Biomedical and Environmental Applications of Nanotechnology PDF
Document Details
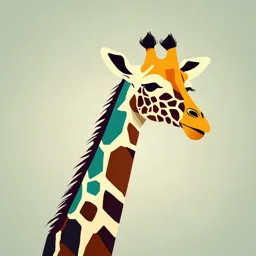
Uploaded by UnrealStatistics
Indian Institute of Technology Roorkee
Tags
Summary
This document provides an overview of biomedical and environmental applications of nanotechnology, focusing on therapeutic applications and drug delivery systems. It discusses the challenges in drug development and how nanotechnology can potentially overcome these obstacles.
Full Transcript
INDIAN INSTITUTE OF TECHNOLOGY ROORKEE Biomedical and Environmental Applications of Nanotechnology Therapeutic Application Nano-biotechnology 2 Drug Delivery An important and long-term goal of the pharmaceutical industry is to develop therapeuti...
INDIAN INSTITUTE OF TECHNOLOGY ROORKEE Biomedical and Environmental Applications of Nanotechnology Therapeutic Application Nano-biotechnology 2 Drug Delivery An important and long-term goal of the pharmaceutical industry is to develop therapeutic agents (DRUGS) that can be selectively delivered to specific areas in the body to maximize the therapeutic efficacy by transporting and releasing the drug (actively or passively). Challenges in Drug Development: A) poor solubility and biodistribution, therefore drugs does not interact with the site of action B) poor oral absorption C) low solubility at physiological pH D) insufficient cellular uptake E) rapid drug elimination F) adverse effects An answer to poor drug physiochemical properties is to associate the drug with a pharmaceutical carrier [i.e., a drug delivery system (DDS)] Afzal, Obaid, et al. "Nanoparticles in drug delivery: From history to therapeutic applications." Nanomaterials 12.24 (2022): 4494. 3 Drug Delivery Systems (DDS) A drug delivery system (DDS) can enhance a drug pharmacokinetics and cellular penetration. Moreover, obstacles arising from low drug solubility, degradation, fast clearance rates, nonspecific toxicity, and inability to cross biological barriers may also be addressed by a DDS. To be useful, a DDS is required to be biocompatible with processes in the body as well as with the drug to be delivered. Overall, the challenge of increasing a drug's therapeutic effect, with a concurrent minimization of side effects, can be optimized through proper design and DDS engineering Drug delivery systems for the diagnosis and/or therapy of various diseases 4 Biological Barriers Biological barriers like cell, nuclear, and endosomal membranes significantly impact drug delivery. When nanoparticles are administered, proteins form a "protein corona" on their surface, influenced by nanoparticle properties, which affects their uptake, biodistribution, immune response Macrophages in the reticuloendothelial system (RES) quickly clear nanoparticles, reducing their bioavailability. In the bloodstream, nanoparticles face complex fluid dynamics, and the extracellular matrix (ECM) further obstructs their transport due to its structural rigidity. After extravasation, nanoparticles must bind to cell membranes and navigate endocytosis and potential lysosomal degradation. Developing nanomedicines targeting specific molecules or immune cells at inflammation sites can overcome these obstacles, with surface modifications enhancing passive and active drug targeting. 5 Mechanisms of Targeted Drug Delivery Systems PASSIVE TARGETING Mechanism: Nanomedicines (NMs) exploit the enhanced permeability and retention (EPR) effect observed in tumors, where leaky blood vessels allow nanoparticles to extravasate into the tumor tissue. NMs accumulate in tumors based on their physicochemical properties and prolonged circulation half-life, without requiring specific ligand-receptor interactions with tumor cells. Effectiveness: NMs may have limited specificity for targeting tumor cells directly, potentially leading to variable therapeutic outcomes. ACTIVE TARGETING Mechanism: Nanomedicines are functionalized with targeting ligands (e.g., antibodies, peptides) that specifically recognize and bind to receptors or antigens overexpressed on tumor cells. NMs aim to enhance specificity and uptake by tumor cells, potentially improving therapeutic efficacy and reducing off-target effects compared to passive targeting nanomedicines. Attia, Mohamed F., et al. Journal of Pharmacy and Pharmacology 71.8 (2019): 1185-1198. 