N-TERMINUS IN THE LUMEN OR IN THE CYTOSOL DEPENDING ON THE ORIENTATION THAT IT ENTERS THE TRANSLOCON, IT CAN HAVE THE N-TERMINUS IN THE LUMEN OR IN THE CYTOSOL Notice how the surroundings of the transmembrane domain will determine the orientation PROTEINS PRESENT IN THE ER Hydrophobic Segments of Multipass Transmembrane Proteins Are Interpreted Contextually to Determine Their Orientation PROTEINS PRESENT IN THE ER Some Proteins Are Integrated Into the ER Membrane by a Post- translational Mechanism In this post-translational pathway for the insertion of tail-anchored membrane proteins into the ER, a soluble pre- targeting complex captures the hydrophobic C-terminal transmembrane segment (red) after it emerges from the ribosomal exit tunnel and loads it onto the Get3 targeting factor. The resulting complex is targeted to the ER membrane by interaction with the Get1–Get2 receptor complex, which functions as a membrane protein insertion machine. After the tail- anchored protein is released from Get3 and inserted into the ER membrane DEPENDING ON THE ORIENTATION THAT IT ENTERS THE TRANSLOCON, IT CAN HAVE THE N-TERMINUS IN THE LUMEN OR IN THE CYTOSOL NOTICE HOW MOST OF THE PROTEIN IS FACING THE CYTOSOL, NOT THE ER LUMEN PROTEINS PRESENT IN THE ER Some Membrane Proteins Acquire a Covalently Attached Glycosylphosphatidylinositol (GPI) Anchor The attachment of a GPI anchor to a protein in the ER The attachment of a GPI anchor to a protein in the ER GPI-anchored proteins are targeted to the ER membrane by an N-terminal signal sequence (not shown), integrated into the membrane, and processed by signal peptidase similarly to a single-pass transmembrane protein The attachment of a GPI anchor to a protein in the ER Immediately after the completion of protein synthesis, the precursor protein remains anchored in the ER membrane by a hydrophobic C-terminal sequence of 15–20 amino acids; the rest of the protein is in the ER lumen The attachment of a GPI anchor to a protein in the ER Within less than a minute, a transamidase enzyme in the ER cleaves the protein from its membrane-bound C- terminus and simultaneously attaches the new C-terminus to an amino group on a preassembled GPI intermediate. The attachment of a GPI anchor to a protein in the ER The sugar chain contains an inositol attached to the lipid from which the GPI anchor derives its name The attachment of a GPI anchor to a protein in the ER Because of the covalently linked lipid anchor, the protein remains membrane-bound, with all of its amino acids exposed initially on the lumenal side of the ER and eventually on the exterior of the plasma membrane. PROTEINS PRESENT IN THE ER RESIDENT ER PROTEINS TRANSLOCONS AND RECEPTORS SIGNAL PEPTIDASE CHAPERONS THAT HELP PROPER FOLDING PROTEINS OF THE UPR (UNFOLDED PROTEIN RESPONSE) PROTEINS THAT CATALYZE DISULFIDE BONDS PROTEINS THAT ADD POLYSACCHARIDES TO PROTEINS: Glycosylation Proteases PROTEIN DISULFIDE ISOMERASE (PDI) is a resident ER lumen prtei that catalyzes the formation of the disulfide bond inside the ER THE ER IS FILLED WITH CHAPERONS TO HELP THEM FOLD PROPERLY Polypeptides must assume the correct folding pattern in order to function properly. The correct folding of a protein is mediated by chaperones (they also are proteins--chaperones are abundant in the ER lumen). A completed polypeptide will assume the correct folding pattern spontaneously, however before translation is complete, it could assume an incorrect formation or it could aggregate with other partially made polypeptides. To prevent this, chaperones in the ER (and cytosol) bind to the nascent polypeptide and keep it from interacting with anything until the polypeptide is completely synthesized The chaperons for ER proteins will not fold them right in the cytosol. Chaperons is not enough, the conditions for proper folding need to be present in addition to chaperons Examples: BiP, Calnexin and Calreticulin Adapted from Todd et al. (2008) Nat.Rev.Immunol. 8 663 PROTEINS PRESENT IN THE ER Most Proteins Synthesized in the Rough ER Are Glycosylated by the Addition of a Common N-Linked Oligosaccharide by ER resident proteins Note: Proteins can be glycosylated at Asparagines (N) (N-linked Oligosaccharide) by far the most common Serine, Threonines, hydroxylysine or hydroxyproline. (0-linked Oligosaccharides), less abundants N-linked protein glycosylation in the rough ER occurs as soon as the polypeptide enters the ER lumen The precursor oligosaccharide (shown in color) is attached only to asparagine side chains in the sequences Asn-X-Ser and Asn-X-Thr (where X is any amino acid except proline). These sequences occur much less frequently in glycoproteins than in non-glycosylated cytosolic proteins. Evidently there has been selective pressure against these sequences during protein evolution, presumably because glycosylation at inappropriate sites would interfere with protein folding The five sugars in the gray box form the core region