NIH Public Access PDF: The Plasticity of Human Treg and Th17 Cells
Document Details
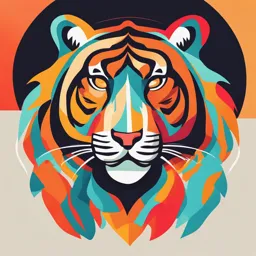
Uploaded by KidFriendlyAstrophysics
Markus Kleinewietfeld and David A. Hafler
Tags
Summary
This article discusses the plasticity of human Treg and Th17 cells, focusing on their role in autoimmune diseases, like multiple sclerosis (MS). It details the recent findings about T cell plasticity with an emphasis on Treg and Th17 cells and their role in human autoimmune disease.
Full Transcript
NIH Public Access Author Manuscript Semin Immunol. Author manuscript; available in PMC 2014 November 15. Published in final edited form as: NIH-PA Author Manuscript...
NIH Public Access Author Manuscript Semin Immunol. Author manuscript; available in PMC 2014 November 15. Published in final edited form as: NIH-PA Author Manuscript Semin Immunol. 2013 November 15; 25(4): 305–312. doi:10.1016/j.smim.2013.10.009. The plasticity of human Treg and Th17 cells and its role in autoimmunity Markus Kleinewietfeld1,2 and David A. Hafler1,2,* 1Departments of Neurology and Immunobiology, Yale School of Medicine, New Haven, CT, United States 2Broad Institute of MIT and Harvard, Boston, MA, United States Abstract CD4+ T helper cells are a central element of the adaptive immune system. They protect the organism against a wide range of pathogens and are able to initiate and control many immune reactions in combination with other cells of the adaptive and the innate immune system. Starting NIH-PA Author Manuscript from a naïve cell, CD4+ T cells can differentiate into various effector cell populations with specialized function. This subset specific differentiation depends on numerous signals and the strength of stimulation. However, recent data have shown that differentiated CD4+ T cell subpopulations display a high grade of plasticity and that their initial differentiation is not an endpoint of T cell development. In particular, FoxP3+ regulatory T cells (Treg) and Th17 effector T cells demonstrate a high grade of plasticity, which allow a functional adaptation to various physiological situations during an immune response. However, the plasticity of Treg and Th17 cells might also be a critical factor for autoimmune disease. Here we discuss the recent developments in CD4+ T cell plasticity with a focus on Treg and Th17 cells and its role in human autoimmune disease, in particular multiple sclerosis (MS). Keywords Autoimmunity; CD4+ T cells; IL-17; Th17; FoxP3; Treg; T cell plasticity 1. Introduction CD4+ T helper (Th) cells are an essential element of the adaptive immune system, regulating NIH-PA Author Manuscript B cell dependent, humoral as well as CD8+ cytotoxic T cell dependent cellular immune responses. Moreover, CD4+ T cells are able to interact with the innate immune system and respond to stimuli in particular from dendritic cells. Upon peptide/MHC-class II TCR mediated antigen encounter, naive CD4+ T cells are activated and differentiate into T effector cells, which can generate long lasting memory T cells. Depending on the antigen © 2013 Elsevier Ltd. All rights reserved. * corresponding author: David A. Hafler, M.D., Gilbert H. Glaser Professor of Neurology and Immunobiology, Chairman, Department of Neurology, Yale School of Medicine, 15 York Street, PO Box 208018, New Haven CT 06520-8018, Phone: 203 785-6351, Fax: 203 785-2238, [email protected]. The authors declare no competing financial interests. Publisher's Disclaimer: This is a PDF file of an unedited manuscript that has been accepted for publication. As a service to our customers we are providing this early version of the manuscript. The manuscript will undergo copyediting, typesetting, and review of the resulting proof before it is published in its final citable form. Please note that during the production process errors may be discovered which could affect the content, and all legal disclaimers that apply to the journal pertain. Kleinewietfeld and Hafler Page 2 and strength of stimulation, cytokine milieu, co-stimulatory and various additional factors, CD4+ T cells can differentiate into distinct subpopulations with specialized functions [1, 2]. NIH-PA Author Manuscript Classically, CD4+ T cells were divided into Th1 and Th2 subsets. Th1 cells produce the signature cytokine interferon (IFN)-γ and are induced in the presence of interleukin (IL)-12. They express the specific transcription factor Tbet (TBX21) and are generated in response to viral infections where they provide help to CD8+ T cells. Th2 cells are primarily linked to humoral immune responses by providing B cell help and are induced in the presence of IL-4. Th2 cells express the transcription factor GATA3 and are characterized by the expression of the signature cytokines IL-4, IL-5, and IL-13. However, since the discovery of Th1 and Th2 cells, several additional Th cell subpopulations have been described. So far, the most prominent additions are the FoxP3+ regulatory T cells (Treg) and IL-17 producing Th17 cells. Regulatory T cells are a distinct lineage of CD4+ T cells, generated during thymic development, which play an important role in maintaining peripheral tolerance. While Th17 cells are induced in the presence of transforming growth factor (TGF)-beta and IL-21, IL-6 or IL-1β and play a major role in fighting extracellular pathogens. Importantly, both of the latter populations are believed to play a major role in human autoimmune diseases [1, 3]. Although CD4+ Th cell polarization based on the Th1/Th2 paradigm was believed to be a stable process with low grade of variability, recent data provided evidence that this is not the case for many Th subpopulations. Indeed, under certain circumstances most of the NIH-PA Author Manuscript differentiated Th cells and in particular Th17 and Treg cells show a great magnitude of plasticity and are able to change their phenotype and function. In this review we discuss the recent findings about Th cell plasticity with an emphasis on Treg and Th17 cells and their role in the human autoimmune disease MS. 2. Human Th17 and Treg cells 2.1 Th17 cells It is now established that Th17 cells represent, in addition to Th1 and Th2 cells, an independent helper T cell lineage [4, 5]. Th17 cells were initially described based on their secretion of IL-17. They express a series of other cytokines including IL-17F, IL-21, GM- CSF and IL-22. Their lineage specific transcription factor is the retinoic acid receptor- related orphan receptor γt (RORγt, in humans RORc), which controls development and function of Th17 cells. However, RORγt acts in concert with other transcription factors such as the RAR-related orphan receptor α (RORα), signal transducer and activator of transcription 3 (STAT3), Basic leucine zipper transcription factor (BATF) and interferon regulatory factor 4 (IRF4) which also have been described to play an important role in Th17 development and function [1, 7–12]. Recently, a comprehensive study defined the NIH-PA Author Manuscript transcription factor dependent network contributing to Th17 generation. Rodent studies helped identify factors involved in the induction of Th17 cells from naïve CD4+ T cells, namely transforming growth factor beta (TGF-β) in combination with IL-6 or IL-21. TGF-β is important for the induction of RORγt in Th17 cells. Studies in the human system also indicated that the cytokines IL-1β and IL-23 play an important role in the induction of human Th17 cells from memory cells [15–17], while TGF-β and IL-21 induce naïve CD4+ cells to become Th17 cells. The cytokine IL-23 is also critical for the inflammatory potential of Th17 cells [18, 19] and is the most important survival factor for Th17 cells. Although, currently no specific cell surface markers for Th17 cells