Advanced Therapeutic Technology Lecture 5 & 6 PDF
Document Details
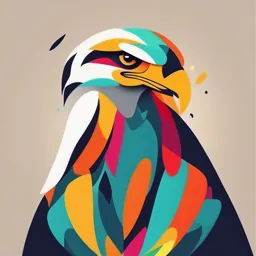
Uploaded by BeneficiaryEuphoria1755
2024
Tags
Summary
This lecture details contrasting myocardial aging, covering life expectancy and the advancements in medical and technological innovations. It discusses competing risks of disease and the study of aging in mice. The lecture considers heart failure as a prominent disease in aging populations and the role of medications like Sacubitril/Valsartan and Dapagliflozin.
Full Transcript
ADVANCED THERAPEUTIC TECHNOLOGY Lecture 5 and 6 (19-21.11.2024) CONTRASTING MYOCARDIAL AGING: FROM MICE TO MEN Life expectancy of the world population is around 73.3 years old. In the graph, there’s the representatio...
ADVANCED THERAPEUTIC TECHNOLOGY Lecture 5 and 6 (19-21.11.2024) CONTRASTING MYOCARDIAL AGING: FROM MICE TO MEN Life expectancy of the world population is around 73.3 years old. In the graph, there’s the representation of death distribution, considering on the x axis the age and on the y axis the number of deaths. Comparing the 2016 data with the 1900 ones, the curve has moved causing the FIRST LONGEVITY REVOLUTION. This occurred thanks to medical and technological innovations, which together increased the healthspan and changed the age-target of geroscience. Indeed, as a consequence of innovation, COMPETING RISKS have arised: when the risk of death from a disease decreases, the risk of death from other diseases increases. In the past, for example, the main cause of death was related to infectious diseases, but once fought them, other diseases have shown up, like diabetes, hypertension, or cancer. The maximum lifespan of human beings has been elongated through time, but the probability of any substantial increase in maximum lifespan in this century is remote. The improvement in the life expectancy is generally decelerating: future increases require improvements in mortality at older ages. Indeed, the goal of life extension has been achieved, so the principal outcome and most important metric of success should be the extension of healthspan. Life expectancy is around 73.3 years, whereas healthspan is around 64 years: there is a gap of 9.2 years, which can be “filled” around 2030 thanks to the generation of some research program. HEART FAILURE is one of the predominant diseases in aging populations and in Italy is one of the leading causes of hospitalization and death. A major risk factor for heart failure and overall cardiovascular disease is age. Multiple processes contribute to cardiac dysfunction, with the development of Fibrosis, inflammatory response, diastolic disfunction, mitochondrial disfunction, increased apoptosis and loss of regenerative capacity. FISCHER (CDF) RATS are the main aged rodent colonies, used to study aging. A specific mouse subgroup (C57BL/6 +HFD +DOCP) is common among the aging, hypertension and cardiometabolic mouse models. CDF rats were treated with drug used for Heart failure, like Sacubitril or Valsartan, which block in humans the neurohormonal pathway involved in the development of HF, but they were approved only for HF in patients with reduced ejection fraction (and not for preserved ejection fraction). Preservation of performance in all experimental groups, as demonstrated by EF and FS values and unexpected inefficacy of drug treatments (SACUBITRIL/VALSARTAN AND VALSARTAN) to improve diastolic function was confirmed by hemodynamic and echocardiographic analysis. In this experimental set, mice were sacrificed at 3 months, but then three main changes have been performed: 1. The treatment was anticipated from 18 months old to 15 months old. 2. The treatment was extended from 3 to 10 months (extension of treatment duration). 1 3. The drugs were combined with Dapagliflozin, one of the last drugs discovered for HF treatment. According to this set, three populations of old mice are present: some of them are vehicles, some are treated with Sacubitril and Valsartan and others with Sacubitril, Valsartan and Dapagliflozin. Dapagliflozin was at first approved as a diabetic drug, but then it was showed its positive effect in cardiovascular diseases. Indeed, some years before, Rosiglitazone was approved as a diabetic drug, but then it was found to be cardiotoxic. This determined the presence of controls, during clinical trials, for cardiovascular risk, whenever a diabetic drug has to be approved. When this analysis was performed for Dapagliflozin, not only it was possible to exclude the cardiotoxicity, but also to show a reduced hospitalization for cardiovascular diseases in treated patients. Moreover, this drug results good for the treatment of patients