of this oligosaccharide. For many glycoproteins, only the core sugars survive the extensive oligosaccharide trimming that takes place in the Golgi apparatus. Oligosaccharyl Transferase mediates the transfer of the precursor oligosaccharide to the N One copy of this enzyme is associated with each protein translocator in the ER membrane PROTEINS PRESENT IN THE ER Proteins are folded properly at ER because… There is the right environment There are the right modifications: disulfide bonds, glycosylation.. But also because there are many chaperon proteins that aid in the proper folding and that ensure that only proper folding proteins made it out of the ER THE ER IS FILLED WITH CHAPERONS TO HELP THEM FOLD PROPERLY Polypeptides must assume the correct folding pattern in order to function properly. The correct folding of a protein is mediated by chaperones (they also are proteins--chaperones are abundant in the ER lumen). A completed polypeptide will assume the correct folding pattern spontaneously, however before translation is complete, it could assume an incorrect formation or it could aggregate with other partially made polypeptides. To prevent this, chaperones in the ER (and cytosol) bind to the nascent polypeptide and keep it from interacting with anything until the polypeptide is completely synthesized The chaperons for ER proteins will not fold them right in the cytosol. Chaperons is not enough, the conditions for proper folding need to be present in addition to chaperons Examples: BiP, Calnexin and Calreticulin Adapted from Todd et al. (2008) Nat.Rev.Immunol. 8 663 THE ENDOPLASMIC RETICULUM Oligosaccharides Are Used as Tags to Mark the State of Protein Folding THE ENDOPLASMIC RETICULUM Improperly Folded Proteins Are Exported from the ER and Degraded in the Cytosol THE ENDOPLASMIC RETICULUM Misfolded Proteins in the ER Activate an Unfolded Protein Response Chapter 13 Intracellular Membrane Traffic Copyright © 2022 W. W. Norton & Company, Inc. CELLS ARE IN CONSTANT FLUX WITH THE ENVIRONMENT CELLS SECRETE PROTEINS, LIPIDS, AND CARBOHYDRATES TO THE OUTSIDE AND THEY UPTAKE MATERIALS FROM THE OUTSIDE CELLS ALSO NEED TO RENEW MEMBRANE RECEPTORS AND MEMBRANES AT THE SAME TIME CELLS NEED TO RESPOND TO THE ENVIRONMENT AND MAKE APPROPRIATE CHANGES THAT MAY INCLUDE CHANGES OF MEMBRANE PROTEINS, SECRITIONS…. THE RESULT OF ALL THIS IS A CELL THAT IS IN CONSTANT FLUX, CREATING AND SHUFFLING PMATERIALS FROM DIFFERENT COMPARTMENTS IN THE INSIDE OR FROM THE INSIDE TO THE OUTSIDE AND VICEVERSA THE MOST COMMON WAY TO TRANSPORT MOLECULES TO/FROM DIFFERENT COMPARTMENTS AND TO/FROM THE OUTSIDE IS NOT BY CROSSING MEMBRANES BUT BY FUSING MEMBRANES EXAMPLE: EXOCYTOSIS AND ENDOCYTOSIS FUSE MEMBRANES TO SECRETE OR UPTAKE MATERIALS TO OR FROM THE OUTSIDE THE IMPORTANT THING IS THAT THE ENVIRONMENT INSIDE THE VESICLE IS THE SAME AS THE OUTSIDE TRANSPORT VESICLES ARE THE MAIN WAY TO TRANSPORT MATERIALS BETWEEN COMPARTMENTS AND BETWEEN THE INSIDE AND THE OUTSIDE Notice how the environment inside the vesicles and the two compartments in the same The important thing is that the vesicle needs to discriminate what things make it to the vesicle and which one need to be left behind. CELL ROAD MAP: SECRETORY, ENOCYTIC AND RETRIEVAL PATHWAYS SECRETORY PATHWAY: ER contents are transported from the endoplasmic reticulum (ER) to the plasma membrane , extracellular space, or (via endosomes) to lysosomes ENDOCYTIC PATHWAY: molecules are ingested in endocytic vesicles derived from the plasma membrane and delivered to early endosomes and then (via late endosomes) to lysosomes RETRIEVAL PATHWAYS: (1) endocytosed molecules are retrieved from early endosomes and returned (some via recycling endosomes) to the cell surface for reuse; (2) molecules are retrieved from the early and late endosomes and returned to the Golgi apparatus, and (3) some are retrieved from the Golgi apparatus and returned to the ER 2 1 3 The lumens of most membrane-enclosed compartments are topologically equivalent to each other and to the outside of the cell. All compartments shown communicate with one another and the outside of the cell by means of transport vesicles AUTOPHAGY: THE CELLS ”EAT” SOME OF ITS PARTS. MECHANISMS OF MEMBRANE TRANSPORT AND COMPARTMENT IDENTITY The question is: “how does the cell ensure that a transport vesicle goes to the right place?” The question can be also put as how vesicle and compartment identity is achieved. HOW VESICLE AND COMPARTMENT IDENTITY IS ACHIEVED? The answer is a multilayered mechanism The type of coat protein in the vesicle The type of phosphoinositides (PIPs) in the membrane The type of Rab proteins present in different organelles The v-SNARES and t-SNARES present in different vesicles and organelles MECHANISMS OF MEMBRANE TRANSPORT AND COMPARTMENT IDENTITY There Are Various Types of Coated Vesicles There Are Various Types of Coated Vesicles Different coat proteins select different cargo and shape the