Nucleophilic Substitution Reaction PDF
Document Details
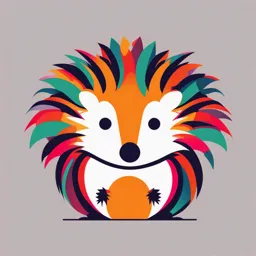
Uploaded by MajesticAntagonist650
Integral University
Mohd Anas
Tags
Summary
These lecture notes cover nucleophilic substitution reactions, specifically SN1 and SN2 reactions. They discuss reaction mechanisms, carbocation stability, and the effects of solvents and leaving groups on reaction rates. The document also features examples and diagrams.
Full Transcript
Nucleophilic Substitution Reaction Mohd Anas Lecturer Department of Pharmacy Integral University Lucknow(U.P.), India PC: Microsoft Copilot Nucleophilic Substitution Reaction A nucl...
Nucleophilic Substitution Reaction Mohd Anas Lecturer Department of Pharmacy Integral University Lucknow(U.P.), India PC: Microsoft Copilot Nucleophilic Substitution Reaction A nucleophilic substitution reaction involves the Example: replacement of a group (leaving group) on a CH3Br + NaOH → CH3OH + NaBr saturated carbon atom In this reaction : Methyl Bromide (CH₃Br) with a nucleophile. This is the substrate. process is characterized Bromide Ion (Br⁻) is the leaving group. by the nucleophile attacking the carbon Hydroxide Ion (OH⁻) is the nucleophile. atom and displacing the leaving group. SN1 (Substitution Nucleophilic Unimolecular) where: Substitution refers to the replacement of one group The SN1 mechanism involves with another. the substitution of a leaving Nucleophilic indicates that group in an organic molecule the reaction involves a by a nucleophile. The "SN1" nucleophile, which is an designation stands for electron-rich species that "Substitution Nucleophilic donates a pair of electrons. Unimolecular," Unimolecular signifies that the rate-determining step involves only one molecule, the substrate, which is undergoing the reaction. Mechanism Slow Fast R X R Carbocation X R Nu R Nu Intermediate Step 1: Formation of Carbocation: The leaving Step 2: Nucleophilic Attack: group departs, forming a The nucleophile then attacks the carbocation intermediate. This carbocation, leading to the step is the slow, rate- formation of the final product. determining Mechanism Tertiary alkyl halides undergo hydrolysis in the presence of water or alcohols, forming tertiary alcohols, tert-butyl chloride reacts with water to form tert-butanol and hydrochloric acid. CH3 CH3 Slow Step 1: CH3 C Cl CH3 C Cl CH3 CH3 CH3 CH3 Fast Step 2: CH3 C H 2O CH3 C OH H CH3 CH3 Energy Diagram An energy diagram (Following figure) for SN1 reaction involves two transition states. The transition state for the slow step (the ionization step) is higher in energy than the transition state for the fast step (second step, the capture of the ion by a nucleophile). Kinetics of SN1 First-Order Reaction: The rate of an SN1 reaction is first-order with respect to the substrate. This indicates that the rate-determining step (the formation of the carbocation) involves only one molecule, the substrate. The nucleophile's concentration does not affect the rate of this step. Rate=k[R-X] Rate is the reaction rate. k is the rate constant. [R-X] is the concentration of the substrate (alkyl halide). Stereochemistry of the SN1 Reaction Planar Carbocation: The carbocation intermediate is planar, allowing the nucleophile to approach from either the front or the back side. This leads to a mixture of products, as the nucleophile can attack from both sides of the planar carbocation. Racemization: If the substrate is chiral, the formation of a racemic mixture occurs because the nucleophile can attack from either face of the carbocation. Stereochemistry of the SN1 Reaction The stereochemistry of the SN1 reaction is characterized by the formation of a racemic mixture due to the planar nature of the carbocation intermediate, which allows nucleophilic attack from either side. The reaction is influenced by substrate structure, solvent, and the stability of the carbocation formed during the process. Carbocation and their Stability Carbocations are positively charged carbon species that play a crucial role in many organic reactions, particularly in the SN1 (substitution nucleophilic unimolecular) mechanism. The stability of carbocations significantly influences the rate and outcome of these reactions. Carbocations can be classified based on the number of carbon atoms attached to the positively charged carbon: 1. Tertiary Carbocations (3°): Formed when the positively charged carbon is attached to three other carbon atoms. Most stable due to hyperconjugation and inductive effects from adjacent alkyl groups. Carbocation and their Stability Secondary Carbocations (2°): Formed when the positively charged carbon is attached to two other carbon atoms. Less stable than tertiary carbocations but more stable than primary. Primary Carbocations (1°): Formed when the