6 Nanoparticles as Drug Delivery Systems Advantages of Nano-Drug Delivery system: Enhanced stability and solubility Reduce toxicity Improve therapeutic efficacy Improve bioavailability of drugs Enhanced cellular uptake Targeting diseased sites Act as controlled release system Less amount of dose is required Nanoparticles commonly used as drug delivery systems. Each class has numerous advantages and disadvantages regarding cargo, delivery and patient response. Mitchell, Michael J., et al. "Engineering precision nanoparticles for drug delivery." Nature reviews drug discovery 20.2 (2021): 101-124. 7 Liposomes Liposomal classification based on number of lipid Liposome bilayer (lamellae) and the vesicle size. Phospholipid Bilayer Enclosing an Aqueous Core ▪ Small Unilamellar Vesicles (SUVs, 20-100 nm) ▪ Large Unilamellar Vesicles (LUV, >100 nm) Advantages of Liposomal Deliver ▪ Multilamellar Vesicles (MUV, >500 nm) High bioavailability and absorption compared with other oral ▪ Multi Vesicular Vesicles (MVV, >1000 nm) forms of supplements. Can hold both hydrophilic and hydrophobic drugs. Increased intracellular delivery. Guimarães, Diana,et al. "Design of liposomes as drug delivery system for therapeutic applications." International journal of pharmaceutics 601 (2021): 120571. 8 Different types of liposomes used in therapeutic applications Conventional liposomes composed Stealth or PEGylated liposomes of neutral, cationic or anionic charged involves coating liposomal phospholipids, and in combination membranes with biocompatible with cholesterol promote the hydrophilic polymers like PEG, stabilization of the liposomal bilayer chitosan to reduce immunogenicity Drawbacks: instability in plasma, and macrophage uptake, enhancing short blood circulation half-life, blood circulation and reducing rapidly captured by toxicity. However, this approach reticuloendothelial system and results in broad biodistribution, removed from the blood circulation. preventing selective delivery to specific target cells Ligand targeted liposomes are functionalized with PEG and ligands Multifunctional liposomes modified like glycoproteins, polysaccharides, for diverse functions, can carry both or specific receptor-targeting agents imaging and therapeutic agents for targeted delivery to desired (theranostic liposomes) or have dual- tissues, enhancing therapeutic targeting ligands for enhanced activity. These ligands binds to targeting receptors and overexpressed on diseased cells, minimizing off-target effects on healthy cells 9 Mucoadhesive liposomal delivery system combining anti-inflammation and anti-oxidation for improved treatment of dry eye disease Phenylboronic acid (PBA)-modified liposomes containing immunosuppressant cyclosporin A (CsA) and antioxidant crocin (Cro) adhere to the ocular surface by forming covalent bonds with mucin's sialic acid residues, enhancing drug retention. These liposomes inhibit ROS production and block the NF-κB inflammatory pathway, providing both anti-inflammatory and antioxidant effects. Consequently, they promote corneal healing and effectively relieve dry eye disease (DED). Huang, Kexin, et al." Journal of Controlled Release 368 (2024): 318-328. 10 Disrupting redox homeostasis for tumor therapy based on therapeutic hybrid liposomes Hybrid liposomes, composed of Ce6 lipid conjugates, encapsulate doxorubicin (DOX) and Fe3O4 nanoparticles, offering synergistic PDT/chemo/ferroptosis therapy. Photodynamic therapy (PDT) generates ROS under 650 nm light, Fe3O4 triggers Fenton reactions, and DOX induces apoptosis, amplifying oxidative stress in cancer cells. This disrupts cellular redox balance, promoting lipid, protein, and DNA damage, enhancing cancer cell death through combined apoptosis and ferroptosis induction. Huang, Yuanping, et al. RSC advances 14.28 (2024): 20152-20162. 