transport vesicles that mediate the various steps in the secretory, endocytic and retrieval pathways There Are Various Types of Coated Vesicles When the same coats function in different places in the cell, they usually incorporate different coat protein subunits that modify their properties (not shown) There Are Various Types of Coated Vesicles Clathrin–coated vesicles mediate transport originating from the Golgi, endosome and plasma membrane There Are Various Types of Coated Vesicles COPI–coated vesicles mediate transport originating from the Golgi There Are Various Types of Coated Vesicles COPII–coated vesicles mediate transport originating from the ER There Are Various Types of Coated Vesicles Retromer mediate transport from the endosome to the Golgi in the retrieval pathway CLATHRIN-COATED VESICLES The Assembly of a Clathrin Coat Drives Vesicle Formation Clathrin have a heavy chain a light chain that forms heterotrimers called “triskelions” Triskelions can assemble into much bigger geometric structures that coat transport vesicles Triskelion The structure of a clathrin coat Group; reproduced with permission of SNCSC A, from E. Ungewickell and D. Branton, Nature 289:420–422, published 1981 by Nature Publishing An example of clathrin coat composed of 36 triskelions organized in a network of 12 pentagons and 6 hexagons, with some heavy chains and light chains The structure of a clathrin coat Group; reproduced with permission of SNCSC A, from E. Ungewickell and D. Branton, Nature 289:420–422, published 1981 by Nature Publishing The light chains link to the actin cytoskeleton, which helps generate force for membrane budding and vesicle movement, and their phosphorylation regulates clathrin coat assembly The structure of a clathrin coat Group; reproduced with permission of SNCSC A, from E. Ungewickell and D. Branton, Nature 289:420–422, published 1981 by Nature Publishing The interwoven legs of the clathrin triskelions form an outer shell from which the N- terminal domains of the triskelions protrude inward. These domains bind to the adaptor proteins in the membrane of the vesicle CLATHRIN-COATED VESICLES Adaptor Proteins Select Cargo into Clathrin-coated Vesicles Assembly/disassembly of Clathrin-coated vesicle during endocytosis 1. When receptors bind cargo that need to be transported inside during endocytosis, they undergo conformational changes that allows them to bind adaptor proteins Assembly/disassembly of Clathrin-coated vesicle during endocytosis 2. The adaptor proteins bind both clathrin triskelions and membrane-bound cargo receptors, thereby mediating the selective recruitment of both membrane and soluble cargo molecules into the vesicle Assembly/disassembly of Clathrin-coated vesicle during endocytosis The assembly of the coat introduces curvature into the membrane, which leads in turn to the formation of a coated bud. Assembly/disassembly of Clathrin-coated vesicle during endocytosis 3. Other membrane-bending and fission proteins are recruited to the neck of the budding vesicle, where sharp membrane curvature is introduced Assembly/disassembly of Clathrin-coated vesicle during endocytosis 4. The coat is rapidly lost shortly after the vesicle buds off. DYNAMIN in in charge of pinching off clathrin-coated vesicles Multiple dynamin molecules assemble into a spiral around the neck of the forming bud. DYNAMIN in in charge of pinching off clathrin-coated vesicles Activation of the Dynamin GTPase activity induces a conformation changes that strangles the “neck”, bringing the two membranes together and inducing pinching and release Polymerization of actin filaments occurs near the vesicle neck, helping propel the budding vesicle away from the plasma membrane. Don’t forget the motor proteins that help move transport vesicle Another example: Formation of a COPII-coated vesicle Driven by Coat-recruitment GTPases In the case of CopII, the Coat- recruitment GTPase in Sar1 When Sar1-GDP is turned into Sar— GTP by a GEF (sar1-GEF) in the ER membrane it exposes a amphighilic helix that inserts into the cytosol- facing leaflet of the ER membrane GTP-bound Sar1 binds to a complex of two COPII adaptor coat proteins, called Sec23 and Sec24, which form the inner coat. GTP-bound Sar1 binds to a complex of two COPII adaptor coat proteins, called Sec23 and Sec24, which form the inner coat Sec24 has several different binding sites for the cytosolic tails of cargo receptors. Sec23/24 recruit a complex of two additional COPII coat proteins, called Sec13 and Sec 31 that forms the outer shell of the coat Coats do not always form spherical forms and can form larger structures (like the ones required to transport collagen HOW VESICLE AND COMPARTMENT IDENTITY IS ACHIEVED? The answer is a multilayered mechanism The type of coat protein in the vesicle The type of phosphoinositides (PIPs) in the membrane The type of Rab proteins present in different organelles The v-SNARES and t-SNARES present in different