Multicompartment Models PDF
Document Details
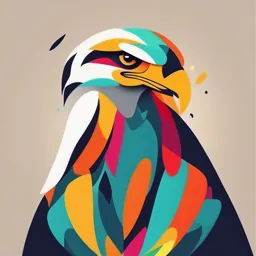
Uploaded by IndulgentChaparral
Sultan Qaboos University Hospital
Tags
Summary
These notes provide an overview of multicompartment models, specifically focusing on the two-compartment open model. They cover concepts such as body tissue categorization, drug distribution, and elimination. It also touches on pharmacokinetic parameters and clinical applications.
Full Transcript
OBJECTIVES To understand kinetic analysis of a multicompartment model. To MULTICOMPARTMENT MODELS Winter, Part1 TWO COMPARTMENT OPEN MODEL Most common model Body tissues two broad categories: Central Compartment: Group of tissues which equilibrate instantaneously are supposed to re...
OBJECTIVES To understand kinetic analysis of a multicompartment model. To MULTICOMPARTMENT MODELS Winter, Part1 TWO COMPARTMENT OPEN MODEL Most common model Body tissues two broad categories: Central Compartment: Group of tissues which equilibrate instantaneously are supposed to reside in central compartment. Sampled compartment ( though such sampling is not always necessary). know various Distribution factors. To learn more about Two Compartment Open Model. Highly liver, perfused tissues : lungs, kidney etc. Peripheral Contains or Tissue Compartment: slowly equilibrating tissues Drug requires some length of time for equilibration. This model assumes Drug eliminated from compartment. central Possible to have Part of an organ in the central compartment and Rest in the tissue compartment. Determining factor for classification Rate of equilibration. Categorization of two compartment model Depending upon the compartment from which the drug is eliminated TWO COMPARTMENT OPEN MODEL: INTRAVENOUS INJECTION (I.V. BOLUS ADMINISTRATION) 1.Two compartment model with elimination from central compartment 2.Two compartment model with elimination from peripheral compartment 3.Two compartment model with elimination from both the compartments. Drug that follows two compartment kinetics After an intravenous injection Decline in plasma conc. : Biexponential Two disposition processes Distribution and Elimination Rapid decline due to Distribution into more slowly perfused tissues. Initial rapid decline in the central compartment distribution phase sometime, pseudo-distribution equilibrium achieved between two compartments of the curve. After State of equilibrium between central compartment and more poorly perfused tissue compartment. After this equilibrium is established, loss of drug from central compartment appears to be single first-order process It is due to overall processes of elimination of drug from the body. The second, slower rate process elimination phase. Two compartment model assumes t = 0 there is no drug in the tissue compartment. Tissue drug level curve after a single intravenous dose of drug shown in fig. The tissue drug level will eventually peak start to decline as conc. gradient between two compartment narrows. Theoretical tissue conc. together with the blood conc., IDEA OF : total amount of drug remaining in the body at any time. This information would not be available without using pharmacokinetic models. Samples of blood removed from central compartment - analyzed for presence of drug. Distribution Phase Elimination phase Distribution phase may take minutes or hours may be missed entirely if blood is sampled too late after administration of the drug. In the model depicted above K12 and K21 : first order rate constants Depict drug transfer between central and peripheral compartments. Let the subscript c and p define central and peripheral compartment respectively. Relationship between amount of drug in each compartment and the conc. of drug in each compartment : Xc Cc = ---------(2) Vc Xp Cp = ---------(3) Vp Where Xc = Amount of drug in the central compartment Xp = Amount of drug in the peripheral compartment The rate of drug change in tissues : d Cp ---------- = K12 Cc - K21 Cp dt (1) = Volume of drug in the central compartment Vp = Volume of drug in the peripheral compartment Eqn 1 becomes Rate d Cp ---------- = K12 Xc / Vc - K21 Xp / Vp dt Vc (4) of change in drug conc. in central compartment : d Cc ---------- = K21 Cp - K12 Cc - KeCc dt (5) d Cc ---------- = K21 Xp / Vp - K12 Xc / Vc - Ke Xc / Vc dt ( 6) PHARMACOKINETIC PARAMETERS Single compartment pharmacokinetic calculations relatively simple. Situations HAVING two, and occasionally more than two compartments drug distribution, elimination and pharmacologic effect. First compartment smaller, rapidly equilibrating volume, usually made up of plasma or blood and those organs or tissues that have high blood flow are in rapid equilibrium with the blood or plasma drug conc. First compartment volume : Vi or initial volume of distribution. Second compartment Equilibrates over a somewhat longer period. This volume referred to as V t or tissue volume of distribution. OBJECTIVES To study Effects of a Two – Compartment Model on the loading dose and plasma conc.( C ) To learn Consequences of an inaccurate prediction To learn how the problems can be circumvented Half life for distribution phase alpha half life Half life for drug elimination beta half life. Sum of Vi and Vt : apparent volume of distribution (V). Drugs are assumed to enter into and be eliminated from Vi. Any drug that distributes into tissue compartment (Vt ) must reequilibrate into Vi before it can be eliminated. Effects of a Two – Compartment Model on the loading dose and plasma conc.