with preserved ejection fraction, for which no effective therapy resulted available. However, it is still not known how the drug works, but several hypotheses have been proposed. One of the target of these new treatments (alone or in combination with Dapagliflozin) is fibrosis, which resulted reduced in treated rats, but also inflammation, which is the major pathological component of heart disfunction progression, was reduced in micro-vessels, extracellular matrix proteins and cardiomyocytes. Considering oxidative stress, these treatments reduced it in two ways, both by decreasing the synthesis of enzymes that participate in the generation of oxidative species, and increasing the scavengers, so the cells able to remove ROS species. Long-term treatment with Sacubitril/Valsartan improves cardiac function, but the addition of Dapagliflozin activates the ANTIOXIDANT DEFENSE and the AMPK/NAMPT/SIRT pathway, leading to further improvement of cardiac function. Moreover, the addition of Dapagliflozin improved heart functions and reduced skeletal muscle weakness. Dapagliflozin activates SIRT1 pathway and in different stress conditions, the drug affects the stress-induced premature senescence pathway. Many studies have evaluated the ability of this drug to reduce endothelial cell senescence in type 2 diabetes through the inhibition of SGLT2, on which Dapagliflozin exerts most of its effects, to alleviate pathological aging. Upon stress, cardiac cells can be repaired to overcome stressful conditions, so they turn back to their normal metabolism, or they can die when the stress is too much to fight, causing heart ischemia, or they become senescent. Senescence cells produce some molecules and form the SENESCENT CELL ASSOCIATED PHENOTYPE, through which they affect the neighbor cells and spread the “sickness.” Silent information regulator 2 (Sir2) family of proteins is a group of NAD+-dependent deacetylases/ADP- ribosyl-transferases (type III histone deacetylase) initially discovered in yeast and are identified as key regulators of health span and lifespan in yeast and other organisms. Mammals have seven (SIRT1–7) known sirtuins, of which SIRT1 is the closest and best-characterized mammalian orthologue of yeast Sir2. SIRT1 deacetylase activity has been involved in: Chromatin remodeling; Gene silencing; DNA damage response. SIRT1 is recruited to chromatin upon different DNA damage insults, where it favors efficient repair of double- strand breaks (DSBs) by homologous recombination. SIRT1 physically interacts and deacetylates the WRN helicase (Werner syndrome ATP-dependent helicase), in this manner modulating homologous recombination– dependent DSB DNA repair. Together, these findings suggest a potential role of SIRT1 in the regulation of DNA repair pathways. In one recent study, it was found that SIRT1 contributes to telomere maintenance and augments global homologous recombination. A potential role of SIRT1 in telomere biology was studying in both loss of function (SIRT1-deficient mice) and gain of function (SIRT1super mice) mouse models. SIRT1 interacts with the mouse 2 telomeric repeats and acts as a positive regulator of telomere length in vivo by significantly attenuating telomere shortening associated with mouse aging. These findings link SIRT1 to telomeres and provide new mechanistic explanations for the known roles of augmented SIRT1 expression in protection from DNA damage and in prevention from some age-associated pathologies. Senescence can be induced by various stressors and physiological states: replicative senescence; senescence involved in physiological processes; premature senescence. REPLICATIVE SENESCENCE is a type of senescence induced by the progressive shortening of telomeres during cell division. Leonard Hayflick discovered that cultured normal human cells have limited capacity to divide, after which they become senescent —a phenomenon now known as the ‘Hayflick limit.’ What was shown in these studies is that SIRT1 interferes with senescence in two ways, so by inhibiting DNA damage and preventing telomere shortening, so cell cannot move in cell cycle block and keeps doubling. Telomeres are repetitive DNA sequences located at the ends of chromosomes and function as essential guardians of genomic stability. Telomeres are specialized nucleoprotein structures that protect the ends of eukaryotic chromosomes from unscheduled DNA repair reactions and degradation. In vertebrates, telomeres consist of TTAGGG repeats bound by a specialized multiprotein complex known as shelterin, which has fundamental roles in the regulation of telomere length and protection. Because of the intrinsic inability of the DNA replication machinery