vesicles and organelles PHOSPHOINOSITIDES (PIP) Phosphoinositides Mark Organelles and Membrane Domains Phosphoinositides are phosphatidylinositol phosphates (PIPs) A type of lipid that can be found in membranes that can be phosphorylated in multiple places and multiple times The structure of PI shows the free hydroxyl groups in the inositol sugar that can be modified This is just one of the Phosphatidylinositol (PI) Phosphosphoinositide (PIP) possibilities Phosphoinositide head groups are recognized by protein domains that discriminate between the different PIPs forms. In this way, select groups of proteins containing such domains are recruited to regions of membrane in which these phosphoinositides are present Different types of PIPs are located in different membranes and membrane domains, where they are often associated with specific vesicle transport events like adaptor proteins Diferent PIPsà Different Proteins attracted to that area. à Among them there will be different adaptor proteins à different coat proteins attracted to that area EXAMPLE: AP2 is an adaptor protein that mediates assembly of Clathrin-coated vesicles and that is recruited to the right membrane by its interaction with PIP(4,5)2 Please notice that the PIP is only present in one of the Leaflet of the membrane, in this case the one facing the cytosol Answer this question: Why do clathrin-coated vesicles do not form on the ER membrane??? Partly, because the ER membrane does not have PIP(4,5)P2, therefore, adaptors like AP2 are not recruited there and not clathrin forms on the outside Answer this question: Why do clathrin-coated vesicles do not form on the ER membrane??? Please keep track what is inside and what is outside HOW VESICLE AND COMPARTMENT IDENTITY IS ACHIEVED? The answer is a multilayered mechanism The type of coat protein in the vesicle The type of phosphoinositides (PIPs) in the membrane The type of Rab proteins present in different organelles The v-SNARES and t-SNARES present in different vesicles and organelles RAB PROTEINS Rab Proteins are GTP_proteins that Guide Transport Vesicles to Their Target Membrane DIFFERENT RAB PROTEINS ARE LOCATED AT DIFFERENT PLACES Importantly, there are different effectors proteins that interact with different Rab proteins located at different places A certain Rab protein attached to a GTP in a certain vesicle is recognized by a tethering protein that only recognizes that type of Rab protein. This ensures that a vesicle does not fuse with the wrong compartment (because it does not have the right tethering protein and thus it does not recognize the vesicle) SPECIFICITY!!! After fusion, the intrinsic GTP-ase activity og Rab-GDP is activated. RanGDP lacks any affinity to the tethering protein and the vesicle HOW VESICLE AND COMPARTMENT IDENTITY IS ACHIEVED? The answer is a multilayered mechanism The type of coat protein in the vesicle The type of phosphoinositides (PIPs) in the membrane The type of Rab proteins present in different organelles The v-SNARES and t-SNARES present in different vesicles and organelles SNARES SNAREs Mediate Membrane Fusion There are 35 types of SNARES, each associated with a particular organelle and thus, they contribute to identity and specificity They are long helical proteins Depending on localization we can classify them as v-Snares: present in the transport vesicle (only one protein) t-Snares: present in the target compartment (three proteins) One v-snares can interact with 3 t-snares creating a trans-SNARE complex A certain Rab protein attached to a GTP in a certain vesicle is recognized by a tethering protein that only recognizes that type of Rab protein. This ensures that a vesicle does not fuse with the wrong compartment (because it does not have the right tethering protein and thus it does not recognize the vesicle) SPECIFICITY!!! One v-SNARE is the surface of the transport vesicle interacts with t-snares in the target membrane to form a trans-SNARE Works with Rab/tethering protein to ensure specificity and identity how SNARE proteins catalyze membrane fusion? Bilayer fusion occurs in multiple steps. A tight pairing between v- and t-SNAREs forces lipid bilayers into close apposition and expels water molecules from the interface how SNARE proteins catalyze membrane fusion? Lipid molecules in the two interacting (cytosolic) leaflets of the bilayers then flow between the membranes to form a connecting stalk. Lipids of the two noncytosolic leaflets then contact each other, forming a new bilayer, which widens the fusion zone (hemifusion, or half-fusion). Rupture of the new bilayer completes the fusion reaction SNARES Interacting SNAREs Need to Be Pried Apart Before They Can Function Again NSF is the protein that perform this task TRANSPORT FROM THE ENDOPLASMIC RETICULUM THROUGH THE GOLGI APPARATUS TRANSPORT FROM THE ENDOPLASMIC RETICULUM THROUGH THE GOLGI APPARATUS Proteins Leave the ER in COPII-coated Transport Vesicles The recruitment