Optical Mineralogy Lecture (1-5) PDF
Document Details
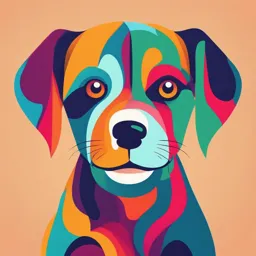
Uploaded by EnergyEfficientHeliotrope4485
Al al-Bayt University
Sanaa Al-Zyoud
Tags
Summary
These lecture notes cover the basic concepts of optical mineralogy, including light properties, interactions with minerals, and various techniques like polarized light microscopy. The lectures discuss different types of light interactions, wave-particle duality, and optical properties of minerals through examples such as birefringence, pleochroism, and relief.
Full Transcript
Optical Mineralogy (801202) First Semester (2024/2025) Dr. Sanaa Al-Zyoud Applied Earth and Environmental Sciences Department Earth and Environmental Sciences Faculty Al al-Bayt University Text Book Course Content Course Content Course Content...
Optical Mineralogy (801202) First Semester (2024/2025) Dr. Sanaa Al-Zyoud Applied Earth and Environmental Sciences Department Earth and Environmental Sciences Faculty Al al-Bayt University Text Book Course Content Course Content Course Content Course Content Light as the Tool of Examination in Optical Mineralogy Light interacts with the atomic structure of minerals, which helps in identifying minerals in rocks using a petrographic microscope What is LIGHT? It is a form of energy, detectable with the eye, which can be Light is a small part of the transmitted from one place to electromagnetic spectrum, with another at finite velocity. visible wavelengths ranging from Visible light is a small portion of a 390 nm to 770 nm continuous spectrum of radiation ranging from cosmic rays to radio waves. Light spectrum The principle of optical mineralogy relies on how light behaves as it passes through or reflects off a mineral How the light transfer in microscope? Light and Electromagnetic Radiation Radio Waves: Small portion Long of the wavelengths and electromagneti low energy c spectrum X-rays and with Gamma Rays: wavelengths of Very short 390 nm (violet) wavelengths and to 770 nm high energy (red) Light is a form of electromagnetic radiation, part of the electromagnetic spectrum that includes various other forms of radiation such as Light and The visible spectrum for the human eye is Electromagnetic only a small portion of this range Radiation The behavior of light when interacting with minerals allows for optical examination of their properties QED combines principles from Maxwell's electrodynamics and Schrödinger's quantum mechanics, describing light as being composed of photons Light exhibits wave-particle duality Quantum Wave nature explains phenomena like refraction and interference Electrodynamics Particle nature explains energy interactions at the atomic level, such as electron excitation and Light This theory is fundamental to modern physics and helps explain how light interacts with mineral structures Quantum Electrodynamics and Light Particle Theory: Light is composed of photons that can excite electrons, which affects optical properties such as color Particle and Wave Theory: Light behaves as a Wave wave, explaining how it refracts, reflects, polarizes, or interferes Theory of when interacting with minerals Light Both particle and wave theories are used in optical mineralogy depending on the phenomena being observed Electromagnetic radiation and Light Waves The electromagnetic radiation theory of light implies that light consists of electric and magnetic components which vibrate at right angles to the direction of propagation. In optical mineralogy only the electric component, referred to as the electric vector, is considered and is referred to as the vibration direction of the light ray. The vibration direction of the electric vector is perpendicular to the direction in which the light is propagating. The behavior of light within minerals results from the interaction of the electric vector of the light ray with the electric character of the mineral, which is a reflection of the atoms and the chemical bonds within that minerals. Light waves are described in terms of velocity, frequency and wavelength. Electromagnetic radiation and Light Waves The velocity (V) and the wavelength are related in the following equation, where: F = Frequency or number of wave crests per second which pass a reference points => cycles/second of Hertz (Hz). For the purposes of optical mineralogy, F = constant, regardless of the material through which the light travels. If velocity changes, then the wavelength must change to maintain constant F. Light does not consist of a single wave => infinite number of waves which travel together WAVE FRONT, WAVE NORMAL With an infinite number of waves travelling together from a light source, we now define: 1.Wave front - parallel surface connecting similar or equivalent points on adjacent waves. 2.Wave Normal - a line perpendicular to the wave front, representing the direction the 3.wave is moving. 