to copy the end of linear molecules, and to endogenous DNA end– degrading activities, telomeres become progressively shorter after every cell division cycle. Telomere repeats are generated by a ribonucleoprotein reverse transcriptase known as telomerase. Telomerase abundance and activity in adult tissues is not sufficient to compensate for the progressive telomere attrition that occurs with aging. CELLULAR SENESCENCE Cellular senescence is a state where cells enter a permanent state of growth arrest. While this might sound detrimental, it actually plays a crucial role in various biological processes, such as wound healing and preventing tumor formation. However, an accumulation of senescent cells can also contribute to age-related diseases and other health problems. This state is triggered through two primary mechanisms: 1. Replicative senescence, which arises when cells reach their natural limit of divisions. Telomeres, the protective caps at the ends of chromosomes, shorten with each cell division and when they become critically short, the cell enters replicative senescence, halting further division. 2. Stress-induced premature senescence, activated by environmental or physiological stressors. Various stresses, such as DNA damage, oxidative stress or exposure to certain chemicals, can trigger premature cellular senescence. This is a protective mechanism to prevent the propagation of damaged cells. 3 While senescent cells no longer replicate, they refuse to die, persisting in tissues, where they can interfere with normal functions and trigger inflammation. Their presence has widespread effects, impacting processes like tissue repair and contributing to the progression of chronic diseases. To study and address senescence, the NIH Cellular Senescence Network (SenNet) Consortium has developed guidelines to detect and classify these cells. Detection progresses through two phases: first, validating the presence of senescence, and second, specifying its subtype. The presence of senescence is confirmed through various markers. One of the most common is SA- ß-Gal (Senescence-Associated ß-Galactosidase), an enzyme that accumulates in the lysosomes of senescent cells and can be detected through staining. Another marker is lipofuscin, a pigmented material that builds up in senescent cells due to impaired degradation processes. Proliferation markers are also used to verify that the cells are no longer actively dividing. Additionally, genes such as p16, p21, lamin B1, and core senescence transcripts are upregulated in senescent cells, reflecting their altered gene expression profile. Finally, senescent cells are known to release a variety of proteins collectively referred to as the senescence-associated secretory phenotype (SASP), which impact the surrounding tissue environment. Phase II involves determining the subtype of senescence present in the cells. This step is crucial because different subtypes of senescence can have varying effects on disease progression and potential treatment strategies. In this phase, researchers focus on markers such as pro-inflammatory SASP transcripts and secreted proteins, as some senescent cells release pro- inflammatory cytokines that can contribute to age-related diseases. Additionally, senescence subtype- specific transcripts are examined, as different senescence subtypes may be distinguished by the expression of particular genes. By identifying these markers, researchers can more precisely detect and characterize senescent cells, providing valuable insights into the role of senescence in aging and its involvement in disease development. In the lab, cells pass through three distinct phases of culture: Phase I, or the primary culture, marks the beginning of cellular life. Phase II is the period of exponential replication, the pinnacle of cellular growth. Phase III signals the onset of senescence, where replication ceases but metabolism continues. Morphological changes in Phase III are striking. Senescent cells, which were previously spindle-shaped and compact, become flattened and irregular in shape. Their nuclei expand in size, and the cytoplasm is filled with granular structures. These alterations serve as clear visual indicators of cellular aging. SA-β-galactosidase (SA-β-gal) is one of the most widely recognized and reliable biomarkers for detecting cellular senescence. Its elevated activity in senescent cells serves as a hallmark of this state, distinguishing senescent cells from their non-senescent counterparts. This increased activity is a result of an upregulation of lysosomal enzymes, which is a characteristic feature of senescent cells. The underlying mechanism involves the GLB1 gene, which is overexpressed in senescent cells. The GLB1 gene encodes the β-galactosidase enzyme, responsible for cleaving terminal β-galactose from glycoconjugates. This functional shift in lysosomal activity is closely linked to the overall metabolic changes associated with cellular aging. 