of membrane and soluble cargo molecules into ER transport vesicles is mediated by EXIT Signals These exit signals can be found on soluble ER lumen proteins or proteins attached to membrane. Some of the exit-containing membrane proteins act as cargo receptors The recruitment of membrane and soluble cargo molecules into ER transport vesicles is mediated by EXIT Signals These exit signals can be found on soluble ER lumen proteins or proteins attached to membrane The exit signals are recognized by adaptors proteins that recruit the COPII coat and thus, they concentrate exit- signal containing proteins and their cargos in the place of vesicle formation Other proteins may enter the vesicle by bulk flow A typical 50-nm transport vesicle contains about 200 transmembrane proteins, which can be of many different types Resident proteins do not contain exit signals and thus, they are not recruited to the vesicle Resident chaperons like BiP make help ensure proper foldimg and also act as a floding property control. If a protein is not properly folded is detected by proteins like BiP and sent outside the ER to be degraded before is recruited to the vesicle TRANSPORT FROM THE ENDOPLASMIC RETICULUM THROUGH THE GOLGI APPARATUS Vesicular Tubular Clusters Mediate Transport from the ER to the Golgi Apparatus Homotypic membrane fusion Right after COPII vesicles have been formed, the coat is lost and NSF pries apart identical pairs of v-SNAREs and t-SNAREs in both membranes which leads to membrane fusion and the formation of one continuous compartment Subsequently, the compartment grows by further homotypic fusion (of SNAREs) with vesicles from the same kind of membrane, displaying matching SNARE, forming a vesicular tubular cluster Vesicular tubular clusters move along microtubules to carry proteins from the ER to the Golgi apparatus COPI coats mediate the budding of vesicles that return to the ER from these clusters (and from the Golgi apparatus). = Retrieval Pathway The retrieval pathway ensures that resident proteins that may have leaked into a vesicle are returned. Also ensures that cargo receptors can go back to the ER for more cargo The Retrieval Pathway to the ER Uses Sorting Signals like KDEL present in certain ER resident proteins (like BiP) The KDEL receptor present in both vesicular tubular clusters and the Golgi apparatus captures the soluble ER resident proteins and carries them in COPI-coated transport vesicles back to the ER. (Recall that the COPI-coated vesicles shed their coats as soon as they are formed.) TRANSPORT FROM THE ENDOPLASMIC RETICULUM THROUGH THE GOLGI APPARATUS The Golgi Apparatus Consists of an Ordered Series of Compartments The Golgi Apparatus Consists of an Ordered Series of Compartments The Golgi apparatus consists of a collection of flattened , membrane-enclosed compartments called cisternae The Golgi Apparatus Consists of an Ordered Series of Compartments Each Golgi Stack consists of 4-6 cisternae, connected through tubular connections The Golgi Apparatus Consists of an Ordered Series of Compartments Remember that the Golgi apparatus connects to cytoskeleton and it is placed closer to the nucleus The Golgi Apparatus Consists of an Ordered Series of Compartments Closer to the nucleus Closer to the plasma membrane The Golgi Apparatus Consists of an Ordered Series of Compartments The Golgi Apparatus Consists of an Ordered Series of Compartments The Golgi Apparatus Consists of an Ordered Series of Compartments Resident proteins in the ER are not in the Lumen but they are exclusively in the membrane Two mechanisms explaining the organization of the Golgi apparatus and how proteins move through it Two mechanisms explaining the organization of the Golgi apparatus and how proteins move through it In the vesicle transport mechanism, Golgi cisternae are static compartments, which contain a characteristic complement of resident enzymes. The passing of molecules from cis to trans through the Golgi is accomplished by forward-moving transport vesicles, which bud from one cisterna and fuse with the next in a cis-to-trans direction Two mechanisms explaining the organization of the Golgi apparatus and how proteins move through it In the cisternal maturation mechanism, each Golgi cisterna matures as it migrates outward through the stack. At each stage, the Golgi resident proteins that are carried forward in a maturing cisterna are moved backwards (blue arrows) to an earlier compartment in COPI-coated vesicles. When a newly formed cisterna moves to a medial position, for example, “leftover” cis Golgi enzymes would be extracted and transported retrogradely to a new cis cisterna behind. Likewise, the medial enzymes would be received by retrograde transport from the cisternae just ahead. In this way, a cis cisterna would mature to a medial and then trans cisterna as it moves outward. transport of cargo molecules through the Golgi apparatus in the forward direction (red arrows) involves elements of both mechanisms!!! WHAT HAPPENS AT THE GOLGI? 