4.Light Ray is the direction of propagation of the light energy. WAVE FRONT, WAVE NORMAL Electric Vector: Vibrates at a right angle to the magnetic vector Light is composed of Magnetic Vector: two main components Perpendicular to both the electric vector and the direction of propagation Components As light propagates, it interacts with the lattice of a crystal, and its of Light and direction and speed can change depending on the mineral structure Propagation This change in light behavior is essential for understanding refractive index and other optical properties of minerals Light components Light is described as having two components, an electric and a magnetic vector vibrating at a right angle to each other and traveling at a right angle to these two vectors along the direction of propagation Isotropic and Anisotropic Minerals Isotropic Minerals Isotropic materials show the same velocity of light in all directions because the chemical bonds holding the minerals together are the same in all directions, so light travels at the same velocity in all directions. Examples of isotropic material are volcanic glass and isometric minerals (cubic) Fluorite, Garnet, Halite In isotropic materials the Wave Normal and Light Ray are parallel. Anisotropic Minerals Anisotropic minerals have a different velocity for light, depending on the direction the light is travelling through the mineral. The chemical bonds holding the mineral together will differ depending on the direction the light ray travels through the mineral. Anisotropic minerals belong to tetragonal, hexagonal, orthorhombic, monoclinic and triclinic systems. Refractive Index, Dispersion,Polarization, and Double Refraction ** The Snell-Descartes law 2. The velocity c of light in vacuum or V vacuum is 299 792 km/s which means that you could travel around the world 7.5 times in a second. 2. The velocity of light is reduced in denser media. Velocity in a medium is expressed as the refractive index (n). 3. (n) is defined as the ratio of the velocity of light in a vacuum to that in a medium and varies with temperature and wavelength. Refractive Index For example, the refractive index of pure water is on average 1.333 and would indicate that the light in the vacuum travels 1.333 times faster than in water. The velocity of light in water is calculated at 224 900 km/s and is therefore slower than for light in the vacuum. Refractive index As a light ray passes across the boundary separating two media, in our case air and a mineral, the path of the light ray is bent. The path of the light follows the law of Snell-Descartes. where n1 and nr are the refractive indices of the two media, and θi and θr are the angles of incidence and refraction between the normal to the surface between the two media Refractive index Polarization of Light Understanding Light Behavior in Optical Mineralogy Introduction to Polarization of Light Polarization Basics: Polarization refers to the restriction of light's vibration to a single plane Why is Polarization Important in Mineralogy? Polarized Light Microscopy: This form of microscopy uses polarized light to study thin sections of minerals and rocks, revealing key optical properties such as birefringence, pleochroism, and refractive index Ordinary Light and Its Properties Ordinary Light Characteristics: Ordinary light, such as sunlight or artificial light, vibrates in all directions perpendicular to its path of travel Challenges in Mineral Analysis: Unpolarized light makes it difficult to study how light interacts with mineral structures, as it vibrates in multiple planes Electromagnetic Nature of Light: Light consists of oscillating electric and magnetic fields that propagate through space as waves Types of polarization Three types of polarization are possible. Plane Polarization Circular Polarization Elliptical Polarization Plane Polarized Light Plane Polarized Light Definition: Plane polarized light consists of light waves that vibrate only in one specific plane Polarized Light and Crystals: Polarized light changes direction and velocity when it interacts with a crystal, revealing details about the crystal’s structure Electromagnetic Sine Wave: A plane polarized light wave can be visualized as an oscillating electric field that moves in a consistent direction Generating Polarized Light Polarization Methods: Polarized light can be generated through several techniques Selective Absorption: Light is absorbed based on its orientation relative to a polarizing filter Reflection and Refraction: Polarization occurs when light reflects off surfaces like water or glass Scattering: Light scatters in the atmosphere, creating polarization, such as in the blue sky Laboratory Techniques: In mineralogy, polarized light is usually generated by passing light through specialized filters, such as polaroid sheets or Nicol prisms Polarization by Selective Absorption Selective Absorption in Polarization: A polarizing filter, often made from polyvinyl alcohol , absorbs light waves vibrating parallel to the filter’s molecular structure Role of Polymer Chains: The polymer chains in the filter are aligned to selectively absorb certain light vibrations while allowing perpendicular vibrations to pass through Iodine Dopant: Iodine is used to enhance the absorption properties of the polarizing filter, further restricting unwanted light vibrations Polarization in Crystals and Color Effects Double Refraction in Crystals: When polarized light enters a crystal, it often splits into two rays – an ordinary and an extraordinary ray Pleochroism in Minerals: Certain minerals exhibit pleochroism, where they display different colors when viewed under polarized light from different angles Importance in Mineral Identification: These color changes are important for identifying minerals and understanding their properties Polarization by Reflection Light Reflection on Surfaces: When light strikes a reflective surface at an angle, some of it is refracted , while a portion is reflected Polarization by Reflection: The reflected light is partially or fully polarized, depending on the angle of incidence Brewster’s Angle: When light strikes a surface at Brewster’s angle, the reflected