4 To detect SA-β-gal activity, a common and straightforward method employs X-gal (5-bromo-4-chloro-3-indolyl β-D- galactopyranoside) as a chromogenic substrate. When cells or tissues are incubated with X-gal, β-galactosidase cleaves the substrate, producing a blue precipitate. The blue staining serves as a direct, visual indicator of senescence. The intensity and spread of the blue color are proportional to the level of SA-β-gal activity, enabling researchers to identify and quantify senescent cells. This method has proven effective in both in vitro (cell culture) and in vivo (tissue samples) studies, making it an indispensable tool in the field of aging research and senescence biology. While the X-gal assay is relatively simple and inexpensive, it is also highly sensitive, allowing for the detection of even low levels of senescent cells. Furthermore, the ability to visually distinguish senescent cells under a microscope provides qualitative insights into the spatial distribution and morphology of these cells within a sample, complementing other molecular and biochemical assays. Despite its advantages, it is important to note that SA-β-gal activity is not entirely exclusive to senescent cells. It can occasionally be detected in other cell types, such as macrophages, under certain conditions. As such, the use of SA-β-gal is often combined with additional senescence markers—such as p16INK4a, p21WAF1/CIP1, or SASP factors—to ensure accurate identification and characterization of senescent cells. The C12FDG assay is a powerful method for detecting cellular senescence by utilizing a fluorescent probe that acts as a substrate for β-galactosidase. Once inside living cells, C12FDG is cleaved by β- galactosidase, producing fluorescence that correlates with the enzyme's activity. This allows researchers to visualize and quantify senescent cells in real-time, making it ideal for studying senescence dynamics. Using flow cytometry, the fluorescence intensity of individual cells can be analyzed, distinguishing senescent cells as a distinct population based on their higher fluorescence. This provides a quantitative and high-throughput approach to senescence detection. Microscopic images, such as the inset (d), further confirm the presence of senescent cells by showing fluorescence accumulation alongside typical morphological changes, such as cell flattening and enlargement. Together, this method combines precision, live-cell compatibility, and versatility, making it indispensable in senescence research. Lipofuscin, a yellow-brown pigment that accumulates in aging cells, is a byproduct of the cell's recycling process. It forms from the lipid-containing residues left over from lysosomal digestion, which the cell fails to fully degrade. As a result, these indigestible materials accumulate over time, particularly in long-lived or metabolically active cells, such as neurons and muscle cells. Lipofuscin buildup is closely linked to cellular dysfunction. It is often associated with the impairment of mitochondria, lysosomes, and the proteasome, the cellular machinery responsible for energy production, waste processing, and protein turnover. Various stressors, such as oxidative damage and metabolic inefficiencies, exacerbate this accumulation, making lipofuscin not only a marker of aging but also a contributor to cellular decline. As a sensitive biomarker of aging and senescence, lipofuscin serves as a reliable indicator of cellular health. By measuring lipofuscin levels, researchers can assess the extent of cellular aging and identify cells that have entered a state of senescence. Recently, advancements in detection techniques have enhanced the study of lipofuscin. The GL13 reagent, based on biotinylated Sudan Black B, has been developed for precise detection and quantification. This innovative reagent is used in a hybrid histochemical-immunohistochemical method, which combines traditional staining with immunological detection to measure soluble lipofuscin levels in biological fluids. This method offers both high sensitivity and specificity, enabling researchers to track aging processes and study the role of senescent cells in diseases with greater accuracy. By leveraging tools like GL13, scientists can deepen their understanding of cellular aging mechanisms and develop targeted interventions for age-related disorders. 