1. Protein maturation (mostly glycosylation/MAYBE some cutting by proteases) 2. Sorting to the right next compartment TRANSPORT FROM THE ENDOPLASMIC RETICULUM THROUGH THE GOLGI APPARATUS Oligosaccharide Chains Are Processed in the Golgi Apparatus Corfield, A. Eukaryotic protein glycosylation: a primer for histochemists and cell biologists. Histochem Cell Biol 147, 119–147 (2017). https://doi.org/10.1007/s00418-016-1526-4 MEMBRANE PROTEINS and SECRETED PROTEINS usually contain a complex set of sugar attached to them that is essential for their function MEMBRANE PROTEINS and SECRETED PROTEINS usually contain a complex set of sugar attached to them that is essential for their function Image courtesy of Lorenzo Casalino, Zied Gaieb and Rommie Amaro/ UC San Diego These sugars are added first at the ER and then they are modified in multiple forms as they pass through the Golgi These modifications include adding/removing/substituting the original sugars added at the ER and adding extra elsewhere in the protein These modifications are carried by Golgi resident proteins at different parts of the Golgi These modifications can be so complex that the term Glyco-code has been coined Protein maturation in the ER: (not in order) 1. Removal of ER signal if necessary 2. Processing of protein in smaller pieces if necessary 3. N-Linked glycosylation (asparagine) 4. Disulfide bond formation 5. Proper Folding by Chaperons 6. Subunit assembly Protein maturation in the Golgi: (not in order) 1. Modification of N-linked glycosylation placed in ER 2. More N-linked Glycosylation 3. O-Linked Glycosylation (TSY) 4. Some proteolysis may occur too TRANSPORT FROM THE ENDOPLASMIC RETICULUM THROUGH THE GOLGI APPARATUS The Final step that happens in the Golgi is the sorting of each vesicle into the right compartment The three best-understood pathways of protein sorting in the trans Golgi network Proteins with the mannose 6-phosphate (M6P) marker are diverted to lysosomes (via endosomes) in clathrin-coated transport vesicles. A constitutive secretory pathway delivers proteins with no special features to the cell surface It is always on Proteins with signals directing them to secretory vesicles are concentrated in such vesicles as part of a regulated secretory pathway that is present only in specialized secretory cells In the signal-mediated secretory pathways vesicles store the contents until a signal triggers its release. The vesicles in the signal mediated pathway are called secretory vesicles TRANSPORT FROM THE TRANS GOLGI NETWORK TO THE CELL EXTERIOR AND ENDOSOMES A Mannose 6-Phosphate Receptor Sorts Lysosomal Hydrolases in the Trans Golgi Network A Mannose 6-Phosphate Receptor Sorts Lysosomal Hydrolases in the Trans Golgi Network Lysosomal Hydrolases are enzymes whose final destination is the lysosome They enter the ER in the regular way, pass through the Golgi where are M6P is added The MP6 is recognized by receptors that will concentrate them in lysosome bound vesicles (via the endosome) The transport of newly synthesized lysosomal hydrolases to endosomes The sequential action of two enzymes in the cis and trans Golgi network adds mannose 6- phosphate (M6P) groups to the precursors of lysosomal enzymes The transport of newly synthesized lysosomal hydrolases to endosomes The M6P-tagged hydrolases then segregate from all other types of proteins in the TGN because adaptor proteins (not shown) in the clathrin coat bind the M6P receptors, which, in turn, bind the M6P-modified lysosomal hydrolases The transport of newly synthesized lysosomal hydrolases to endosomes The clathrin-coated vesicles bud off from the TGN, shed their coat, and fuse with early endosomes The transport of newly synthesized lysosomal hydrolases to endosomes At the lower pH of the endosome, the hydrolases dissociate from the M6P receptors, and the empty receptors are retrieved in retromer-coated vesicles to the TGN for further rounds of transport TRANSPORT FROM THE TRANS GOLGI NETWORK TO THE CELL EXTERIOR AND ENDOSOMES Secretory Vesicles Bud from the Trans Golgi Network Secretory vesicles bud from the Trans Golgi Network and they concentrate into mature secretory pathways Exocytosis of secretory vesicles requires a signal (Example: Ca2+, action potential…) Secretory vesicles are quite important in some specialized cells like neurons Petzoldt, A.G. Presynaptic Precursor Vesicles—Cargo, Biogenesis, and Kinesin-Based Transport across Species. Cells 2023, 12, 2248. https://doi.org/10.3390/cells12182248 TRANSPORT INTO THE CELL FROM THE PLASMA MEMBRANE: ENDOCYTOSIS LET”S DO THE OPPOSITE TRIP: from the outside to the inside What do cells uptake from the outside? Solutes / macromolecules / particulate substances Fluids Receptor-ligand complexes Nutrients Extracellular matrix components Cell debris Bacteria Viruses Even other cells in some cases TRANSPORT INTO THE CELL FROM THE PLASMA