have been identified, studies have demonstrated that Th17 cells highly express the chemokine receptor CCR6 [20–22]. In addition to CCR6 , human Th17 cells also express CD161 and high levels of CD49d, the α-chain of the integrin VLA-4 [24, 25]. Semin Immunol. Author manuscript; available in PMC 2014 November 15. Kleinewietfeld and Hafler Page 3 Th17 cells can be found under homeostatic conditions, particularly in the lamina propria of the small intestine. However, during infection or under inflammatory conditions, Th17 cells are induced in other tissues [26–28]. Based on these and other data, analyzing the antigen NIH-PA Author Manuscript specificity of Th17 cells, the natural role of Th17 cells in the immune system is to protect the host of extracellular bacteria and fungal infections in tissues such as the gut [20, 29]. The physiological role of Th17 cells is more apparent in rare human genetic diseases, which are related to Th17 cells. The autosomal dominant hyper-IgE syndrome (HIES, ‘Job’s syndrome’) is characterized by mutations in the stat3 gene, which lead to the absence of IL-17 production in T cells and severe fungal and bacterial infection [31, 32]. Moreover, patients with Chronic mucocutaneous candidiasis (CMC) suffering from severe candida infection of the skin, nails and mucous membranes, carry a gain of function mutation in stat1 which blocks effective Th17 generation [33, 34]. 2.1.1 The role of Th17 cells in multiple sclerosis—MS is an inflammatory CNS white matter disease where over 100 allelic variants have been identified that, together with a number of environmental factors are associated with the disease. These factors include low vitamin D, smoking, and an increased body mass index. MS is characterized by increases in myelin-antigen reactive T cells, secreting inflammatory cytokines that mediate an attack on the myelin sheaths surrounding axons in the brain and spinal cord. So far, several targets of the immune response have been suggested but the presence of T cells reactive to myelin self-antigens alone is not sufficient for disease to occur. Indeed, T cells NIH-PA Author Manuscript reactive to the same antigens can be found in healthy subjects but various mechanisms are available that control these self-reactive T cells in normal individuals [35–37]. Although Th1 cells were previously thought to drive MS, it now appears that pathogenic Th17 cells play an important role in disease pathogenesis. Based on studies on experimental autoimmune encephalomyelitis (EAE), it became clear that IL-23/Th17 mediated responses are critical for the disease [18, 19]. Of note, recent studies suggested that the cytokine GM- CSF plays a fundamental role in the pathogenicity of Th17 cells in EAE [38, 39]. In line with these murine data, there is also increasing evidence that Th17 cells are critically involved in human MS. Almost a decade before the identification of Th17 cells, increased levels of IL-17 were reported to be associated with disease and several more recent studies have supported a role for pathogenic Th17 cells in MS [35, 41–45]. Moreover, genetic variants associated to the IL-23/Th17 pathway are risk factors for disease. Although not completely understood, one potential mechanism as to how Th17 cells contribute to MS might be the disruption and early penetration of the blood-barrier , potentially by a CCL20-CCR6 guided mechanism through the choroid plexus which then lead to the recruitment, influx and immune activation of other pathogenic cell types [35, 47]. NIH-PA Author Manuscript Recent data indicate that the pathogenicity of Th17 cells, particularly in autoimmune neuro- inflammation, could be directly controlled by environmental factors. The composition of the gut microbiota can greatly impact the host immune system and an imbalance in the gut microbiome could lead to alterations of immune responses both in gut-associated tissues and in the periphery [48, 49]. It was demonstrated that gut residing bacteria such as segmented filamentous bacteria (SFB) can specifically induce Th17 cells. Moreover, luminal ATP, secreted from bacteria was found to indirectly induce Th17 cells. More recently, it was shown that the microbiota could have indeed an impact on the development of EAE [51, 52]. Besides gut bacteria, dietary components itself have been shown to influence the generation of pathogenic Th17 cells. It has long been noted that NaCl-induced hypertonicity can have an impact on immune cells. Moreover, T cells may face different sodium concentrations and hypertonicity in secondary lymphoid tissues and in the interstitium, especially after Semin Immunol. Author manuscript; available in PMC 2014 November 15. Kleinewietfeld and Hafler Page 4 a sodium rich diet [55, 56]. Most recently, it was shown that increased sodium chloride concentrations, similar to concentrations that could be found in interstitial tissues after a high-salt diet [56, 57,] boost the differentiation of Th17 cells in mice and humans. The NIH-PA Author Manuscript high-salt conditions induced a particularly pathogenic phenotype in Th17 cells, with the upregulation of a pro-inflammatory signature characterized by increases in GM-CSF, TNFα, IL-2 and IL-23R expression. Consequently, a high-salt diet led to a severe worsening of EAE, associated with augmented induction of pathogenic Th17 cells in vivo [58, 59]. The effect was dependent on the induction of p38/MAPK, nuclear factor of activated T cells 5 (NFAT5) and serum/glucocorticoid-regulated kinase 1 (SGK1) which in turn regulates indirectly the expression of the IL-23R. Considering the involvement of highly conserved stress induced pathways in this process, the in vivo effect is likely to be more complex involving other cell types, including cells of the innate immune system. Moreover, a high- salt diet may change the composition of the gut microbiota and thus can indirectly affect host immunity as well. However, it remains to be demonstrated whether these environmental factors indeed are contributors to the development of MS and therefore might represent novel environmental risk factors for disease development. 2.2 FoxP3+ regulatory T cells Studies in the past decades have established that regulatory T cells are a central element for NIH-PA Author Manuscript the maintenance of peripheral tolerance. Up to now, several types of regulatory T cells have been identified, which predominantly belong to the CD4+ T cell lineage [3, 60]. Upon activation they are able to neutralize the function of other effector cells, including CD4+ T cells, CD8+ T cells, NK cells, B cells and other antigen presenting cells (APC) [3, 61]. Depending on the type of regulatory T cell, their mechanism of suppression is mainly based on cell/cell contact dependent mechanisms (e.g. CTLA-4, granzymes), the release of suppressive cytokines (e.g. TGF-β or IL-10) or the generation of suppressive metabolites (e.g. adenosine) [3, 60, 61]. The best-characterized regulatory T cell population is the natural regulatory T cell population (Treg or nTreg). Tregs can be identified in humans and rodents by the high expression of the IL-2 receptor alpha chain (CD25) and by the expression of the transcription factor FoxP3 [3, 61, 62]. Tregs are generated in the thymus and are believed to be a specific lineage of T helper cells, imprinted early during development by the expression of FoxP3 in a specific context and by stably induced epigenetic changes [64, 65]. FoxP3 largely controls the phenotype and function of Tregs and mutations in the gene can result in IPEX (Immune dysregulation, Polyendocrinopathy, Enteropathy X-linked syndrome), a severe and rapidly fatal autoimmune disorder. Although it is not NIH-PA Author Manuscript completely understood how precisely