light becomes fully polarized, and the refracted ray continues into the medium Brewster’s Angle and Its Calculation Brewster’s Angle Explained: Brewster’s angle is the angle of incidence at which light reflected from a surface becomes completely polarized Snell’s Law: Brewster’s angle can be calculated using Snell’s Law, based on the refractive indices of the two media Practical Applications: Polarization by reflection is used in photography to reduce glare and in optical instruments for enhancing visibility in certain conditions Crossed Polarizers in Microscopy Using Crossed Polarizers: When two polarizers are placed at 90-degree angles to each other in a microscope, they block all light from passing through unless a mineral sample introduces birefringence XPL and PPL in Mineralogy: Crossed Polarizers reveal different mineral properties compared to Plane Polarized Light , helping scientists identify minerals more accurately Applications in Analysis: Crossed polarizers are used to observe birefringence, pleochroism, and extinction angles in minerals Polarization by Scattering Scattering of Light in the Atmosphere: Polarization occurs naturally when light scatters off small particles in the atmosphere, such as air molecules Applications in Astronomy: Polarization by scattering is used to study planetary atmospheres and detect exoplanets Astronomical Observations: Polarized light observations help astronomers understand the composition and behavior of distant celestial bodies Summary of Polarization in Mineralogy Review of Polarization: Polarized light plays a critical role in optical mineralogy, allowing scientists to study the internal structure and optical properties of minerals Different Polarization Methods: Methods like selective absorption, reflection, scattering, and double refraction help generate polarized light Applications in Science: Polarization is widely used in fields ranging from geology to astronomy, providing insights into material composition and behavior Lecture (4) Double refraction in anisotropic minerals: example calcite In anisotropic minerals light does not propagate at the same velocity in all directions. Light entering a crystal is split into two rays that take different paths during their journey through the crystal and emerge from the crystal as separate light rays. These rays vibrate at right angles, have different velocities giving different refraction indices for the mineral and may have different absorption behavior. This phenomenon is called double refraction. Ordinary and Extraordinary rays 1. The wave front is perpendicular to the direction of propagation and the ray travels through the crystal and emerges at the other side. This ray is called the ordinary ray. 2. the wave front of the refracted light rays spreads out as ellipsoids and the so-called optic axis is the major axis of the ellipsoid. The propagation direction is not parallel to the wave front. This ray is called the extraordinary ray. Optic axis Along the c-axis light is not refracted or polarized and the direction is called the optic axis of the crystal. In calcite, it represents the crystallographic c-axis of threefold rotation Retardation of Rays in Anisotropic Minerals and Birefringence In a crystal the slow ray lags behind the fast ray and their difference on emergence is called the retardation Retardation 1.In Phase 2.Out of Phase 3.In/Out of phase Retardation When this plane polarized light passes through an anisotropic medium, in this case a uniaxial mineral, the light is doubly refracted or split into two waves, the slow and the fast ray or the ordinary and extraordinary rays, vibrating at 90° and traveling with two different velocities. This means that the crystal lattice and the chemical composition of a mineral govern the double refraction and the difference in velocity and refractive indices and are highly characteristic for a mineral. Retardation When the two rays exit the mineral above the thin section plane, the fast ray will be ahead of the slow ray and the slow ray will lag behind the fast ray by a certain distance called retardation Retardation The magnitude of this retardation depends on the thickness d of the mineral and the values of the refractive indices for the two vibration directions can be calculated. The slow ray needs more time to travel through the crystal. The time trslow (time of the slow ray) can be calculated as Retardation Vrslow is the velocity of the slow ray, and d is the distance (thickness) traveled. During the time trslow, the fast ray not only raveled through the crystal, but already covered an additional distance outside the crystal called the retardation C. Vrfast is the velocity of the fast ray and V the velocity of light in air (for practical purposes, the same as in a vacuum). Retardation By combining and rearranging the equations, we get Retardation The retardation C is the distance the slow ray has lagged behind the fast ray on emergence and depends on the thickness of the mineral the light travels through. It is given in nm or wavelength k depending on the context. Since thin sections are normally at a thickness of 30 lm, we can omit the thickness d and just use the difference between the indices of refraction of the slow and the fast ray. which is called the birefringence. The birefringence is an important optical characteristic. Lecture (5) Minerals properties in PPL Optical mineralogy involves studying rocks and minerals by studying their optical properties. Some of these properties are macroscopic and