positively charged carbon is attached to only one other carbon atom. Least stable and often not formed under normal conditions Carbocation and their Stability The stability Hyperconjugation: The ability of adjacent C-H or C-C bonds to donate electron density to the empty of p-orbital of the carbocation stabilizes it. Tertiary carbocations benefit the most from this effect as they have more adjacent alkyl groups. carbocations Inductive Effect: Alkyl groups are electron- donating due to their inductive effect, which helps is influenced to stabilize the positive charge on the carbocation. The more alkyl groups attached to the carbocation, the greater the stabilization. by several Resonance: Carbocations can be stabilized by resonance if they are adjacent to double bonds or aromatic systems. This delocalization of the factors: positive charge can significantly enhance stability. Rearrangement of Carbocation Carbocation rearrangement. This occurs when the initially formed carbocation can undergo structural changes to form a more stable carbocation before the nucleophilic attack takes place. Rearrangement typically happens through two main processes: hydride shifts and alkyl shifts. Hydride Shift: A hydride shift involves the movement of a hydrogen atom (along with its bonding electrons) from an adjacent carbon atom to the positively charged carbocation. Alkyl Shift: An alkyl shift involves the migration of an alkyl group (a carbon chain) from an adjacent carbon to the carbocation center. Rearrangement of Carbocation Example: The reaction begins with the departure of the bromide from the neopentyl bromide, leading to the formation of a primary carbocation at the neopentyl position. This primary carbocation is very unstable, which triggers a rearrangement. To stabilize the carbocation, a methyl shift occurs from one of the adjacent tertiary carbons. This shift moves one of the methyl groups to the carbon with the positive charge, leading to the formation of a more stable tertiary carbocation. Once the more stable tertiary carbocation is formed, it can be attacked by a nucleophile Effect of Solvent on SN1 Reactions As a result, the reaction Polar Protic They stabilize This proceeds Solvents: the stabilization faster in polar These carbocation lowers the protic solvents solvents, intermediate energy barrier (Water (H₂O) which contain formed during for the rate- Methanol hydrogen the reaction by determining (CH₃OH) The SN1 atoms bonded solvation (the step, which is Ethanol favors to process of the (CH₃CH₂OH) electronegativ surrounding dissociation of Acetic Acid e atoms (like - and stabilizing the leaving (CH₃COOH) OH or -NH ions or group and Formic Acid groups), are molecules by formation of (HCOOH) preferred in solvent the Isopropanol SN1 reactions. molecules). carbocation. (CH₃CHOHC H₃) n-Butanol (C₄H₉OH) Effect of Nucleophile on SN1 Reactions In SN1 reactions, the nucleophile does not participate in the However, the rate-determining nucleophile must If multiple The resulting step (the first step), be able to nucleophiles are products will as the rate depends effectively attack present, their depend on their only on the the carbocation in concentrations and concentrations and formation of the the second step, relative reactivities reactivities, carbocation. but its nature does can influence the leading to different Therefore, the not influence the distribution of product strength or speed of the first products formed. distributions concentration of step. the nucleophile has little to no effect on the reaction rate. Effect of Leaving Group on SN1 Reactions These are typically weak bases that The leaving group is a crucial factor in can stabilize the negative charge after SN1 reactions as it directly impacts the leaving Such as halides (like iodide and reaction rate. A good leaving group bromide) and sulfonate groups (like facilitates the formation of the tosylate and mesylate). The better the carbocation by breaking away easily leaving group, the faster the formation from the substrate. of the carbocation, thus accelerating the overall reaction SN2 (Substitution Nucleophilic Bimolecular) The SN2 reaction, or Substitution Nucleophilic Bimolecular, is a type of nucleophilic substitution reaction where a nucleophile attacks an electrophilic carbon atom simultaneously as a leaving group departs. This mechanism is characterized by a one-step process that involves the formation of a bond between the nucleophile and the substrate while breaking the bond between the substrate and the leaving group. Bimolecular Nature: The reaction involves two reactants in the rate-determining step, leading to second-order kinetics. The rate depends on the concentrations of both the nucleophile and the substrate. SN2 (Substitution Nucleophilic Bimolecular) Nucleophiles