regulatory T cells work, there is increasing evidence that FoxP3+ Treg cells are able to suppress ongoing immune reactions using several direct or indirect mechanisms [61, 67, 68]. Interestingly, naïve T cells can also be converted to so- called adaptive or induced Treg (iTreg) in the periphery. This process occurs in the presence of TGF-β or at low dose of antigen encounter, most probably also in the presence of a TGF-β rich or inducing milieu [3, 69]. While adaptive Tregs resemble natural Tregs in phenotype and function there are, however, differences between these cell types most prominently regarding their epigenetic status and stability [65, 70]. Besides TGF-β, which seems to be crucial for the development of Tregs, their survival and homeostasis is dependent on the cytokine IL-2. Recent studies have demonstrated that Tregs can be further subdivided into distinct subsets, partly explaining the functional heterogeneity of Treg cells. Several Treg subsets have been described by differential expression of surface markers such as CD45RA, CD45RO, CD127, Semin Immunol. Author manuscript; available in PMC 2014 November 15. Kleinewietfeld and Hafler Page 5 CCR6, HLA-DR, CD39, CD95, ICOS, CD147 or CD31 [72–78] and can be largely subdivided into memory-like or naïve-like Tregs. Memory-like Tregs are presumably induced from naïve-like Tregs after antigen contact in a specific context and appear to NIH-PA Author Manuscript resemble a Treg population that exerts its suppressive function directly in inflamed tissues. For this reason, they are equipped with homing and chemokine receptors as well as specific effector functions. In contrast, naïve-like Tregs appear to function in secondary lymphoid tissues [3, 79, 80]. This heterogeneous view of Treg populations was recently extended by a series of murine studies that demonstrated the need for Tbet, IRF4, STAT3 or BCL6 induction in Tregs to effectively control Th1, Th2, Th17 or follicular helper cell (TFh) specific effector cell responses. Of note, it remains unclear if analogues to CD4+ effector Th cells, long lasting central-memory type Tregs exist in humans. There are only a few studies, mainly in experimental animal systems, indicating that Tregs or iTregs can also contribute to relatively long lasting immunologic memory [81–83]. 2.2.1 The role of Tregs in Multiple Sclerosis—Based on experimental animal models and studies in human subjects with autoimmune diseases, it became clear that Tregs play a major role in controlling peripheral immune tolerance. Deficits in Treg function appear to be a common cause of human autoimmune diseases. Treg defects have been discovered in patients multiple sclerosis (MS), type I diabetes (T1D), psoriasis, myasthenia gravis and other autoimmune diseases. Similar correlations also have been observed in atopy and allergic diseases [66, 84–87]. There are several observations providing possible NIH-PA Author Manuscript mechanisms, which might contribute to the loss of Treg suppression in MS patients. These mechanisms include for instance a lower thymic Treg output, decreased FoxP3 expression, decreases in CD39 expression, decreased CD58/CD2 dependent co-stimulation or subset- specific changes of Tregs [73, 88–91]. Similar to Th17 cells, Tregs are also influenced by the gut microbiota. Studies in mice have proven a connection of particular gut residing bacteria to the generation of Tregs [92–94]. Of note, the specific induction of IL-10 producing Tregs by a polysaccharide of Bacteroides fragilis was able to protect mice from EAE induction [95, 96]. However, direct evidence that the microbiota may have impact on human Treg generation and a subsequent effect on disease prevention is absent. Nonetheless, recent data using human microbes for the induction of Tregs in murine models of autoimmune diseases show promising results. 2.3 Relationship between Th17 and Treg cells Both Th17 and Tregs are reciprocally related to each other; for example TGF-β links the development of Th17 cells to that of FoxP3+ Tregs. It was shown that TGF-β induces the differentiation of Tregs, whereas the combination of IL-6 or IL-21 results in the induction of NIH-PA Author Manuscript Th17 cells and inhibition of Treg differentiation. On the molecular level it was demonstrated that FoxP3 could bind physically to RORγt and RORα to antagonize each other’s function, which appears to be the basis for their reciprocal relationship [98, 99]. Furthermore, the balance between Th17 and Treg differentiation can be influenced by retinoic acid. In the presence of the vitamin A metabolite, Tregs are preferentially induced over Th17 cells, since retinoic acid enhances TGF-β signaling while it blocks the expression of the IL-6 receptor. Additionally, the aryl hydrocarbon receptor (AHR) that is highly expressed on Tregs and Th17 cells, can promote the induction of both cell types by integrating environmental stimuli Thus, depending on the ligand, AHR mediated signaling results in either Treg or Th17 cell differentiation [101, 102]. Another recent example is the balanced regulation of Th17 and Treg development based on metabolic inputs signaled by the transcription factor hypoxia inducible factor 1α (HIF1α). HIF1α can bind to and enhance RORγt expression, while inhibiting FoxP3 expression and thereby promote Th17 differentiation over Treg development [103–105]. Moreover, the close relation between Semin Immunol. Author manuscript; available in PMC 2014 November 15. Kleinewietfeld and Hafler Page 6 Tregs and Th17 cells is also evident in a rodent obesity model, where probiotic bacteria shifted the balance from a pro-inflammatory, IL-17 dominated immune response towards an anti-inflammatory, IL-10 and Treg dependent response. NIH-PA Author Manuscript These examples underline the close relationship between both cell types and indicate that there is a particular need for a balanced regulation of both subsets in many immunological settings. Although its in vivo relevance for the human immune system is not clear yet, several mechanism have apparently evolved, which can, depending on the surrounding signals, regulate the immune response in the direction of either suppression or inflammation. 3. Plasticity and functional variability of Th17 and Treg cells in relation to MS 3.1 Th17 plasticity It was noted from early on that at least a subset of Th17 cells had the potential to secrete IFN-γ in mice and humans and thus identify a population with Th1-like features. This was surprising, since initial studies have found that IFN-γ can block Th17 development. By using reporter mice for IL-17F this phenomenon was studied in more detail in vitro and in vivo and it was found that the Th17 stability is dependent on TGF-β and IL-23 but is lost in the presence of IL-12, favoring the expression of IFN-γ in line with the roles of Tbet and STAT4. This flexibility of Th17 cells is underlined by the epigenetic status NIH-PA Author Manuscript of these cells. Th17 cells presented for instance a semi-permissive/bivalent histone modification status of the Tbx21 gene. The development of a transgenic system to map the fate of IL-17 expressing cells further demonstrated the in vivo plasticity of murine Th17 cells. Strikingly, as demonstrated in the EAE model, almost all of the pathogenic cells in the spinal cord that expressed IFN-γ or other pro-inflammatory cytokines were derived from IL-23 dependent Th17 cells. Evidence from repertoire analyses of human T cells suggests that a similar mechanism exists in humans and it was proposed that these cells could be distinguished from Th1 cells by the expression of CD161. Moreover, these observations are in line with the high frequency of IFN-γ and IL-17 co-expressing cells in human memory Th17 cells [21, 25, 