we can see them in mineral hand specimens. But generally we use a petrographic microscope, also called a polarizing microscope and the technique is called transmitted light microscopy or polarized light microscopy (PLM). For more info: https://www.youtube.com/watch?v=ahS5KlXqQXc Minerals properties in PPL A fundamental principle of PLM is that most minerals – even dark- colored minerals and others that appear opaque in hand specimens – transmit light if they are thin enough. In standard petrographic microscopes, polarized light from a source beneath the microscope stage passes through samples on the stage and then to your eye(s). Minerals properties in PPL Most optical mineralogy today involves specially prepared thin sections (0.03-mm-thick specimens of minerals or rocks mounted on glass slides). How to make thin sections? 1. Cutting the Rock Sample 1. Polishing the Rock Slice 1. Mounting on a Glass Slide 1. Grinding the Slice to thinness 1. Final Polishing 2. Viewing Under Microscope For more info: https://www.youtube.com/watch?v=8Op9jSgdmfA Minerals thin sections Whether looking at grain mounts or thin sections, transmitted light microscopy allows us to determine and measure properties that are otherwise not discernible. We can identify minerals, sometimes their compositions, and we can observe mineral relationships that allow us to learn about mineral origins. Minerals Color Minerals with metallic luster and a few others are termed opaque minerals. They will not transmit light even if they are thin-section thickness. So they always appear black when viewed with a microscope. Magnetite is an opaque mineral. For studying opaque minerals, transmitted light microscopy is of little use. Reflected light microscopy (RLM), a related technique, can reveal some of the same properties. As the name implies, when using RLM, the light source is above the sample and light reflects from the sample to our eye. RLM, although an important technique for economic geologists who deal with metallic ores, is not used by most mineralogists or petrologists. Minerals Color Some minerals that are darkly colored in hand sample will also appear to have color in thin section, even though the rock slices in thin sections are typically only 30 micrometers thick. Sometimes the color of a mineral in plane polarized light can aid in identification because it is characteristic for a specific mineral. But, be cautious about relying too heavily on color as an identification tool! Minerals within a solid solution group can have very different color characteristics in hand sample and under the microscope. Minerals Color Pleochroism is defined as the color variation observed in anisotropic materials under a microscope when the specimen is rotated, resulting from destructive interference with the polarization direction of light. With the upper polar removed, many colored anisotropic minerals display a change in color - this is pleochroism or diachroism. Produced because the two rays of light are absorbed differently as they pass through the colored mineral and therefore the mineral displays different colors. Pleochroism is not related to the interference colors For real video: https://youtu.be/1UZslfztDCE Pleochroism The selective absorption in crystals of light vibrating in different planes. Pleochroism is the general term for both dichroism, which is found in uniaxial crystals (crystals with a single optic axis), and trichroism, found in biaxial crystals (two optic axes). It can be observed only in colored, doubly refracting crystals. When ordinary light is incident on a crystal exhibiting double refraction, the light is split into two polarized components, an ordinary ray and an extraordinary ray, vibrating in mutually perpendicular planes. A dichroic substance such as tourmaline transmits only the extraordinary ray, having absorbed the ordinary ray. Minerals Color Relief Refractometry involves the determination of the refractive index of minerals, using the immersion method. This method relys on having immersion oils of known refractive index and comparing the unknown mineral to the oil. If the indices of refraction on the oil and mineral are the same light passes through the oil-mineral boundary un-refracted and the mineral grains do not appear to stand out. Relief If noil nmineral then the light travelling though the oil-mineral boundary is refracted and the mineral grain appears to stand out. Relief RELIEF - the degree to which a mineral grain or grains appear to stand out from the mounting material, whether it is an immersion oil, Canada balsam or another mineral. Relief 1. Strong relief mineral stands out strongly from the mounting medium, whether the medium is oil, in grain mounts, or other minerals in thin section, for strong relief the indices of the mineral and surrounding medium differ by greater than 0.12 RI units. Relief 2. Moderate relief mineral does not strongly stand out, but is still visible, indices differ by 0.04 to 0.12 RI units. Relief 3. Low relief mineral does not stand out from the mounting medium, indices differ by or are within 0.04 RI units of each other. Relief A mineral may exhibit positive or negative relief: +ve relief - index of refraction for the material is greater than the index of the oil. - e.g. garnet 1.76 -ve relief nmin < noil - e.g. fluorite 1.433 It is useful to know whether the index of the mineral is higher or lower that the oil. This will be covered in the second lab section - Becke Line and Refractive Index Determination. Lecture (6) BECKE LINE