Nuclear Chemistry Review - CHM 101 PDF
Document Details
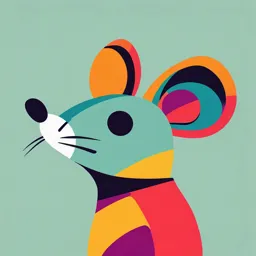
Uploaded by SharpHafnium
LASU
Tags
Summary
This document reviews nuclear chemistry, focusing on radioactivity and nuclear reactions. It describes the types of radioactive decay, such as alpha, beta, and gamma decay, and provides examples of nuclear equations.
Full Transcript
**CHM 101** **RADIOACTIVITY** In **chemical reactions**, only the outer electrons of the atoms are disturbed. The nuclei of the atoms are not affected. In *nuclear reactions, however, the nuclear changes that occur are independent of the chemical environment of the atom*. For example, the nuclear...
**CHM 101** **RADIOACTIVITY** In **chemical reactions**, only the outer electrons of the atoms are disturbed. The nuclei of the atoms are not affected. In *nuclear reactions, however, the nuclear changes that occur are independent of the chemical environment of the atom*. For example, the nuclear changes in a radioactive [~1~^3^*H*]{.math.inline} atom are the same if the atom is part of an H~2~ molecule or incorporated into H~2~O. There are two types of Nuclear reactions: 1. Radoaactive decay 2. Nuclear bombardment reaction **Radioactive Decay (Radioactivity)** **Radioactive decay** is the process in which a nucleus spontaneously disintegrates, giving off radiation. The radiation consists of one or more of the following, depending on the nucleus**: electrons**, nuclear particles (such **as neutrons**), smaller nuclei (usually **helium-4 nuclei**), and **electromagnetic radiation.** The phenomenon of radioactivity was discovered by **Antoine Henri Becquerel** in **1896**. He discovered that photographic plates develop bright spots when exposed to *uranium minerals*, and he concluded that the minerals give off some sort of radiation. The radiation from uranium minerals was later shown to be separable by electric (and magnetic) fields into three types: **alpha (**[**α**]{.math.inline}**),** **beta (**[**β**]{.math.inline}**),** and **gamma (**[**γ**]{.math.inline}**) rays.** Alpha rays bend away from a positive plate and toward a negative plate, indicating that they have a **positive charge**; they are now known to consist of helium-4 nuclei (nuclei with two protons and two neutrons). Beta rays bend in the opposite direction, indicating that they have a **negative charge**; they are now known to consist of high-speed electrons. Gamma rays are unaffected by electric and magnetic fields: they have been shown to be a form **of electromagnetic radiation** that is similar to x rays, except they are higher in energy with shorter wavelengths (about 1 pm, or [1 × 10^12^ m]{.math.inline} ). **Figure 1: Separation of the radiation from a radioactive material (uranium mineral)** Uranium minerals contain a number of radioactive elements, each emitting one or more of these radiations. Uranium-238, the main uranium isotope in uranium minerals, emits alpha rays and thereby decays, or disintegrates, to thorium-234 nuclei. **Nuclear Equations** We can write an equation for the nuclear reaction corresponding to the decay of uranium-238 much as we would write an equation for a chemical reaction. You represent the uranium-238 nucleus by the nuclide symbol [~92~^238^*U*]{.math.inline}. The radioactive decay of [~92~^238^*U*]{.math.inline} by alpha-particle emission (loss of a [~2~^4^He]{.math.inline} nucleus) is written as:  The product, in addition to helium-4, is thorium-234. This is an example of a nuclear equation, which is a symbolic representation of a nuclear reaction. Reactant and product nuclei are represented in nuclear equations by their nuclide symbols. Other particles are given the following symbols, in which the subscript equals the charge and the superscript equals the total number of protons and neutrons in the particle (mass number): The decay of a nucleus with the emission of an electron, [~ − 1~^0^*e*]{.math.inline}, is usually called **beta emission**, and the emitted electron is sometimes labeled [~ − 1~^0^*β*]{.math.inline}. **A positron** is a particle similar to an electron, having the same mass but a positive charge. Positrons are annihilated as soon as they encounter electrons. When a positron and an electron collide, both particles vanish with the emission of two gamma photons that carry away the energy.  **A gamma photon** is a particle of electromagnetic radiation of short wavelength (about 1 pm, or [1 × 10^12^ m]{.math.inline}) and high energy.  