43]. Thus, the flexibility and plasticity of Th17 cells to gain a Th1-type phenotype is highly dependent on the cytokine micro-environment and inflammatory situation and the pathogenicity of Th17 cells in autoimmune diseases seems to be related to this phenotype. Of note, studies in the EAE model indicated that only Th17 generated in the presence of particular stimuli and cytokines adopt a pathogenic phenotype and gain the ability to induce disease. It was for instance shown that the cytokines TGF-β3 and IL-23 play a key role in this process [113, 114]. NIH-PA Author Manuscript A similar differential Th17 cell induction into a pathogenic or an anti-inflammatory phenotype was described in humans by using different antigenic stimuli. Staphylococcus aureus-specific Th17 cells were induced to produce the anti-inflammatory cytokine IL-10, whereas Candida albicans-specific Th17 cells displayed a more pathogenic phenotype with the induced expression of IFN-γ. This phenomenon was dependent on C. albicans triggered IL-1β secretion of monocytes. In line with these data, the induction of IL-10 expression by Th17 cells in the small intestine with an immune suppressive capacity was also described in mice. It was suggested that this process could serve as a control mechanism for excessive inflammation. Analogous to the Th17/Th1 plasticity, recent data indicated that Th17 cells can also acquire a Th2-type phenotype and potentially play a role in asthma. Interestingly, a study using a helminthic infection model demonstrated the in vivo conversion of Th17 cells into IL-4 expressing Th2-type cells, which might be a partial explanation for the inverse Semin Immunol. Author manuscript; available in PMC 2014 November 15. Kleinewietfeld and Hafler Page 7 correlation of helminth infection and protection from autoimmunity. However, the information on Th17/Th2 plasticity needs more investigation (Figure 1). NIH-PA Author Manuscript 3.2 Plasticity of Treg cells Recent findings indicate that there is an unexpected plasticity between both Tregs and T effector cells. Although information on this phenomenon is still limited there are indications that this plasticity might play a key role in the control of the immune system, enabling a rapid switch from suppression to active immunity. Moreover, this phenomenon might be a critical factor for the development of autoimmune diseases. Since the conversion of Treg cells, enriched for autoreactive TCR specificities into pathogenic effector T cells could be an in particular harmful threat to the host , this process must be tightly regulated on several levels. 3.2.1 Treg-Th1 plasticity—The potential of Tregs to convert into Th1-like cells, co- expressing FoxP3 and Tbet was first described in mice almost a decade ago. Several other studies made similar observations, mainly in murine in vivo models of lymphophenia or inflammation [121–124]. Direct evidence that this population was related to natural Tregs come from experiments where the in vivo deletion of FoxP3 led to the development of pro- inflammatory IFN-γ secreting cells, which retained self antigen specificity. The phenomenon of Tbet induction in Tregs was also observed in models of infection and colitis and it was noted that this in vivo plasticity was highly dependent on IL-12. This NIH-PA Author Manuscript data indicated that the conversion to pathogenic Th1-like Treg cells can occur in vivo and thus can have the potential to induce autoimmunity. This data is also supported by the epigenetic regulation of Th1-associated loci in Treg cells. Although a pro-inflammatory cytokine expression profile in human CD25+ Tregs was noted from early on , these data were complicated due to the absence of valid Treg markers and thus by potential contaminations of effector T cells in Treg preparations. The presence of IFN-γ producing cells was also observed in FoxP3 expressing cells after PMA/ionomycin stimulation or after prolonged in vitro expansion of FoxP3+ Tregs [128, 129]. However, since FoxP3 can be also transiently upregulated on human effector T cells , it was unclear if the IFN-γ producing cells derived from Tregs or contaminating effector T cells. Nonetheless, using a more detailed analysis, it has been shown that a subset of human natural FoxP3+ Tregs is capable of inducing Tbet and IFN-γ expression. Of note, IFN- γ producing Treg displayed an almost fully demethylated TSRD region in the FoxP3 locus, similar to IFN-γ negative Treg, which was indicative of a functional nTreg. The IFN-γ production correlated with the upregulation of Tbet, CXCR3 and other classical Th1 marker and could be induced in vitro by IL-12 and IL-2. Moreover, the suppressive activity of this NIH-PA Author Manuscript population was dramatically decreased in an IFN-γ dependent fashion (Figure 1). Importantly, untreated patients with relapsing remitting MS (RRMS) displayed significantly increased levels of IFN-γ secreting Th1-like Treg in peripheral blood and it is likely that this, at least in part, contributes to the observed loss of suppression in MS patients. Interestingly, a similar population could be observed in humans with T1D , indicating that IL-12 dependent Th1-like Tregs are associated with several autoimmune diseases in humans. It remains to be seen, if the Th1-Treg plasticity plays a role for disease pathogenesis. Moreover, the question whether this Treg subset has a physiological role in non-autoimmune conditions remains open. 3.2.2 Treg-Th17 plasticity—Apart from the reciprocal relationship of Th17 and Treg cells (see 2.3), plasticity has been observed between both antagonistic cell types [133–136]. This phenomenon was first reported in mice, where it was shown that IL-6 was able to induce Th17 producing cells from FoxP3+ Treg in the presence of TGF-β. Other Semin Immunol. Author manuscript; available in PMC 2014 November 15. Kleinewietfeld and Hafler Page 8 groups reported similar findings, although based on different stimuli and models [135, 136]. The study by Osorio et al. showed that the conversion seems to be dependent on RORγt expression in a subset of murine FoxP3+ Tregs that can be modulated to express IL-17 by NIH-PA Author Manuscript dendritic cells (DC). Yang et al. demonstrated that this phenomenon partly depends on IL-6 and IL-1β mediated signals affecting STAT3, RORγt and RORα function. Another study analyzed this process in vivo and demonstrated the potential pathogenicity of converted Tregs in murine models of T1D. Most recent data indicated the additional importance of RUNX transcription factors for the induction of a Th17-like phenotype. A similar phenomenon was reported for human FoxP3+ Treg cells. IL-17 expression in ex vivo stimulated human FoxP3+ Tregs was found to be mainly restricted to a subset of CCR6+ and CD49d+ cells and the in vitro conversion to Th17-like Tregs was dependent on the presence of IL-1β and on epigenetic modifications [24, 134, 138]. More recent studies confirmed these observations and demonstrated additionally a correlation of the Th17-like phenotype with RORc expression, although it’s expression was not restricted to IL-17+ FoxP3+ cells [139, 140]. Interestingly, in contrast to Th1-like Tregs, IL-17 secreting Tregs were still suppressive in vitro, but lost their suppressive capacity rapidly upon strong activation in the presence of IL-1β and IL-6 (Figure 1). Another mechanism of Treg- Th17 conversion might occur upon microbial stimuli via TLR2 ligation. Thus, besides the reciprocal relationship in the differentiation of Treg and Th17 cells, there also seem to exist mechanisms that allow the rapid shut down of suppression and the induction of pro- NIH-PA Author Manuscript inflammatory responses in Treg cells. The likely occurrence of a Th17-like