5 A comprehensive approach for identifying senescent cells involves the use of multiple complementary markers, allowing researchers to distinguish these cells from actively dividing ones. This method combines traditional senescence markers, such as SA-β-gal, with proliferation markers like EdU and Ki67, along with other innovative tools like GL13, which detects lipofuscin. Together, these markers provide a reliable framework for accurately identifying and characterizing senescent cells. EdU, a thymidine analogue, incorporates into the DNA of cells undergoing replication and marks active DNA synthesis, while Ki67 is expressed during all active cell cycle phases except the resting phase (G0). The absence of EdU or Ki67 in SA-β-gal-positive cells confirms their non-dividing, senescent state. Visualization through imaging demonstrates these complementary markers: in one case, cells stained for SA-β-gal (green), EdU (red), and DAPI (blue) show mutually exclusive patterns, with SA-β-gal-positive cells lacking EdU incorporation, confirming their senescence. Similarly, staining with Ki67 (red) and GL13 (brown) reveals that lipofuscin-positive cells do not express Ki67, further validating their non-proliferative, senescent nature. This combination of markers provides a robust framework for accurately characterizing senescent cells and their role in aging and disease. Detecting senescent cells is challenging due to the lack of specific and universal biomarkers. While an ideal marker would unambiguously identify senescent cells and assess the effectiveness of senotherapies, current markers like SA-β-gal and p16INK4A are not universally reliable across all cell types. Additionally, false positives, such as M2 macrophages expressing senescence markers like p16INK4A, complicate accurate detection. Overcoming these issues is critical to improving the identification and targeting of senescent cells in aging and disease. Secondary senescence refers to the spread of senescence from one cell to its neighboring cells, amplifying the aging process within tissues. This phenomenon occurs through two main mechanisms: paracrine senescence and juxtacrine senescence. In paracrine senescence, senescent cells secrete a range of pro-inflammatory molecules collectively known as the senescence- associated secretory phenotype (SASP). These factors include cytokines, chemokines, and growth factors that create a local inflammatory environment. SASP factors can induce senescence in nearby healthy cells, effectively propagating the senescence signal and accelerating tissue dysfunction. Juxtacrine senescence, on the other hand, occurs through direct cell-to-cell contact. In this case, senescent cells communicate with their neighbors via specific signaling pathways, such as the NOTCH1-JAG1 pathway that induces senescence in adjacent cells by triggering molecular changes that mimic the conditions of cellular aging. The spread of senescence within tissues can contribute to their degeneration and the progression of age-related diseases. When cells enter senescence, they often develop the senescence-associated secretory phenotype (SASP), which is a mix of factors that have a range of effects, such as promoting inflammation, inducing apoptosis in nearby cells, and contributing to fibrosis. The SASP can create a chronic inflammatory environment that accelerates aging and increases the risk of age-related diseases. Interestingly, senescent cells avoid self- destruction by activating anti-apoptotic pathways, allowing them to persist in tissues. This persistence is problematic because senescent cells not only remain metabolically active, but also continuously secrete factors that worsen tissue damage and dysfunction. The composition of the SASP can vary depending on the cell type and tissue, meaning that the absence of common SASP markers doesn't rule out senescence. Some cells may express a distinct set of SASP factors that are specific to their type or tissue. Therefore, the lack of typical SASP markers should not be considered definitive evidence against senescence. 