The total charge is conserved, or remains constant, during a nuclear reaction. This means that the sum of the subscripts (number of protons, or positive charges, in the nuclei) for the products must equal the sum of the subscripts for the reactants. Similarly, the total number of nucleons (protons and neutrons) is conserved, or remains constant, during a nuclear reaction. This means that the sum of the superscripts (the mass numbers) for the reactants equals the sum of the superscripts for the products. **Note that if all reactants and products but one are known in a nuclear equation, the identity of that one nucleus or particle can be easily obtained.** This is illustrated in the next example: **Example 20.2** Technetium-99 is a long-lived radioactive isotope of technetium. Each nucleus decays by emitting one beta particle. What is the product nucleus?   **Exercise 20.2** Plutonium-239 decays by alpha emission, with each nucleus emitting one alpha particle. What is the other product of this decay? **Nuclear Stability** The existence of stable nuclei with more than one proton is due to the nuclear force. The nuclear force is a strong force of attraction between nucleons that acts only at very short distances (about [10^ − 15^*m*]{.math.inline}). *Beyond nuclear distances, these nuclear forces become negligible.* Therefore, two protons that are much farther apart than [10^ − 15^*m*]{.math.inline} repel one another by their like electric charges. Inside the nucleus, however, two protons are close enough together for the nuclear force between them to be effective. This force in a nucleus can more than compensate for the repulsion of electric charges and thereby give a stable nucleus. Nuclear stability depends on three different factors: i. **Presence of Magic numbers** ii. **Deviation from Band of Stability \-\--Neutron to proton ratio (N/P)** iii. **Pairs of Protons and pairs of Neutrons** The protons and neutrons in a nucleus appear to have energy levels much as the electrons in an atom have energy levels. The shell model of the nucleus is a nuclear model in which protons and neutrons exist in levels, or shells, analogous to the shell structure that exists for electrons in an atom. Experimentally, note that nuclei with certain numbers of protons or neutrons appear to be very stable. These numbers**, called magic numbers** and associated with specially stable nuclei, were later explained by the shell model. According to this theory, **a magic number is the number of nuclear particles in a completed shell of protons or neutrons.** Because nuclear forces differ from electrical forces, these numbers are not the same as those for electrons in atoms. **For protons, the magic numbers are 2, 8, 20, 28, 50, and 82.** **Neutrons have these same magic numbers, as well as the magic number 126. For protons, calculations show that 114 should also be a magic number.** Evidence also points to the special stability of pairs of protons and pairs of neutrons, analogous to the stability of pairs of electrons in molecules. Table 20.1 lists the number of stable isotopes that have an even number of protons and an even number of neutrons (157). By comparison, only 5 stable isotopes have an odd number of protons and an odd number of neutrons. **Band of Stability** When you plot each stable nuclide on a graph with the number of protons (Z) on the horizontal axis and the number of neutrons (N) on the vertical axis, these stable nuclides fall in a certain region, or band, of the graph. **The band of stability is the region in which stable nuclides lie in a plot of number of protons against number of neutrons.**  **Figure 2: Band of Stability** The stable nuclides, indicated by black dots, cluster in a band. Nuclides to the left of the band of stability usually decay by beta emission, whereas those to the right usually decay by positron emission or electron capture. Nuclides of Z \> 83 often decay by alpha emission. **Nuclear Decay** For nuclides up to Z = 20, the ratio of neutrons to protons is about 1.0 to 1.1. As Z increases, however, the neutron-to-proton ratio increases to about 1.5. This increase in neutron-to-proton ratio with increasing Z is believed to result from the increasing repulsions of protons from their electric charges. More neutrons are required to give attractive nuclear forces to offset these