conversion in vivo was recently proposed for tumor infiltrating Tregs isolated from human ovarian tumors and in murine models of cancer, where the conversion to Th17-like Tregs was indirectly dependent on indoleamine 2,3-dioxygenase (IDO). Of note, the expression of CD39 on a subset of human Tregs might enable them to effectively self-control the induction of Th17-plasticity and Th17 cells per se. Extracellular ATP is considered as classical ‘danger-signal’ that can induce inflammasome activation and IL-1β release from innate immune cells and thus creates the environment for an efficient induction of the Th17 conversion and de novo induction. As mentioned above, luminal ATP released from certain bacteria have been shown to be critical for Th17 generation. Thus, CD39 activity on Tregs might control this process through the removal of extracellular ATP [73, 144] (Figure 1). This is in line with the observation that CD39+ Tregs are most effective in controlling Th17 cells , moreover a similar mechanism was recently proposed for the effects of IL-27 on DC mediated suppression of Th17 responses and EAE. In summary, although the information on the relevance of Th17-like Tregs for autoimmune disease is still sparse, the subset was recently associated with psoriasis [146, 147], NIH-PA Author Manuscript autoimmune hepatitis [148, 149] and systemic sclerosis , while so far no direct association could be detected in MS. Thus, It will be an important issue for future studies to clarify the role of this Treg subset in autoimmune diseases. 4. Concluding remarks The plasticity of CD4+ T cells and in particular of Tregs and Th17 cells might have evolved to keep elasticity in combination with stability to enable the immune system to most flexibly deal with pathogens. The plasticity of CD4+ cells enables the immune system to quickly react to changes in the environment and pathogens. However, this flexibility also comprises a potential threat to the host, since the deregulation of this system enhances the risk of developing autoimmunity. It is therefore not surprising that several mechanisms have evolved to control T cell plasticity and many of them are related to interfaces of mucosal tissues where changes in the environment are most critical. Semin Immunol. Author manuscript; available in PMC 2014 November 15. Kleinewietfeld and Hafler Page 9 Thus, factors that regulate Treg and Th17 plasticity would be targets for immune therapies aiming at the manipulation of the immune system in settings of cancer or autoimmune diseases. In this context, the manipulation of the gut microbiota or related factors might have NIH-PA Author Manuscript the most favorable potential for being therapeutically used in autoimmune diseases. Promising data from animal studies has already demonstrated that it could influence Th17 and Treg homeostasis and actively induce immune suppression via the induction of Tregs but also through the conversion of Th17 cells into non-pathogenic phenotypes. In summary, the information on the phenomena of CD4+ T cell plasticity and its relation to human autoimmune disease and cancer is still limited. However, future research applying most up-to-date techniques (e.g. humanized mouse models), under consideration of genetic and environmental factors in human research, are likely to quickly gain more insight into this field to ultimately develop new therapeutic approaches for the treatment of human diseases. Acknowledgments This work was supported by a National MS Society Collaborative Research Center Award CA1061-A-18, National Institutes of Health Grants P01 AI045757, U19 AI046130, U19 AI070352, and P01 AI039671, and by a Jacob Javits Merit award (NS2427) from the National Institute of Neurological Disorders and Stroke, the Penates Foundation and the Nancy Taylor Foundation for Chronic Diseases, Inc. (to D.A.H.). The authors would like to thank S. Ni Choileain for critical reading of the manuscript. NIH-PA Author Manuscript References 1. Korn T, Bettelli E, Oukka M, Kuchroo VK. IL-17 and Th17 Cells. Annual review of immunology. 2009; 27:485–517. 2. Vahedi G, ACP, Hand TW, Laurence A, Kanno Y, O’Shea JJ, et al. Helper T-cell identity and evolution of differential transcriptomes and epigenomes. Immunological reviews. 2013; 252:24–40. [PubMed: 23405893] 3. Sakaguchi S, Yamaguchi T, Nomura T, Ono M. Regulatory T cells and immune tolerance. Cell. 2008; 133:775–787. [PubMed: 18510923] 4. Park H, Li Z, Yang XO, Chang SH, Nurieva R, Wang YH, et al. A distinct lineage of CD4 T cells regulates tissue inflammation by producing interleukin 17. Nature immunology. 2005; 6:1133– 1141. [PubMed: 16200068] 5. Harrington LE, Hatton RD, Mangan PR, Turner H, Murphy TL, Murphy KM, et al. Interleukin 17- producing CD4+ effector T cells develop via a lineage distinct from the T helper type 1 and 2 lineages. Nature immunology. 2005; 6:1123–1132. [PubMed: 16200070] 6. Ivanov, McKenzie BS, Zhou L, Tadokoro CE, Lepelley A, Lafaille JJ, et al. The orphan nuclear receptor RORgammat directs the differentiation program of proinflammatory IL-17+ T helper cells. NIH-PA Author Manuscript Cell. 2006; 126:1121–1133. [PubMed: 16990136] 7. Bettelli E, Korn T, Kuchroo VK. Th17: the third member of the effector T cell trilogy. Current opinion in immunology. 2007; 19:652–657. [PubMed: 17766098] 8. Dong C. TH17 cells in development: an updated view of their molecular identity and genetic programming. Nature reviews Immunology. 2008; 8:337–348. 9. Schraml BU, Hildner K, Ise W, Lee WL, Smith WA, Solomon B, et al. The AP-1 transcription factor Batf controls T(H)17 differentiation. Nature. 2009; 460:405–409. [PubMed: 19578362] 10. Yang XO, Pappu BP, Nurieva R, Akimzhanov A, Kang HS, Chung Y, et al. T helper 17 lineage differentiation is programmed by orphan nuclear receptors ROR alpha and ROR gamma. Immunity. 2008; 28:29–39. [PubMed: 18164222] 11. Huber M, Brustle A, Reinhard K, Guralnik A, Walter G, Mahiny A, et al. IRF4 is essential for IL-21-mediated induction, amplification, and stabilization of the Th17 phenotype. Proceedings of the National Academy of Sciences of the United States of America. 2008; 105:20846–20851. [PubMed: 19088203] Semin Immunol. Author manuscript; available in PMC 2014 November 15. Kleinewietfeld and Hafler Page 10 12. Chung Y, Chang SH, Martinez GJ, Yang XO, Nurieva R, Kang HS, et al. Critical regulation of early Th17 cell differentiation by interleukin-1 signaling. Immunity. 2009; 30:576–587. [PubMed: 19362022] NIH-PA Author Manuscript 13. Ciofani M, Madar A, Galan C, Sellars M, Mace K, Pauli F, et al. A validated regulatory network for Th17 cell specification. Cell. 2012; 151:289–303. [PubMed: 23021777] 14. Bettelli E, Korn T, Oukka M, Kuchroo VK. Induction and effector functions of T(H)17 cells. Nature. 2008; 453:1051–1057. [PubMed: 18563156] 15. Wilson NJ, Boniface K, Chan JR, McKenzie BS, Blumenschein WM, Mattson JD, et al. Development, cytokine profile and function of human interleukin 17-producing helper T cells. Nature immunology. 2007; 8:950–957. [PubMed: 17676044] 16. Acosta-Rodriguez EV, Napolitani G, Lanzavecchia A, Sallusto F. Interleukins 1[beta] and 6 but not transforming growth factor-[beta] are essential for the differentiation of interleukin 17- producing human T helper cells. Nature immunology. 2007; 8:942–949. [PubMed: 17676045] 17. Yang L, Anderson DE, Baecher-Allan C, Hastings WD, Bettelli E, Oukka M, et al. IL-21 and TGF-beta are required for differentiation of human T(H)17 cells. Nature. 2008; 454:350–352. [PubMed: 18469800] 18. Cua DJ, Sherlock J, Chen Y, Murphy CA, Joyce B, Seymour B, et al. Interleukin-23 rather than interleukin-12 is the critical cytokine for autoimmune inflammation of the brain. Nature. 