11 Polymeric Nanoparticles Polymeric nanoparticles (NPs) are synthesized using techniques like emulsification, nanoprecipitation, ionic gelation, and microfluidics, each producing different outcomes. Biodegradable polymers like PLA, PLGA, and natural polymers such as chitosan are preferred due to their biocompatibility and biosafety. These NPs can deliver various therapeutics, encapsulating drugs within the core, entrapping them in the polymer matrix, chemically conjugating them to the polymer, or binding them to the NP surface. This versatility allows for the delivery of hydrophobic and hydrophilic compounds, as well as The two most common forms of polymeric NPs small molecules, biological macromolecules, proteins, and vaccines, making them ideal for co- delivery applications. nanocapsules (cavities surrounded by a polymeric membrane or shell) By adjusting composition, stability, responsivity, and surface charge, the loading efficacies and release kinetics of these therapeutics can be finely controlled. nanospheres (solid matrix systems). 12 A poly(amidoamine)-based polymeric nanoparticle platform for efficient in vivo delivery of mRNA ▪ The development of bioreducible poly(amidoamine)-based nanoparticles (PAA PNPs) for mRNA delivery has led to improved transfection efficiency. ▪ Functionalization with chloroquinoline (Q) enhances endosomal escape and stabilizes the mRNA-polymer construct, while PEG-polymer coatings improve stability and reduce surface charge. ▪ These enhancements resulted in significantly higher luciferase activity in mice muscle after intramuscular injection of luciferase mRNA-loaded PNPs. ▪ This technology is promising for creating new nucleotide-based vaccines and therapeutics for various diseases. Pontes, Adriano P., et al. Biomaterials advances 156 (2024): 213713. 13 pH-responsive copolymer synthesized via click chemistry for design of polymeric nanoparticles for targeted drug delivery Polymeric nanoparticles (PNPs) loaded with prednisolone are designed with pH-responsive properties using hydrazone linkages between chitosan and mPEG. In acidic environments typical of diseased tissues, these bonds break, releasing the drug at the targeted site. The PNPs are spherical, approximately 200 nm in size, with a negative surface charge and high encapsulation efficiency (71.1%). In cellular studies, these PNPs effectively reduce inflammatory markers such as nitric oxide (NO) and reactive oxygen species (ROS) in RAW264.7 cells, showing promise for treating both inflammatory conditions and cancers through their pH-responsive drug delivery system Antoniraj, Mariya Gover, et al.Carbohydrate Research (2024): 109200. 14 Co-delivery of fucoxanthin and siRNA using hydroxyethyl starch- cholesterol self-assembled polymer nanoparticles Researchers developed siTwist/FX@HES-CH nanomedicine by modifying hydroxyethyl starch (HES) with cholesterol (CH), which allows for efficient loading of negatively charged siRNA (siTwist) and creates pH-sensitive properties for targeted release in acidic tumor environments. These nanoparticles, sized between 100-200 nm, effectively accumulated in tumor tissues. They delivered both FX (fucoxanthin) and siTwist, synergistically eliminating triple- negative breast cancer (TNBC) cells and preventing fibroblasts from transforming into cancer-promoting cells called cancer-associated fibroblasts (CAFs). This approach reduced solid tumor pressure (STP), and facilitated effective treatment of primary TNBC and lung metastasis by remodeling the tumor microenvironment (TME) and targeting intracellular pathways Wu, Zeliang, et al. Journal of Advanced Research (2024). 15 Dendritic Nanoparticles as effective nanocarriers Typically, 1 to 10 nm in diameter, dendrimers have flexible branches that allow for strong multivalent binding with cell receptors, enhancing binding efficacy and surface targeting in both in vitro and in vivo applications. Dendrimers are characterized by a spherical shape, adaptable surface Dendrimers are determined by composition, and internal three unique components: hydrophobic pockets for (1) the initiator core, encapsulating drugs. (2) the interior layer, made up of Their unique properties, repetitive generations (units) including multivalency and connected with initiator core aqueous solubility, make (3) Surface (reactive terminal them ideal for drug delivery. group). Kim, DaWon, et al. Nanoscale (2024), Torabi Fard, Niloufar, et al. "Journal of Polymers and the Environment (2024): 1-27. 