repulsions. It appears that when the number of protons becomes very large, the proton--proton repulsions become so great that stable nuclides are impossible. **No stable nuclides are known with atomic numbers greater than 83**. On the other hand, all elements with Z equal to 83 or less have one or more stable nuclides, with the exception of technetium (Z = 43) and promethium (Z = 61). **Exercise 20.3: Predicting the Relative Stabilities of Nuclides**  **Types of Radioactive Decay** There are six common types of radioactive decay; the first five are listed in Table 20.2. Nuclides outside the band of stability (Figure 20.3) are generally radioactive. **Nuclides to the left of the band of stability have a neutron-to-proton ratio (N/Z) larger than that needed for stability.** *These nuclides tend to decay by beta emission*. Beta emission reduces the neutron-to-proton ratio, *because in this process a neutron is changed to a proton.* The product is a stabler nuclide. In contrast, **nuclides to the right of the band of stability have a neutron-to-proton ratio smaller than that needed for stability.** *These nuclides tend to decay by either positron emission or electron capture.* **Both processes convert a proton to a neutron, increasing the neutron-to-proton ratio** and giving a stabler product nuclide. The types of radioactive decay expected of unstable nuclides are noted in Figure 20.3 1. **Alpha emission** . **Beta emission** ([~ − 1~^0^*e*]{.math.inline}) This is the emission of a high-speed electron from an unstable nucleus. Beta emission is equivalent to the conversion of a neutron to a proton. An example of beta emission is the radioactive decay of carbon-14. The product nucleus has an atomic number that is one more than that of the original nucleus. The mass number remains the same . **Positron emission (**[~ + 1~^0^*e*]{.math.inline})) *This is the emission of a positron from an unstable nucleus*. Positron emission is equivalent to the conversion of a proton to a neutron. The radioactive decay of technetium-95 is an example of positron emission.  *The product nucleus has an atomic number that is one less than that of the original nucleus. The mass number remains the same.* *.* ***Electron capture (abbreviated EC):*** *This is the decay of an unstable nucleus by capturing, or picking up, an electron from an inner orbital of an atom. In effect, a proton is changed to a neutron, as in positron emission.* An example is given by potassium-40, which has a natural abundance of 0.012%. Potassium-40 can decay by electron capture, as well as by beta and positron emissions. The equation for electron capture is:  *The product nucleus has an atomic number that is one less than that of the original nucleus. The mass number remains the same.* *.* **Gamma emission (**[~**0**~^**0**^**γ**]{.math.inline}*)* *This is the emission from an excited nucleus of a gamma photon, corresponding to radiation with a wavelength of about* [1 × 10^ − 12^*m*]{.math.inline}*. In many cases, radioactive decay results in a product nucleus that **is in an excited state**.* As in the case of atoms, the excited state is unstable and goes to a lower-energy state with the emission of electromagnetic radiation. For nuclei, this radiation is in the gamma-ray region of the spectrum. Often gamma emission occurs very quickly after radioactive decay. In some cases, however, an excited state has significant lifetime before it emits a gamma photon. **A metastable nucleus is a nucleus in an excited state with a lifetime of at least one nanosecond (**[1 × 10^ − 9^*s*]{.math.inline} **).** In time, the metastable nucleus decays by gamma emission. An example is metastable technetium-99, denoted by [~43~^99*m*^Tc]{.math.inline}, which is used in medical diagnosis. The product nucleus is simply a lower-energy state of the original nucleus, so there is no change of atomic number or mass number. **Spontaneous fission** This is the spontaneous decay of an unstable nucleus in which a heavy nucleus of mass number greater than 89 splits into lighter nuclei and energy is released. For example, a uranium-236 atom can spontaneously undergo the following nuclear reaction:  **Further Examples of Radioactive Decay** Consider a series of isotopes of a given element, such as carbon. Carbon-12 and carbon-13 are stable isotopes, whereas the other isotopes of carbon are radioactive. The isotopes of mass number smaller than 12 decay by positron emission. For example, carbon-11 decays by positron emission to boron-11: Carbon-11 has a neutron-to-proton ratio of 5/6 (0.8), which increases in the product boron-11 to 6/5 (1.2). The isotopes of carbon with mass number greater than 13 decay by beta emission. Carbon-14 decays by beta emission to produce nitrogen-14.  