2003; 421:744–748. [PubMed: 12610626] 19. Langrish CL, Chen Y, Blumenschein WM, Mattson J, Basham B, Sedgwick JD, et al. IL-23 drives a pathogenic T cell population that induces autoimmune inflammation. The Journal of NIH-PA Author Manuscript experimental medicine. 2005; 201:233–240. [PubMed: 15657292] 20. Acosta-Rodriguez EV, Rivino L, Geginat J, Jarrossay D, Gattorno M, Lanzavecchia A, et al. Surface phenotype and antigenic specificity of human interleukin 17-producing T helper memory cells. Nature immunology. 2007; 8:639–646. [PubMed: 17486092] 21. Annunziato F, Cosmi L, Santarlasci V, Maggi L, Liotta F, Mazzinghi B, et al. Phenotypic and functional features of human Th17 cells. The Journal of experimental medicine. 2007; 204:1849– 1861. [PubMed: 17635957] 22. Hirota K, Yoshitomi H, Hashimoto M, Maeda S, Teradaira S, Sugimoto N, et al. Preferential recruitment of CCR6-expressing Th17 cells to inflamed joints via CCL20 in rheumatoid arthritis and its animal model. The Journal of experimental medicine. 2007; 204:2803–2812. [PubMed: 18025126] 23. Annunziato F, Cosmi L, Liotta F, Maggi E, Romagnani S. The phenotype of human Th17 cells and their precursors, the cytokines that mediate their differentiation and the role of Th17 cells in inflammation. International immunology. 2008; 20:1361–1368. [PubMed: 18820263] 24. Kleinewietfeld M, Starke M, Di Mitri D, Borsellino G, Battistini L, Rotzschke O, et al. CD49d provides access to “untouched” human Foxp3+ Treg free of contaminating effector cells. Blood. 2009; 113:827–836. [PubMed: 18941119] 25. Brucklacher-Waldert V, Steinbach K, Lioznov M, Kolster M, Holscher C, Tolosa E. Phenotypical characterization of human Th17 cells unambiguously identified by surface IL-17A expression. J NIH-PA Author Manuscript Immunol. 2009; 183:5494–5501. [PubMed: 19843935] 26. Ivanov, Atarashi K, Manel N, Brodie EL, Shima T, Karaoz U, et al. Induction of intestinal Th17 cells by segmented filamentous bacteria. Cell. 2009; 139:485–498. [PubMed: 19836068] 27. Pappu R, Rutz S, Ouyang W. Regulation of epithelial immunity by IL-17 family cytokines. Trends in immunology. 2012; 33:343–349. [PubMed: 22476048] 28. Ouyang W, Kolls JK, Zheng Y. The biological functions of T helper 17 cell effector cytokines in inflammation. Immunity. 2008; 28:454–467. [PubMed: 18400188] 29. Iwakura Y, Ishigame H, Saijo S, Nakae S. Functional specialization of interleukin-17 family members. Immunity. 2011; 34:149–162. [PubMed: 21349428] 30. Puel A, Cypowyj S, Marodi L, Abel L, Picard C, Casanova JL. Inborn errors of human IL-17 immunity underlie chronic mucocutaneous candidiasis. Current opinion in allergy and clinical immunology. 2012; 12:616–622. [PubMed: 23026768] Semin Immunol. Author manuscript; available in PMC 2014 November 15. Kleinewietfeld and Hafler Page 11 31. Milner JD, Brenchley JM, Laurence A, Freeman AF, Hill BJ, Elias KM, et al. Impaired T(H)17 cell differentiation in subjects with autosomal dominant hyper-IgE syndrome. Nature. 2008; 452:773–776. [PubMed: 18337720] NIH-PA Author Manuscript 32. Ma CS, Chew GY, Simpson N, Priyadarshi A, Wong M, Grimbacher B, et al. Deficiency of Th17 cells in hyper IgE syndrome due to mutations in STAT3. The Journal of experimental medicine. 2008; 205:1551–1557. [PubMed: 18591410] 33. Liu L, Okada S, Kong XF, Kreins AY, Cypowyj S, Abhyankar A, et al. Gain-of-function human STAT1 mutations impair IL-17 immunity and underlie chronic mucocutaneous candidiasis. The Journal of experimental medicine. 2011; 208:1635–1648. [PubMed: 21727188] 34. van de Veerdonk FL, Plantinga TS, Hoischen A, Smeekens SP, Joosten LA, Gilissen C, et al. STAT1 mutations in autosomal dominant chronic mucocutaneous candidiasis. The New England journal of medicine. 2011; 365:54–61. [PubMed: 21714643] 35. Nylander A, Hafler DA. Multiple sclerosis. The Journal of clinical investigation. 2012; 122:1180– 1188. [PubMed: 22466660] 36. Greer JM, Pender MP. Myelin proteolipid protein: an effective autoantigen and target of autoimmunity in multiple sclerosis. Journal of autoimmunity. 2008; 31:281–287. [PubMed: 18502611] 37. Raddassi K, Kent SC, Yang J, Bourcier K, Bradshaw EM, Seyfert-Margolis V, et al. Increased frequencies of myelin oligodendrocyte glycoprotein/MHC class II-binding CD4 cells in patients with multiple sclerosis. J Immunol. 2011; 187:1039–1046. [PubMed: 21653833] 38. Codarri L, Gyulveszi G, Tosevski V, Hesske L, Fontana A, Magnenat L, et al. RORgammat drives NIH-PA Author Manuscript production of the cytokine GM-CSF in helper T cells, which is essential for the effector phase of autoimmune neuroinflammation. Nature immunology. 2011; 12:560–567. [PubMed: 21516112] 39. El-Behi M, Ciric B, Dai H, Yan Y, Cullimore M, Safavi F, et al. The encephalitogenicity of T(H)17 cells is dependent on IL-1- and IL-23-induced production of the cytokine GM-CSF. Nature immunology. 2011; 12:568–575. [PubMed: 21516111] 40. Matusevicius D, Kivisakk P, He B, Kostulas N, Ozenci V, Fredrikson S, et al. Interleukin-17 mRNA expression in blood and CSF mononuclear cells is augmented in multiple sclerosis. Mult Scler. 1999; 5:101–104. [PubMed: 10335518] 41. Kebir H, Kreymborg K, Ifergan I, Dodelet-Devillers A, Cayrol R, Bernard M, et al. Human TH17 lymphocytes promote blood-brain barrier disruption and central nervous system inflammation. Nature medicine. 2007; 13:1173–1175. 42. Han MH, Hwang SI, Roy DB, Lundgren DH, Price JV, Ousman SS, et al. Proteomic analysis of active multiple sclerosis lesions reveals therapeutic targets. Nature. 2008; 451:1076–1081. [PubMed: 18278032] 43. Brucklacher-Waldert V, Stuerner K, Kolster M, Wolthausen J, Tolosa E. Phenotypical and functional characterization of T helper 17 cells in multiple sclerosis. Brain: a journal of neurology. 2009; 132:3329–3341. [PubMed: 19933767] 44. Li Y, Wang H, Long Y, Lu Z, Hu X. Increased memory Th17 cells in patients with neuromyelitis optica and multiple sclerosis. Journal of neuroimmunology. 2011; 234:155–160. [PubMed: NIH-PA Author Manuscript 21489641] 45. Tesmer LA, Lundy SK, Sarkar S, Fox DA. Th17 cells in human disease. Immunological reviews. 2008; 223:87–113. [PubMed: 18613831] 46. Reboldi A, Coisne C, Baumjohann D, Benvenuto F, Bottinelli D, Lira S, et al. C-C chemokine receptor 6-regulated entry of TH-17 cells into the CNS through the choroid plexus is required for the initiation of EAE. Nature immunology. 2009; 10:514–523. [PubMed: 19305396] 47. Zepp J, Wu L, Li X. IL-17 receptor signaling and T helper 17-mediated autoimmune demyelinating disease. Trends in immunology. 2011; 32:232–239. [PubMed: 21493143] 48. Littman DR, Rudensky AY. Th17 and regulatory T cells in mediating and restraining inflammation. Cell. 2010; 140:845–858. [PubMed: 20303875] 49. Round JL, Mazmanian SK. The gut microbiota shapes intestinal immune responses during health and disease. Nature reviews Immunology. 2009; 9:313–323. 50. Atarashi K, Nishimura J, Shima T, Umesaki Y, Yamamoto M, Onoue M, et al. ATP drives lamina propria TH17 cell differentiation. Nature. 2008; 455:808–812. [PubMed: 18716618] Semin Immunol. Author manuscript; available in PMC 2014 November 15. Kleinewietfeld and Hafler Page 12 51. Lee YK, Menezes JS, Umesaki Y, Mazmanian SK. Proinflammatory T-cell responses to gut microbiota promote experimental autoimmune encephalomyelitis. Proceedings of the National Academy of Sciences of the United States of America. 2011; 108 (Suppl 1):4615–4622. [PubMed: NIH-PA Author Manuscript 20660719] 52. Berer K, Mues M, Koutrolos M, Rasbi ZA, Boziki M, Johner C, et al. Commensal microbiota and myelin autoantigen cooperate to trigger autoimmune demyelination. Nature. 2011; 479:538–541. [PubMed: 22031325] 53. Junger WG, Liu FC, Loomis WH, Hoyt DB. Hypertonic saline enhances cellular immune function. Circ Shock. 