MEMBRANE: ENDOCYTOSIS LET”S DO THE OPPOSITE TRIP: from the outside to the inside Why do cells need to take up material from the outside ? To recycle proteins and lipids from the membrane and ECM To eliminate damaged proteins or lipids To get nutrients To renew fluids inside As part of a cell signaling pathway As protection against bacteria and viruses To eliminate dead cells To respond to changes in the environment TRANSPORT INTO THE CELL FROM THE PLASMA MEMBRANE: ENDOCYTOSIS LET”S DO THE OPPOSITE TRIP: from the outside to the inside ENDOCYTOSIS is accomplished through the formation of endocytic vesicles that uptake material from the upside All cells are constantly creating endocytic vesicles through a process called pinocytosis (”cell drinking”) Some cells can incorporate large molecules or even bacteria or entire cells in a process called phagocytosis (“cell eating”) THE ENDOCYTIC PATHWAY THE ENDOCYTIC PATHWAY Endocytic vesicles form in the inside, pinching parts of the outside and membrane. They are mostly clathrin-coated but there are exceptions THE ENDOCYTIC PATHWAY Near the cell periphery, endocytic vesicles fuse with an early endosome, which is the primary sorting station THE ENDOCYTIC PATHWAY Tubular portions of the early endosome bud off vesicles that recycle endocytosed cargo back to the plasma membrane—either directly or indirectly via recycling endosomes. Recycling endosomes can store proteins until they are needed THE ENDOCYTIC PATHWAY Maturation from early endosome to late endosome occurs as it is transported through microtubule cystoskeleton placing the late endosome near the nucleus THE ENDOCYTIC PATHWAY As maturation occurs tubular projections are lost, and membrane proteins destined for degradation are internalized in intralumenal vesicles. At this point the endosome is now a multivesicular body THE ENDOCYTIC PATHWAY ph As the Early endosome is transformed into the Late endosome the ph inside the endosome keeps getting more acidic THE ENDOCYTIC PATHWAY Fully matured late endosomes no longer send vesicles to the plasma membrane, and they fuse with one another and with endolysosomes and lysosomes to degrade their contents THE ENDOCYTIC PATHWAY Endolysosome is the product of a lysosome fusing to a late endosome before fully converting into a lysosome THE ENDOCYTIC PATHWAY Each stage of endosome maturation is connected to the TGN via transport vesicles, providing a continual supply of newly synthesized lysosomal proteins. THE ENDOCYTIC PATHWAY The entry into the endocytic pathway can be mediated by a receptor TRANSPORT INTO THE CELL FROM THE PLASMA MEMBRANE: ENDOCYTOSIS Early Endosomes Mature into Late Endosomes ESCRT Protein Complexes Mediate the Formation of Intralumenal Vesicles in Multivesicular Bodies CHOLESTEROL TRANSPORT INSIDE CELLS: an example Receptor-Mediated Endocytosis Most cholesterol is transported in the blood as cholesterol esters in the form of lipid-protein particles known as Low Density Lipoproteins (LDL) LDL contains a core of about 1500 cholesterol molecules esterified to long-chain fatty acids and smaller amounts of free cholesterol and triacylglycerol molecules CHOLESTEROL TRANSPORT INSIDE CELLS: an example Receptor-Mediated Endocytosis A lipid monolayer composed of about 800 phospholipid and 500 unesterified cholesterol molecules surrounds the core of cholesterol esters. A single molecule of apolipoprotein B, a 500,000- dalton beltlike protein, organizes the particle and mediates the specific binding of LDL to cell-surface LDL receptors. The receptor-mediated endocytosis of LDL LDL-receptors at the plasma membrane recognize ApolipoproteinB and mediate formation of Clathrin-coated vesicles The receptor-mediated endocytosis of LDL Shortly After, the Clathrin coat is lost and the vesicle fuses to other vesicles to form the early endosome The receptor-mediated endocytosis of LDL As the acidity increases, the LDL particle dissociates from the LDL receptor, which is recycled back to the plasma membrane while the LDL is retained in the lumen The receptor-mediated endocytosis of LDL As endosome matures, the LDL will end up be inside the endolysosome and lysosomes that contain hydrolytic enzymes that will free cholesterol to be used by the cell for membrane production A COUPLE OF DETAILS Recycling endosomes can serve as an intracellular storage site for specialized plasma membrane proteins that can be mobilized when needed ESCRT Protein Complexes Mediate the Formation of Intralumenal Vesicles in Multivesicular Bodies THE ENDOCYTIC PATHWAY Recycling endosomes can serve as an intracellular storage site for specialized plasma membrane proteins that can be mobilized when needed EXAMPLE: insulin binding to the insulin receptor triggers an intracellular signaling pathway that causes the rapid insertion of glucose transporters into the plasma membrane of a fat or muscle cell, greatly increasing its glucose intake THE ENDOCYTIC PATHWAY Formation of Intralumenal