Carbon-14 has a neutron-to-proton ratio of 8/6 (1.3), which decreases in the product nitrogen-14 to 7/7 (1.0). **Note:** *Positron emission and electron capture* are competing radioactive decay processes, and what is observed depends on the relative rates of the two processes. *The rate of **electron capture** increases with atomic number of the decaying nuclide and therefore becomes i**mportant in heavier elements.*** ***Positron emission** is generally seen **in lighter elements*****.** However, *in the very heavy elements, especially those with Z greater than 83, radioactive decay is often **by alpha emission.***  **Radioactive decay Series** *All nuclides with atomic number greater than Z = 83 are radioactive.* Many of these nuclides decay by alpha emission. Alpha particles, or [~2~^4^He]{.math.inline} nuclei, are especially stable and are formed in the radioactive nucleus at the moment of decay. By emitting an alpha particle, the nucleus reduces its atomic number, becoming more stable. However, if the nucleus has a very large atomic number, the product nucleus is also radioactive. Natural radioactive elements, such as uranium-238, give a radioactive decay series. **Radioactive series is a sequence in which one radioactive nucleus decays to a second, which then decays to a third, and so forth.** Eventually, a stable nucleus, which is an isotope of lead, is reached. Three radioactive decay series are found naturally. One of these series begins with uranium-238. Figure 3 shows the sequence of nuclear decay processes. In the first step, uranium-238 decays by alpha emission to thorium-234.  Thorium-234 in turn decays by beta emission to protactinium-234, which decays by beta emission to uranium-234. After the decay of protactinium-234 and the formation of uranium-234, there are a number of alpha-decay steps. The final product of the series is lead-206.  **Figure 3: Uranium-238 radioactive decay series** Natural uranium is 99.28% [~92~^238^*U*]{.math.inline}, which decays as we have described. However, the natural element also contains 0.72% [~92~^239^*U*]{.math.inline}. This isotope starts *a second radioactive decay series, which consists of a sequence of alpha and beta decays, ending with lead-207.* *The third naturally occurring radioactive decay series begins with thorium-232 and ends with lead-208.* **All three radioactive decay series found naturally end with an isotope of lead.** **NUCLEAR BOMBARDMENT** The nuclear reactions discussed in the previous section are radioactive decay reactions, in which a nucleus spontaneously decays to another nucleus and emits a particle, such as an alpha or beta particle. The second type of nuclear reaction is a **nuclear bombardment reaction**, a nuclear reaction in which a nucleus is bombarded, or struck, by another nucleus or by a nuclear particle. If there is sufficient energy in this collision, the nuclear particles of the reactants rearrange to give a product nucleus or nuclei. In 1919, **Ernest Rutherford** discovered that it is possible to change the nucleus of one element into the nucleus of another element by processes that can be controlled in the laboratory. **Transmutation** **Transmutation is the change of one element to another by bombarding the nucleus of the element with nuclear particles or nuclei.** Rutherford used a radioactive element as a source of alpha particles and allowed these particles to collide with nitrogen nuclei. He discovered that protons are ejected in the process. The equation for the nuclear reaction is given below: The experiments were repeated on other light nuclei, most of which were transmuted to other elements with the ejection of a proton. These experiments yielded two significant results. *First, they strengthened the view that all nuclei contain protons.* *Second, they showed for the first time that it is possible to change one element into another under laboratory control.