1994; 42:190–196. [PubMed: 8055665] 54. Go WY, Liu X, Roti MA, Liu F, Ho SN. NFAT5/TonEBP mutant mice define osmotic stress as a critical feature of the lymphoid microenvironment. Proceedings of the National Academy of Sciences of the United States of America. 2004; 101:10673–10678. [PubMed: 15247420] 55. Machnik A, Neuhofer W, Jantsch J, Dahlmann A, Tammela T, Machura K, et al. Macrophages regulate salt-dependent volume and blood pressure by a vascular endothelial growth factor-C- dependent buffering mechanism. Nature medicine. 2009; 15:545–552. 56. Wiig H, Schroder A, Neuhofer W, Jantsch J, Kopp C, Karlsen TV, et al. Immune cells control skin lymphatic electrolyte homeostasis and blood pressure. The Journal of clinical investigation. 2013; 123:2803–2815. [PubMed: 23722907] 57. Machnik A, Dahlmann A, Kopp C, Goss J, Wagner H, van Rooijen N, et al. Mononuclear phagocyte system depletion blocks interstitial tonicity-responsive enhancer binding protein/ vascular endothelial growth factor C expression and induces salt-sensitive hypertension in rats. Hypertension. 2010; 55:755–761. [PubMed: 20142563] NIH-PA Author Manuscript 58. Kleinewietfeld M, Manzel A, Titze J, Kvakan H, Yosef N, Linker RA, et al. Sodium chloride drives autoimmune disease by the induction of pathogenic TH17 cells. Nature. 2013; 496:518– 522. [PubMed: 23467095] 59. Wu C, Yosef N, Thalhamer T, Zhu C, Xiao S, Kishi Y, et al. Induction of pathogenic TH17 cells by inducible salt-sensing kinase SGK1. Nature. 2013; 496:513–517. [PubMed: 23467085] 60. Roncarolo MG, Gregori S, Battaglia M, Bacchetta R, Fleischhauer K, Levings MK. Interleukin-10- secreting type 1 regulatory T cells in rodents and humans. Immunological reviews. 2006; 212:28– 50. [PubMed: 16903904] 61. Sakaguchi S, Miyara M, Costantino CM, Hafler DA. FOXP3+ regulatory T cells in the human immune system. Nature reviews Immunology. 2010; 10:490–500. 62. Baecher-Allan C, Brown JA, Freeman GJ, Hafler DA. CD4+CD25high regulatory cells in human peripheral blood. J Immunol. 2001; 167:1245–1253. [PubMed: 11466340] 63. Fontenot JD, Rudensky AY. A well adapted regulatory contrivance: regulatory T cell development and the forkhead family transcription factor Foxp3. Nature immunology. 2005; 6:331–337. [PubMed: 15785758] 64. Baron U, Floess S, Wieczorek G, Baumann K, Grutzkau A, Dong J, et al. DNA demethylation in the human FOXP3 locus discriminates regulatory T cells from activated FOXP3(+) conventional T cells. European journal of immunology. 2007; 37:2378–2389. [PubMed: 17694575] NIH-PA Author Manuscript 65. Ohkura N, Kitagawa Y, Sakaguchi S. Development and maintenance of regulatory T cells. Immunity. 2013; 38:414–423. [PubMed: 23521883] 66. Randolph DA, Fathman CG. Cd4+Cd25+ regulatory T cells and their therapeutic potential. Annu Rev Med. 2006; 57:381–402. [PubMed: 16409156] 67. Vignali DAA, Collison LW, Workman CJ. How regulatory T cells work. Nature Reviews Immunology. 2008; 8:523–532. 68. Tang QZ, Bluestone JA. The Foxp3(+) regulatory T cell: a jack of all trades, master of regulation. Nature immunology. 2008; 9:239–244. [PubMed: 18285775] 69. Bach JF. Regulatory T cells under scrutiny. Nature reviews Immunology. 2003; 3:189–198. 70. Horwitz DA, Zheng SG, Gray JD. Natural and TGF-beta-induced Foxp3(+)CD4(+) CD25(+) regulatory T cells are not mirror images of each other. Trends in immunology. 2008; 29:429–435. [PubMed: 18676178] 71. Zheng Y, Rudensky AY. Foxp3 in control of the regulatory T cell lineage. Nature immunology. 2007; 8:457–462. [PubMed: 17440451] Semin Immunol. Author manuscript; available in PMC 2014 November 15. Kleinewietfeld and Hafler Page 13 72. Kleinewietfeld M, Puentes F, Borsellino G, Battistini L, Rotzschke O, Falk K. CCR6 expression defines regulatory effector/memory-like cells within the CD25(+)CD4+ T-cell subset. Blood. 2005; 105:2877–2886. [PubMed: 15613550] NIH-PA Author Manuscript 73. Borsellino G, Kleinewietfeld M, Di Mitri D, Sternjak A, Diamantini A, Giometto R, et al. Expression of ectonucleotidase CD39 by Foxp3+ Treg cells: hydrolysis of extracellular ATP and immune suppression. Blood. 2007; 110:1225–1232. [PubMed: 17449799] 74. Baecher-Allan C, Wolf E, Hafler DA. MHC class II expression identifies functionally distinct human regulatory T cells. J Immunol. 2006; 176:4622–4631. [PubMed: 16585553] 75. Haas J, Fritzsching B, Trubswetter P, Korporal M, Milkova L, Fritz B, et al. Prevalence of newly generated naive regulatory T cells (Treg) is critical for Treg suppressive function and determines Treg dysfunction in multiple sclerosis. J Immunol. 2007; 179:1322–1330. [PubMed: 17617625] 76. Fritzsching B, Oberle N, Pauly E, Geffers R, Buer J, Poschl J, et al. Naive regulatory T cells: a novel subpopulation defined by resistance toward CD95L-mediated cell death. Blood. 2006; 108:3371–3378. [PubMed: 16868256] 77. Solstad T, Bains SJ, Landskron J, Aandahl EM, Thiede B, Tasken K, et al. CD147 (Basigin/ Emmprin) identifies FoxP3+CD45RO+CTLA4+-activated human regulatory T cells. Blood. 2011; 118:5141–5151. [PubMed: 21937704] 78. Ito T, Hanabuchi S, Wang YH, Park WR, Arima K, Bover L, et al. Two functional subsets of FOXP3+ regulatory T cells in human thymus and periphery. Immunity. 2008; 28:870–880. [PubMed: 18513999] 79. Huehn J, Siegmund K, Hamann A. Migration rules: functional properties of naive and effector/ NIH-PA Author Manuscript memory-like regulatory T cell subsets. Curr Top Microbiol Immunol. 2005; 293:89–114. [PubMed: 15981477] 80. Huehn J, Hamann A. Homing to suppress: address codes for Treg migration. Trends in immunology. 2005; 26:632–636. [PubMed: 16243583] 81. Rosenblum MD, Gratz IK, Paw JS, Lee K, Marshak-Rothstein A, Abbas AK. Response to self antigen imprints regulatory memory in tissues. Nature. 2011; 480:538–542. [PubMed: 22121024] 82. Gratz IK, Rosenblum MD, Abbas AK. The life of regulatory T cells. Annals of the New York Academy of Sciences. 2013; 1283:8–12. [PubMed: 23402657] 83. Apostolou I, von Boehmer H. In vivo instruction of suppressor commitment in naive T cells. The Journal of experimental medicine. 2004; 199:1401–1408. [PubMed: 15148338] 84. Ling EM, Smith T, Nguyen XD, Pridgeon C, Dallman M, Arbery J, et al. Relation of CD4+CD25+ regulatory T-cell suppression of allergen-driven T-cell activation to atopic status and expression of allergic disease. Lancet. 2004; 363:608–615. [PubMed: 14987885] 85. Baecher-Allan C, Hafler DA. Human regulatory T cells and their role in autoimmune disease. Immunological reviews. 2006; 212:203–216. [PubMed: 16903916] 86. Viglietta V, Baecher-Allan C, Weiner HL, Hafler DA. Loss of functional suppression by CD4+CD25+ regulatory T cells in patients with multiple sclerosis. The Journal of experimental medicine. 2004; 199:971–979. [PubMed: 15067033] NIH-PA Author Manuscript 87. Haas J, Hug A, Viehover A, Fritzsching B, Falk CS, Filser A, et al. Reduced suppressive effect of CD4+CD25high regulatory T cells on the T cell immune response against myelin oligodendrocyte glycoprotein in patients with multiple sclerosis. European journal of immunology. 2005; 35:3343– 3352. [PubMed: 16206232] 88. Fletcher JM, Lonergan R, Costelloe L, Kinsella K, Moran B, O’Farrelly C, et al. CD39+Foxp3+ regulatory T Cells suppress pathogenic Th17 cells and are impaired in multiple sclerosis. J Immunol. 2009; 183:7602–7610. [PubMed: 19917691] 89. De Jager PL, Baecher-Allan C, Maier LM, Arthur AT, Ottoboni L, Barcellos L, et al. The role of the CD58 locus in multiple sclerosis. Proceedings of the National Academy of Sciences of the United States of America. 2009; 106:5264–5269. [PubMed: 19237575] 90. Baecher-Allan CM, Costantino CM, Cvetanovich GL, Ashley CW, Beriou G, Dominguez-Villar M, et al. CD2 costimulation reveals defective activity by human CD4+CD25(hi) regulatory cells in patients with multiple sclerosis. J Immunol. 