16 Folic acid conjugated poly (Amidoamine) dendrimer grafted magnetic chitosan as a smart drug delivery platform for doxorubicin Folate-targeted drug delivery systems (DDSs) are crucial in cancer therapy due to the specific binding of folic acid (FA) and its derivatives to folate receptors overexpressed on malignant tissues. This study developed a novel core-shell magnetic nanocarrier (MCS) by grafting generation 2 poly(amidoamine) (G2- PAMAM) dendrimer onto Fe3O4 nanoparticles and conjugating it with folic acid. This design offers dual pH/magnetic sensitivity for controlled doxorubicin (DOX) release, minimizing off-target effects. The efficiency of this bio-nanocomposite for drug loading, sustained release, and its cytotoxicity against MDA-MB-231 cancer cells was systematically evaluated to target Diagram illustrating the synthesis method and drug delivery mechanism of the folate receptors specifically. FA–PAMAMG2–MCS platform Soltany, Parva, Mahsasadat Miralinaghi, and Farshid Pajoum Shariati. International Journal of Biological Macromolecules 257 (2024): 127564. 17 Critical questions for designing nanoparticle delivery systems Question Rationale for the question The specific biological target (organ or tissue, cell (1) Where is the delivery target? type and subcellular location) defines design of the nanoparticle strategy. (2) What is the cargo or active agent that needs to be This defines the chemistry for incorporating the delivered to the target location? agents into the nanoparticle for delivery. The location of administration and the delivery (3) Where is the site of administration? target location define the delivery pathway. (4) What are the specific organs, tissues and cells This defines the barriers that the nanoparticle will encountered along the delivery pathway? encounter. (5) What are the interactions between the nanoparticle These interactions will determine if the carrier and the body in each of these biological formulation is degraded or sequestered before it environments along the delivery pathway? can reach its intended target location. (6) What strategies are available to overcome the barriers This allows the development of specific strategies at each step in the delivery pathway? to overcome the barriers. (7) How will any administered components leave the This helps to define locations of toxicity and disease site and be excreted from the body? elimination routes. 18 Guiding the delivery strategy 19 Future of Drug Delivery While the primary aim of drug delivery technologies is to ensure therapeutic concentrations of a drug at the target site, the future will likely focus on: A. enhancing targeting efficiency at both tissue and cellular levels, and improving delivery across barriers like the brain and lungs B. developing long-acting delivery systems for better pharmacokinetics and controlled release C. creating personalized drug delivery technologies D. integrating advanced technologies like AI, machine learning, and wireless electronics with drug delivery. Gao, Jingjing, et al. Chemistry of Materials 35.2 (2023): 359-363. 20 CANCER Cancer is ranked as the second leading cause of death globally. Cancer is a group of diseases which cause an abnormal and uncontrolled cell division coupled with malignant behavior such as invasion and metastasis Despite remarkable advances in modern medical sciences, cancer remains a disease difficult to treat and becomes a leading cause of death worldwide (around 13% of all deaths) The progress of nanomedicines and nanomaterials presents promising prospects for cancer treatment, demonstrated by approved therapies such as Doxil, Abraxane, and Ferumoxytol in recent decades. 21 Conventional vs Nanotechnology based Cancer Therapies 22 Cellular Internalization Pathway of Nanocarrier 1) Clathrin-mediated endocytosis :Involves the formation of coated pits on the plasma membrane via interactions of clathrin and adaptor proteins. These pits mature into ∼100 nm coated vesicles (CCVs) that are internalized into early endosomes and subsequently degraded. Efficient for nanoparticles +10 mV), facilitating targeted delivery of molecules like iron, transferrin, LDL receptor, and EGFR. 2) Caveolae-mediated endocytosis: Caveolae are lipid raft- associated invaginations (∼50–80 nm) on the cell membrane, facilitated by caveolin proteins. This pathway is ideal for smaller nanoparticles (250 nm) and is non-specific, facilitating the uptake of diverse cargoes including lipid nanoparticles and pathogens. Macropinosomes undergo maturation into acidic structures (macropino-lysosomes), influencing antigen presentation and immune function in addition to cellular nutrient uptake. 