Vesicles is mediated through ESCRT proteins Through a similar process, ESCRT proteins can also mediate cytokinesis and virus exit THE DEGRADATION AND RECYCLING OF MACROMOLECULES IN LYSOSOMES Lysosomes Are the Principal Sites of Intracellular Digestion LYSOSOMES Terminal destination for degradation of proteins, microorganisms, dead cells and other materials ingested through endocytosis or phagocytosis It can receive the material to be degraded directly from the cytosol or the late endosome through the endocytic pathway Remember that in the case of proteins, degradation can also occur through the more targeted polyubiquitination pathway Lysosomes are also the last destination of materials during autophagy LYSOSOMES The action of lysosomes is so destructive because of two reasons: 1. Very low Ph in the lumen caused by a H+ pump 2. The presence of more than 40 enzymes that hydrolyze all kinds of materials (Do you remember how they got there?) (What would happen if one of these enzymes makes it to the cytosol?) LYSOSOMES The action of lysosomes is so destructive because of two reasons: 1. Very low Ph in the lumen caused by a H+ pump 2. The presence of more than 40 enzymes that hydrolyze all kinds of materials The membrane is also special and contains proteins that are highly glycosylated to protect them from acidic environment inside LYSOSOMES The action of lysosomes is so destructive because of two reasons: 1. Very low Ph in the lumen caused by a H+ pump 2. The presence of more than 40 enzymes that hydrolyze all kinds of materials The membrane is also special and contains proteins that are highly glycosylated to protect them from acidic environment inside Once hydrolysis is done, transport proteins at the membrane of lysosomes transport the digested materials into the cytosol so the cell can recycle them Multiple Pathways Deliver Materials to Lysosomes Multiple Pathways Deliver Materials to Lysosomes 1. Straight from Cytosol (not shown) Multiple Pathways Deliver Materials to Lysosomes 2. Using ER-Golgi pathway (not shown). Mostly used to deliver the hydrolytic enzymes present in the lysosomes Multiple Pathways Deliver Materials to Lysosomes 3. Endocytic pathway discussed so far. Remember that it can me selective (receptor-mediated) or non-selective Multiple Pathways Deliver Materials to Lysosomes 4. Macropinocitosis: very large pinocytosis, non-selective uptake of outside materials. It is only used in specific situations for instance, cancerous cells stimulate macropinocitosis to acquire nutrients and materials required to sustain its rapid growth Multiple Pathways Deliver Materials to Lysosomes 5. Phagocytosis. The uptake or large molecules or even entire cells Phagocytosis Incorporation of large structures or entire cells for degradation in the lysosome Amoebas and other organisms use this mechanism to “feed” Humans do not use this mechanism to feed, we rather break down molecules in smaller pieces that get uptaken in the gut and distributed to all cells We do have, however, specialized cells like macrophages or neutrophils that can eliminate bacteria, viruses, and even death cells or dying cells (senescence cells) For instance: macrophages engulf and digest 1011 senescent red blood cells everyday!!!! Antibodies are an important mechanism to initiate phagocytosis They get recognized by Fc receptors at the membrane of macrophages and initiate an actin-mediated phagocytosis Multiple Pathways Deliver Materials to Lysosomes 6. Autophagy. “Self eating” is when a portion of the cytoplasm is engulfed and its contents are sent to the lysosomes Why would cells activate AUTOPHAGY? - During starvation, the lack of nutrients from the outside may force the cell to engulf and digest part of its cytoplasm to supply the cell with the materials to survive. In this case, autophagy is regulated by mTOR a master kinase whose function is regulated by the level of nutrients available. - When an organelle like a mitochondria is not working or it is damaged, autophagy can do the work for that (you can’t use endocytic pathway because the organelle is inside already) - During development or in changing conditions when certain structures or components are no longer needed - Presence of bacteria or viruses inside cells - Presence of macroaggregates that pose a danger for cells - Others… PLANT VACUOLES ARE LYSOSOMES Plant and Fungal Vacuoles Are Remarkably Versatile Lysosomes They are fluid-filled and can occupy 30%-90% of the cell surface They play important roles in turgor pressure to counteract osmosis pressure PLANT VACUOLES ARE LYSOSOMES In many plants it also play very important storage fucntions Examples: Nutrients to be broken down aduring germination Flavor or garlic Opium Pigments that give rise to flower colors Toxins for self defense Many more…. Art Slides This concludes the Slide Set for Chapter 13 Molecular Biology of the Cell, Seventh Edition Copyright © 2022 W. W. Norton & Company, Inc.