6 →While cellular senescence can act as a protective mechanism by preventing the proliferation of damaged cells and potentially contributing to tumor suppression, it can also become a driver of chronic diseases. As we age, the number of senescent cells increases, and the immune system becomes less efficient at clearing them. This imbalance leads to the accumulation of senescent cells and the release of SASP factors, which can promote inflammation, tissue damage, and the development of age-related diseases. However, it's important to note that senescent cells also play a role in tissue repair and wound healing. Therefore, targeting senescent cells requires a delicate balance to avoid unintended consequences. The Threshold Theory of Senescent Cell Accumulation explains how the accumulation of senescent cells contributes to chronic diseases and age-related disorders. It suggests that once senescent cells surpass a certain threshold, their accumulation triggers a self-perpetuating cycle, where the SASP spreads senescence to neighboring cells faster than the immune system can clear them. This imbalance worsens the situation over time. Senolytic interventions, which target and remove senescent cells, may help delay or prevent age-related diseases, offering a promising approach for healthier aging. Senotherapy is an emerging field focused on targeting senescent cells and it consists of two main approaches: senolytic and senomorphic therapies. 1. Senolytic therapy aims to eliminate senescent cells through programmed cell death. This approach involves the use of drugs or other agents that specifically target senescent cells for destruction → senolysis. 2. Senomorphic therapy seeks to modulate the SASP, suppressing it, thus mitigating the negative effects of senescent cells without necessarily eliminating them → senostasis. Both approaches hold promise for treating age-related diseases and promoting healthy aging. By targeting senescent cells, researchers hope to develop therapies that can improve quality of life and extend lifespan. Senolytics are a class of drugs designed to selectively eliminate senescent cells. One of the earliest identified senolytic drug combinations is dasatinib and quercetin. This combination was discovered through a bioinformatics-based study. Dasatinib, an FDA-approved drug for cancer, is a tyrosine kinase inhibitor that can block cell proliferation and migration, and induce cell death. Quercetin, a naturally occurring flavonoid, has various biological activities including interactions with PI3K and Bcl-2 family proteins, which can contribute to its senolytic effects. The combination of dasatinib (D) and quercetin (Q), known as D+Q, has shown promise in treating several age- related conditions in mice, including frailty, osteoporosis, hepatic steatosis (fatty liver), insulin resistance, neurodegeneration, pulmonary fibrosis, chronic kidney disease, and skeletal muscle dysfunction. This senolytic therapy works by targeting and eliminating senescent cells, which contribute to these diseases. Building on these promising results, D+Q is now being tested in human clinical trials for conditions like idiopathic pulmonary fibrosis, chronic kidney disease, skeletal health, hematopoietic stem cell transplant survivors, and Alzheimer’s disease. These trials are critical for determining the safety and efficacy of D+Q in humans, offering potential treatments for age-related diseases and improving quality of life. Senostatics are an emerging and promising approach in senotherapy and focus on suppressing the harmful effects of senescent cells without necessarily removing them. This is particularly useful because eliminating all senescent cells is not always desirable; some senescent cells can play important roles in tissue repair and immune function. Instead of removing these cells, senostatics work by targeting key signaling pathways that drive the harmful effects of senescence, particularly SASP. For instance, NF-κB, JAK-STAT, and mTOR are critical pathways involved in both the induction and maintenance of the SASP. By targeting these pathways, senostatics 7 can reduce the secretion of harmful molecules, thereby mitigating the detrimental impact of senescent cells on surrounding tissues. A notable example of a senostatic drug is rapamycin, a macrolide compound that inhibits mTOR, a central regulator of cell growth and metabolism. Rapamycin has been shown to reduce the SASP, and it has demonstrated the ability to improve healthspan and extend lifespan in various model organisms. Rapamycin, initially developed as an anti-fungal agent, was later found to have immunosuppressive and anti-proliferative properties, leading to its FDA approval for preventing organ rejection in kidney transplant patients. As research progressed, rapamycin's ability to reduce cellular senescence, suppress SASP, and extend lifespan was discovered. When administered late in life, it extended both the median and maximum lifespan of mice, highlighting its potential for promoting healthy aging. Rapamycin works by inhibiting the mTOR pathway. MTOR exists in two complexes, TORC1 and TORC2, with rapamycin specifically inhibiting TORC1. This inhibition reduces protein synthesis, promotes autophagy, and suppresses the harmful effects of senescent cells, making rapamycin a promising approach for