23 Biodegradable Conjugate Polymeric Nanoparticles Amplify Photodynamic Immunotherapy Photodynamic Therapy (PDT) and Its Challenges: PDT is a technique used in cancer treatment where a photosensitizing agent and light are used to generate reactive oxygen species (ROS) that can kill cancer cells. However, one major challenge is that solid tumors often have poor oxygen supply (hypoxia) due to abnormal blood vessels. This low oxygen environment can reduce the effectiveness of PDT and also helps tumors evade the immune system. Vascular Normalization Strategy: Researchers developed a biodegradable polymer-based system (NIR-II bio-degradable pseudo- conjugate polymer, or PSP) carrying regorafenib (Reg) to normalize tumor blood vessels NP-PDT@Reg Nanoparticles: The polymer system (PSP) encapsulates Reg into nanoparticles (NP-PDT@Reg), which release Reg upon exposure to an 808 nm laser (NP-PDT@Reg + L). This triggers vascular normalization, improving oxygen delivery and enhancing NP-PDT@Reg penetration into tumors. Increased ROS production from PDT kills tumor cells while Reg reprograms immune cells from supporting the tumor to attacking it, reversing the tumor's defenses. This approach offers a new way to enhance photodynamic immunotherapy for cancer treatment. Wan, Jia, et al. Advanced Materials 35.31 (2023): 2209799. 24 SMART NANOPARTICLES FOR CANCER THERAPY Smart nanoparticles (NPs) have emerged as a promising alternative to conventional nanoparticles for cancer therapy. Unlike conventional nanoparticles, they can be triggered by specific stimuli and target-specific sites with precise drug delivery. After modification or stimulation by specific factors, smart nanoparticles aggregate at the target site and release their payloads, enabling precise treatment. Their ability to co-deliver therapeutics and diagnostic agents has significantly advanced theranostics for cancer therapy. These nanoparticles exhibit tumor targeting by functionalizing their surface with specific ligands, and they can deliver various drugs, including small molecules, peptides, proteins, nucleic acids, and living cells. Smart nanoparticles can be tailored in size, shape, surface properties, targeting, and composition in response to endogenous and exogenous stimuli. Sun, Leming, et al. "Smart nanoparticles for cancer therapy." Signal transduction and targeted therapy 8.1 (2023): 418. 25 Endogenous and Exogenous stimuli of nanoparticles for cancer therapy Maximizing therapeutic benefits of cancer nanomedicine through the stimuli-triggered dynamic integration of multistage tumor targeting. Either endogenous (changes in pH, redox gradients, enzyme or ATP concentrations) or exogenous (variations in temperature, magnetic fields, ultrasound, or light intensities) stimuli can be utilized to trigger nanoparticle size reduction, charge conversion, as well as ligand exposure, and therefore can dynamically integrate tumor tissue-, cell- and organelle-specific targeting to sequentially achieve high tumor accumulation, deep tumor penetration, efficient cellular internalization, and accurate organelle localization 26 Nanotechnology approaches for infectious diseases Infectious diseases (IDs) represent a persistent global healthcare challenge and are high-priority targets for investigational drugs. The communicable diseases with the highest mortality rates are lower respiratory infections, diarrheal diseases, tuberculosis (TB), HIV infection, and malaria. Effectively managing infectious diseases (IDs) faces numerous challenges, primarily the lack of safe and effective drugs and pathogen resistance, which often necessitates more expensive treatments. Nanotechnology offers transformative potential for detecting and treating diseases, with notable progress in HIV, TB, and malaria treatment, and shows significant impact through mRNA and protein vaccines for SARS-CoV-2. However, transitioning these technologies from lab to clinic remains challenging. Kirtane, Ameya R., et al. "Nanotechnology approaches for global infectious diseases." Nature Nanotechnology 16.4 (2021): 369-384. 