2011; 186:3317–3326. [PubMed: 21300823] 91. Lowther DE, Hafler DA. Regulatory T cells in the central nervous system. Immunological reviews. 2012; 248:156–169. [PubMed: 22725960] Semin Immunol. Author manuscript; available in PMC 2014 November 15. Kleinewietfeld and Hafler Page 14 92. Mazmanian SK, Round JL, Kasper DL. A microbial symbiosis factor prevents intestinal inflammatory disease. Nature. 2008; 453:620–625. [PubMed: 18509436] 93. Round JL, Mazmanian SK. Inducible Foxp3+ regulatory T-cell development by a commensal NIH-PA Author Manuscript bacterium of the intestinal microbiota. Proceedings of the National Academy of Sciences of the United States of America. 2010; 107:12204–12209. [PubMed: 20566854] 94. Atarashi K, Tanoue T, Shima T, Imaoka A, Kuwahara T, Momose Y, et al. Induction of colonic regulatory T cells by indigenous Clostridium species. Science. 2011; 331:337–341. [PubMed: 21205640] 95. Ochoa-Reparaz J, Mielcarz DW, Wang Y, Begum-Haque S, Dasgupta S, Kasper DL, et al. A polysaccharide from the human commensal Bacteroides fragilis protects against CNS demyelinating disease. Mucosal immunology. 2010; 3:487–495. [PubMed: 20531465] 96. Ochoa-Reparaz J, Mielcarz DW, Ditrio LE, Burroughs AR, Begum-Haque S, Dasgupta S, et al. Central nervous system demyelinating disease protection by the human commensal Bacteroides fragilis depends on polysaccharide A expression. J Immunol. 2010; 185:4101–4108. [PubMed: 20817872] 97. Atarashi K, Tanoue T, Oshima K, Suda W, Nagano Y, Nishikawa H, et al. Treg induction by a rationally selected mixture of Clostridia strains from the human microbiota. Nature. 2013; 500:232–236. [PubMed: 23842501] 98. Zhou L, Lopes JE, Chong MM, Ivanov, Min R, Victora GD, et al. TGF-beta-induced Foxp3 inhibits T(H)17 cell differentiation by antagonizing RORgammat function. Nature. 2008; 453:236–240. [PubMed: 18368049] NIH-PA Author Manuscript 99. Du J, Huang C, Zhou B, Ziegler SF. Isoform-specific inhibition of ROR alpha-mediated transcriptional activation by human FOXP3. J Immunol. 2008; 180:4785–4792. [PubMed: 18354202] 100. Mucida D, Park Y, Kim G, Turovskaya O, Scott I, Kronenberg M, et al. Reciprocal TH17 and regulatory T cell differentiation mediated by retinoic acid. Science. 2007; 317:256–260. [PubMed: 17569825] 101. Veldhoen M, Hirota K, Westendorf AM, Buer J, Dumoutier L, Renauld JC, et al. The aryl hydrocarbon receptor links TH17-cell-mediated autoimmunity to environmental toxins. Nature. 2008; 453:106–109. [PubMed: 18362914] 102. Quintana FJ, Basso AS, Iglesias AH, Korn T, Farez MF, Bettelli E, et al. Control of Treg and TH17 cell differentiation by the aryl hydrocarbon receptor. Nature. 2008; 453:65–71. [PubMed: 18362915] 103. Dang EV, Barbi J, Yang HY, Jinasena D, Yu H, Zheng Y, et al. Control of T(H)17/T(reg) balance by hypoxia-inducible factor 1. Cell. 2011; 146:772–784. [PubMed: 21871655] 104. Shi LZ, Wang R, Huang G, Vogel P, Neale G, Green DR, et al. HIF1alpha-dependent glycolytic pathway orchestrates a metabolic checkpoint for the differentiation of TH17 and Treg cells. The Journal of experimental medicine. 2011; 208:1367–1376. [PubMed: 21708926] 105. Tsun A, Chen Z, Li B. Romance of the three kingdoms: RORgammat allies with HIF1alpha against FoxP3 in regulating T cell metabolism and differentiation. Protein & cell. 2011; 2:778– NIH-PA Author Manuscript 781. [PubMed: 22058032] 106. Poutahidis T, Kleinewietfeld M, Smillie C, Levkovich T, Perrotta A, Bhela S, et al. Microbial reprogramming inhibits Western diet-associated obesity. PloS one. 2013; 8:e68596. [PubMed: 23874682] 107. Harrington LE, Mangan PR, Weaver CT. Expanding the effector CD4 T-cell repertoire: the Th17 lineage. Current opinion in immunology. 2006; 18:349–356. [PubMed: 16616472] 108. Lee YK, Turner H, Maynard CL, Oliver JR, Chen D, Elson CO, et al. Late developmental plasticity in the T helper 17 lineage. Immunity. 2009; 30:92–107. [PubMed: 19119024] 109. Wei G, Wei L, Zhu J, Zang C, Hu-Li J, Yao Z, et al. Global mapping of H3K4me3 and H3K27me3 reveals specificity and plasticity in lineage fate determination of differentiating CD4+ T cells. Immunity. 2009; 30:155–167. [PubMed: 19144320] 110. Hirota K, Duarte JH, Veldhoen M, Hornsby E, Li Y, Cua DJ, et al. Fate mapping of IL-17- producing T cells in inflammatory responses. Nature immunology. 2011; 12:255–263. [PubMed: 21278737] Semin Immunol. Author manuscript; available in PMC 2014 November 15. Kleinewietfeld and Hafler Page 15 111. Annunziato F, Cosmi L, Liotta F, Maggi E, Romagnani S. Defining the human T helper 17 cell phenotype. Trends in immunology. 2012; 33:505–512. [PubMed: 22682163] 112. Maggi L, Santarlasci V, Capone M, Rossi MC, Querci V, Mazzoni A, et al. Distinctive features of NIH-PA Author Manuscript classic and nonclassic (Th17 derived) human Th1 cells. European journal of immunology. 2012; 42:3180–3188. [PubMed: 22965818] 113. Ghoreschi K, Laurence A, Yang XP, Tato CM, McGeachy MJ, Konkel JE, et al. Generation of pathogenic T(H)17 cells in the absence of TGF-beta signalling. Nature. 2010; 467:967–971. [PubMed: 20962846] 114. Lee Y, Awasthi A, Yosef N, Quintana FJ, Xiao S, Peters A, et al. Induction and molecular signature of pathogenic TH17 cells. Nature immunology. 2012; 13:991–999. [PubMed: 22961052] 115. Zielinski CE, Mele F, Aschenbrenner D, Jarrossay D, Ronchi F, Gattorno M, et al. Pathogen- induced human TH17 cells produce IFN-gamma or IL-10 and are regulated by IL-1beta. Nature. 2012; 484:514–518. [PubMed: 22466287] 116. Esplugues E, Huber S, Gagliani N, Hauser AE, Town T, Wan YY, et al. Control of TH17 cells occurs in the small intestine. Nature. 2011; 475:514–518. [PubMed: 21765430] 117. Panzer M, Sitte S, Wirth S, Drexler I, Sparwasser T, Voehringer D. Rapid in vivo conversion of effector T cells into Th2 cells during helminth infection. J Immunol. 2012; 188:615–623. [PubMed: 22156341] 118. Hirahara K, Poholek A, Vahedi G, Laurence A, Kanno Y, Milner JD, et al. Mechanisms underlying helper T-cell plasticity: implications for immune-mediated disease. The Journal of NIH-PA Author Manuscript allergy and clinical immunology. 2013; 131:1276–1287. [PubMed: 23622118] 119. Zhou X, Bailey-Bucktrout S, Jeker LT, Bluestone JA. Plasticity of CD4(+) FoxP3(+) T cells. Current opinion in immunology. 2009; 21:281–285. [PubMed: 19500966] 120. Stock P, Akbari O, Berry G, Freeman GJ, Dekruyff RH, Umetsu DT. Induction of T helper type 1-like regulatory cells that express Foxp3 and protect against airway hyper-reactivity. Nature immunology. 2004; 5:1149–1156. [PubMed: 15448689] 121. Wan YY, Flavell RA. Regulatory T-cell functions are subverted and converted owing to attenuated Foxp3 expression. Nature. 2007; 445:766–770. [PubMed: 17220876] 122. Williams LM, Rudensky AY. Maintenance of the Foxp3-dependent developmental program in mature regulatory T cells requires continued expression of Foxp3. Nature immunology. 2007; 8:277–284. [PubMed: 17220892] 123. Duarte JH, Zelenay S, Bergman ML, Martins AC, Demengeot J. Natural Treg cells spontaneously differentiate into pathogenic helper cells in lymphopenic conditions. European journal of immunology. 2009; 39:948–955. [PubMed: 19291701] 124. Zhou X, Bailey-Bucktrout SL, Jeker LT, Penaranda C, Martinez-Llordella M, Ashby M, et al. Instability of the transcription factor Foxp3 leads to the generation of pathogenic memory T cells in vivo. Nature immunology. 2009; 10:1000–1007. [PubMed: 19633673] 125. Oldenhove G, Bouladoux N, Wohlfert EA, Hall JA, Chou D, Dos Santos L, et al. Decrease of Foxp3+ Treg cell number and acquisition of effector cell phenotype during lethal infection. NIH-PA Author Manuscript Immunity. 2009; 31:772–786. [PubMed: 19896394] 126. Feng T, Cao AT, Weaver CT, Elson CO, Cong Y. Interleukin-12 converts Foxp3+ regulatory T