targeting aging-related diseases and extending healthspan. Another senostatic compound is resveratrol which prevents cellular senescence in endothelial progenitor cells by activating telomerase through the PI3K-Akt signaling pathway, helping maintain cell function. In neural cells, resveratrol alleviates senescence by activating SIRT1, which then triggers STAT3 signaling to reduce cellular stress and inflammation. In human bone marrow stromal stem cells, resveratrol inhibits senescence and suppresses SASP factors by blocking the NF-κB pathway, which regulates inflammation. Additionally, sirtuin-activating compounds (STACs) like resveratrol enhance the activity of sirtuins, enzymes that promote cellular repair and stress resilience, similar to the effects of caloric restriction, which also activates sirtuins and reduces SASP. These mechanisms make resveratrol and similar compounds promising tools for mitigating aging and promoting healthy longevity. Metformin has been FDA-approved for over 60 years to treat type 2 diabetes, but recent research suggests it also offers benefits for aging and cellular senescence. One key finding is that metformin reduces cellular SA-β-gal activity which may help limit the accumulation of senescent cells linked to aging and age-related diseases. Metformin also down-regulates senescence markers and SASP factors in various cell types, such as human diploid fibroblasts and lens epithelial cells, reducing inflammation and tissue damage caused by senescent cells. Additionally, it has been shown to increase healthspan and lifespan in model organisms like Caenorhabditis elegans and mice, independent of its effects on diabetes, suggesting it may have broader benefits for promoting healthy aging and longevity. Biguanides, especially metformin, are effective insulin sensitizers commonly used in the treatment of type 2 diabetes. Metformin works by improving peripheral glucose utilization, increasing glucose uptake by muscles, and enhancing glycolysis, which helps lower blood sugar levels. It also inhibits hepatic gluconeogenesis, reducing the liver's production of glucose and further controlling blood sugar. In addition to these effects, metformin improves insulin sensitivity, making the body more responsive to insulin. It helps control intestinal glucose absorption, preventing excess glucose from entering the bloodstream. It also rebalances lipid metabolism, lowering triglycerides and LDL cholesterol, and has anticoagulant and fibrinolytic properties, which help prevent blood clots and promote clot breakdown. 8 As already sain, metformin primarily works by inhibiting the production of glucose in the liver. It achieves this by targeting the mitochondria, specifically by inhibiting complex I of the electron transport chain. This inhibition leads to a decrease in ATP production and an increase in AMP levels. The increased AMP activates AMP-activated protein kinase (AMPK), which in turn phosphorylates and inhibits transcription factors like CBP and CRTC2, leading to decreased expression of gluconeogenic genes. As a result, the liver produces less glucose, and blood sugar levels are lowered. Additionally, metformin promotes glucose uptake in peripheral tissues and increases fatty acid oxidation, further contributing to its glucose- lowering effect. Metformin has a relatively short plasma half-life of about 2 hours, meaning it is quickly cleared from the body. It exhibits minimal protein binding, allowing it to remain free in the bloodstream and readily available to exert its effects. It is not extensively metabolized in the liver; instead, most of the drug is excreted unchanged through the kidneys. Around 30% of the orally administered dose is not absorbed and is eliminated in the feces. It is used as a first-line treatment in monotherapy in all subjects with type 2 diabetes who are unable to achieve glycemic compensation with diet and exercise, while in combination with other hypoglycemic drugs, if the latter are no longer able to achieve good glycemic control. Metformin is being explored for its potential as a senolytic drug, targeting senescent cells that contribute to aging and age-related diseases. The ongoing TAME trial (Targeting Aging with Metformin) is investigating whether metformin can slow aging and improve healthspan by reducing senescent cell burden. And to fully validate metformin as a senolytic, large-scale randomized controlled trials are needed to assess its safety, efficacy, and impact on aging. Moreover, combining lifestyle changes, dietary supplements, and medications could provide valuable insights into more effective approaches for slowing the aging process and enhancing long-term health. 9