27 Application of Nanotechnology in infectious disease: 1) Antimicrobial drug delivery system Nanoparticles (NPs) such as liposomes, dendrimers, and polymeric micelles, inorganic nanoparticles can encapsulate antimicrobial agents and deliver them directly to infected cells, enhancing drug efficacy and reducing side effects. 2) Diagnosis of the pathogens Nanotechnology enhances pathogen detection through highly sensitive and specific diagnostic tools. Gold nanoparticles (AuNPs) and quantum dots (QDs) can be used in biosensors to detect pathogens at very low concentrations. 3) Vaccine production Nanomaterials can significantly improve vaccine efficacy by enhancing the immune response. Nanoparticles like liposomes, polymeric nanoparticles, and virus-like particles (VLPs) serve as effective adjuvants and delivery systems for vaccines. For example, the use of lipid nanoparticles (LNPs) in mRNA COVID-19 vaccines, such as the Pfizer-BioNTech and Moderna vaccines, has shown to elicit strong immune responses. 4) Prevention of the spread of pathogenic microbes Some nanomaterials, such as silver nanoparticles (AgNPs), selenium nanoparticles (SeNPs), and metal NPs solutions can be prepared as environmental sanitizers or as preventive or therapeutic inhalants due to their directive antibacterial or antiviral effects in vitro. Additionally, air filters containing titanium dioxide nanoparticles can degrade airborne pathogens when exposed to UV light, helping to purify the air in enclosed spaces. 5) Therapeutics Nanotechnology can be utilized to modulate the immune response during microbial infections. Nanoparticles can deliver anti-inflammatory drugs or genetic material to immune cells to regulate the immune response. For instance, dendrimers can be engineered to deliver siRNA to macrophages, reducing the production of pro-inflammatory cytokines in conditions like sepsis-repress immunological response due to microbial infection. 28 Nanotechnology based prevention of pathogens Nanotechnology in the prevention of pathogenic bacterial infections, which include killing bacteria in the environment, blocking transmission routes and vaccination Silver Nanoparticles (AgNPs) can release silver ions in the environment, generating ROS that damage the DNA and proteins of bacteria AgNPs can also be applied to masks and disinfectants to prevent the invasion of pathogens and block infection pathways. Nanovaccines can enhance human immunity to improve resistance to infection. Huang, Yujing, et al. "Nanotechnology’s frontier in combatting infectious and inflammatory diseases: prevention and treatment." Signal Transduction and Targeted Therapy 9.1 (2024): 34. 29 Superhydrophobic and Self-Sterilizing Surgical Masks Spray- Coated with Carbon Nanotubes Deactivating SARS-CoV-2 involves subduing its S proteins, which bind to ACE2 receptors, and this can be achieved using the hyperthermal effect of a mask, as the S proteins become inactive at 50°C. Commercial surgical masks exhibit poor hydrophobicity and limited antimicrobial properties. A method to coat single-walled carbon nanotubes (SWCNTs) onto the polypropylene (PP) fibers of surgical masks was developed. The CNT-coated masks show remarkable super-hydrophobicity and photothermal response, with surface temperatures rising above 90°C within 30 seconds of solar illumination, ensuring self-sterilization. Due to the combined super-hydrophobicity and photothermal properties of the SWCNTs, the masks show 99.99% higher bactericidal performance against Escherichia coli compared to pristine masks The scalable spray-coating method makes these nanotube-coated masks a cost-effective solution for personal protection against respiratory diseases. Lee, Sangsu, et al. "Carbon nanotube mask filters and their hydrophobic barrier and hyperthermic antiviral effects on SARS-CoV-2." ACS Applied Nano Materials 4.8 (2021): 8135-8144. 30 Thank you 31