A nucleophile is a species that donates an electron pair to form a chemical bond in a reaction. Nucleophiles are typically characterized by their availability of lone pairs of electrons or negative charges, which enable them to attack electrophilic centers (usually carbon atoms in organic molecules). Common examples of nucleophiles include: For Eg: Iodide ion (I⁻): A strong nucleophile due to its size and polarizability. Hydroxide ion (OH⁻): A strong nucleophile and base. Leaving Groups A leaving group is an atom or group of atoms that detaches from the substrate during a reaction, allowing for the formation of new bonds. Kinetics of SN2 Rate=k[R X][Nu−] Rate is the reaction rate. k is the rate constant. [R-X] is the concentration In contrast, a second-order of the substrate (alkyl reaction involves two halide). reactants in the rate- [Nu−] is the concentration The rate of an SN2 reaction is determining step. For of the nucleophile directly proportional to the bimolecular reactions like In this case, if either concentrations of both the SN2, this means that the rate concentration is increased, substrate and nucleophile depends on the concentrations the overall reaction rate will of both the nucleophile and increase proportionally. the substrate: This second-order behavior is characteristic of SN2 reactions where both reactants participate in forming the transition state Mechanism of SN2 Reaction PC: chem.libretexts.org Stereochemistry and Steric Hindrance in SN2 Reactions Stereochemistry: SN2 reactions result in inversion of configuration at the chiral carbon center due to the backside attack of the nucleophile. This phenomenon is known as Walden inversion. If the substrate has a specific stereochemistry (e.g., R or S), the product will have the opposite configuration after the reaction. Steric Hindrance: The rate of SN2 reactions is significantly affected by steric hindrance around the electrophilic carbon. Methyl and primary substrates react fastest because they have minimal steric hindrance, allowing easier access for the nucleophile. Secondary substrates are slower, while tertiary substrates are generally unreactive in SN2 pathways due to excessive steric hindrance that prevents nucleophilic attack. Role of Solvents in SN2 Reactions Solvent Effects: The choice of solvent plays a In contrast, polar protic solvents (e.g., water, crucial role in SN2 reactions. Polar aprotic alcohols) can solvate nucleophiles too strongly, solvents (e.g., acetone, DMSO) are preferred as reducing their reactivity by forming hydrogen they enhance nucleophilicity by solvating cations bonds. while leaving nucleophiles relatively free. SN1 VS SN2 Characteristics SN1 SN2 Mechanism Stepwise (two-step) Concerted (single-step) Stereochemistry Racemization (no net inversion) Inversion of configuration Rate = k[substrate][nucleophile] Rate = k[substrate] (depend on Substrate Rate Law (dependent on nucleophile & Concentration) Substrate) Substrate Preference Tertiary > secondary > primary Primary > secondary > tertiary Weak nucleophiles (e.g. water, Strong nucleophiles (e.g. hydroxide, Nucleophile Preference methanol) cyanide) Polar aprotic solvents (e.g. DMSO, Solvent Preference Polar protic solvents DMF) Typically carried out at higher Typically carried out at lower Reaction Conditions temperatures temperatures Reaction Rate Slower reaction rate Faster reaction rate Phase Transfer Catalyst Phase-transfer catalysis (PTC) involves using a catalyst to facilitate the transfer of reactants between immiscible phases (e.g., aqueous and organic phases) to enhance reaction rates. PTC is particularly useful for reactions involving ionic species that are poorly soluble in organic solvents. It allows for faster reactions and higher yields without requiring expensive or hazardous solvents. References 1. Morrison, R. T., & Boyd, R. N. (2010). Organic Chemistry (7th ed.). Pearson Education. 2. Solomons, T. W. G., & Fryhle, C. B. (2011). Organic Chemistry (10th ed.). Wiley. 3. Clayden, J., Greeves, N., Warren, S., & Wothers, P. (2012). Organic Chemistry (2nd ed.). Oxford University Press. 4. Bruice, P. Y. (2016). Organic Chemistry (8th ed.). Pearson. 5. McMurry, J. (2015). Organic Chemistry (9th ed.). Cengage Learning. 6. ChemLibreTexts. (n.d.). SN2 reaction mechanism energy diagram and stereochemistry. Organic Chemistry I: Nucleophilic Substitution Reactions. Retrieved from https://chem.libretexts.org/Bookshelves/Organic_Chemistry/Organic_Chemistry_I_(Li u)/07:_Nucleophilic_Substitution_Reactions/7.02:_SN2_Reaction_Mechanism_Energy _Diagram_and_Stereochemistry 7. Microsoft Copilot. (2024). Image generated using Microsoft Copilot for slides in Nucleophilic Substitution Reaction Thank You Contact us: [email protected], [email protected] PC: Microsoft Copilot