* When beryllium is bombarded with alpha particles, a penetrating radiation is given off that is not deflected by electric or magnetic fields. The particles are called neutrons. The reaction that resulted in the discovery of the neutron is:  *Phosphorus-30 was the first radioactive nucleus produced in the laboratory*. Since then over a thousand radioactive isotopes have been made. Nuclear bombardment reactions are often referred to by an abbreviated notation. For example, the nuclear reaction below is abbreviated as:  *In this, notation, you first write the nuclide symbol for the original nucleus (target). Then, in parentheses, you write the symbol for the projectile particle (incoming particle), followed by a comma and the symbol for the ejected particle. After the last parenthesis, you write the nuclide symbol for the product nucleus.* The following symbols are used for particles: Technetium was first prepared by directing deuterons, or nuclei of hydrogen-2 atoms, from a cyclotron to a molybdenum target. The nuclear reaction is:   **Determining the Product Nucleus in a Nuclear Bombardment Reaction**  **Trans-uranium Elements** The trans-uranium elements are elements with atomic numbers greater than that of uranium (Z = 92), **the naturally occurring element of greatest Z**. In 1940, E. M. McMillan and P. H. Abelson, at the University of California at Berkeley, discovered the first transuranium element. They produced an isotope of element 93, which they named **neptunium**, by bombarding uranium-238 with neutrons. This gave uranium-239, by the capture of a neutron, and this nucleus decayed in a few days by beta emission to neptunium-239.  The next transuranium element to be discovered was plutonium (Z = 94). Deuterons, the positively charged nuclei of hydrogen-2, were accelerated by a cyclotron and directed at a uranium target to give neptunium-238, which decayed to plutonium-238. Another isotope of plutonium, plutonium-239, is now produced in large quantity in nuclear reactors, as described in Section 20.7. Plutonium-239 is used for nuclear weapons. **Applications of Nuclear Radiations** 1. Preparation of elements not available naturally, especially the trans-uranium elements. 2. Radioactive decay of a nuclide is constant, this rate can serve as a clock for dating very old rocks and human implements. **Dating wood and similar carbon-containing objects** that are several thousand to fifty thousand years old can be done with **radioactive carbon, carbon-14, which has a half-life of 5730 y.** For the **age of rocks and meteorites**, other, similar methods of dating have been used. One method depends on the radioactivity of naturally occurring **potassium-40**, which decays by positron emission and electron capture (as well as by beta emission).  The **oldest rocks on earth** have been dated at **3.8 x 10^9^ years**. It is now believed from **radio-dating** and other evidence that **the age of the earth is 4.6 x 10^9^ years**. 3. Some radioisotopes are used as radioactive tracers in chemical analyses. *A radioactive tracer is a very small amount of radioactive isotope added to a chemical, biological, or physical system to study the system.* The advantage of a radioactive tracer is that it behaves chemically just as a nonradioactive isotope does, but it can be detected in exceedingly small amounts by measuring the radiations emitted. 4. Nuclear chemistry is used for analysis of elements in a sample using Neutron activation analysis. *Neutron activation is the analysis of elements in a sample based on the conversion of stable isotopes to radioactive isotopes by bombarding a sample with neutrons* 5. Radioisotopes are used in medicine in the treatment of cancer, for example, radium-226 and its decay product radon-222. 6. The greatest advances in the use of radioactive isotopes have been in the diagnosis of disease. **Technetium-99m** is the radioactive isotope used most often to develop images (pictures) **of internal body organs**. It has a half-life of 6.02 h, decaying by gamma emission to technetium-99 in its nuclear ground state. **Thallium-201** is a radioisotope used to determine whether a person **has heart disease** (caused by narrowing of the arteries to the heart). *More than a hundred different radioactive isotopes have been used in medicine.* *Besides technetium-99m and thallium-201, other examples include **iodine-131**, used* *to **measure thyroid activity**; **phosphorus-32**, used to **locate tumors**; and **iron-59**, used* *to measure the **rate of formation of red blood cells**.*