( C ) Some time required for a drug to distribute into Vt, Rapidly administered loading dose calculated on the basis of V ( Vi+ Vt) Consequences of Result in an initial C higher than predicted Why ? initial volume of distribution (Vi) is always smaller than V. In these instances when loading doses are calculated based on the total volume of distribution, conc. of drug delivered to the target organs could be much higher than expected and produce toxicity if loading dose is not administered appropriately. an inaccurate prediction depend on Whether target organ behaves as though it were located in Vi or Vt. Examples: Lidocaine, Phenobarbital, procainamide, and theophylline exert therapeutic and toxic effects on target organs that behave as though they are located in Vi. Problem can First be circumvented by calculating loading dose based on the total volume of distribution ( V ), Then administering the loading dose at a rate slow enough to allow for drug distribution into Vt. This approach is common in clinical practice, Guidelines for rates of drug administration are often based on the principle of two-compartment modeling with Receptors for clinical response ( toxic or therapeutic) responding as though they were located in Vi. Second approach To administer loading dose in sufficiently small individual bolus doses such that C in Vi does not exceed some predetermined critical conc. Potassium is a good example of a drug that follows this principle of two-compartment modeling with the end-organ being located in Vi. When Potassium is primarily an intracellular electrolyte Its cardiac effects parallel the plasma conc. In addition, there is slow equilibrium between plasma and tissue potassium concs. potassium is given intravenously rate of administration must be carefully controlled serious cardiac toxicity and death will occur if patient experiences excessive plasma (Vi) concs. Concept of two compartment modeling also important in evaluating the offset of drug effect. For drugs with end organ for clinical response located in Vi Rapid achievement of a therapeutic response Followed quickly by loss of therapeutic response may be the result of drug being distributed into larger volume of distribution rather than drug being eliminated from the body. When the drug’s target organ is in second or tissue compartment, Vt e.g. digoxin, lithium high C, which may be observed before distribution occurs, is not dangerous. However plasma concs that are obtained before distribution is complete will not reflect the tissue conc. at equilibrium. This These plasma samples cannot be used to predict therapeutic or toxic potential of these drugs. Clinicians usually wait for 1-3 hours after an intravenous bolus dose of digoxin before evaluating the effect delay allows Digoxin to distribute to site of action (myocardium) so that the full therapeutic or toxic effects of a dose can be observed. Slow drug distribution into the tissue compartment can pose problems in accurate interpretation of drug conc. when a drug is given by intravenous route. These Generally not a problem when a drug is given orally. Rate of absorption is usually slower than the rate of distribution from Vi to Vt. Digoxin and lithium are exceptions to this rule. For these two drugs Receptors in end-organs behave as though they are located in more slowly equilibrating tissue compartment Vt. drugs given orally: several hours required for complete absorption and distribution. Digoxin Plasma samples obtained less than 6 hours after an oral dose lithium oral dose of lithium less than 12 hours : questionable value. Plasma concs obtained during the distribution phase ( before equilibrium with the deep tissue compartment is complete) will be increased, Pharmacologic response will be much less than the plasma concs would indicate. DRUGS WITH SIGNIFICANT AND NONOBJECTIVES To learn about drugs with significant and non-significant two compartment modeling. To understand the drugs that border on having significant two compartment modeling means If patient not harmed by initially elevated drug conc. in alpha phase and no drug samples are taken in alpha phase, Then Drug can be successfully modeled as one-compartment drug (i.e only the elimination or beta phase is considered). SIGNIFICANT TWO COMPARTMENT MODELING Alpha phase for most drugs represents distribution of drug from Vi into Vt Relatively little drug eliminated during distribution phase. Drugs that behave in this way are generally referred to as nonsignificant two compartment drugs. Non-significant For some drugs, Increased drug plasma concs during the alpha phase can be clinically significant Why? serious toxicity If end organ behaves as though it lies within the initial volume of distribution (Vi) Drugs These drugs considered to exhibit “nonsignificant ” two compartment modeling only after alpha phase or distribution has been completed. Plasma samples obtained for pharmacokinetic modeling only during beta or elimination phase. Drugs that border on having significant two compartment modeling lithium and lidocaine. When a one-compartment model is used for drugs that exhibit significant drug elimination in the alpha phase, Actual trough concs will be lower than those predicted by onecompartment model. with “ significant ” two compartment modeling Those eliminated to significant extent during initial alpha phase. Example: Methotrexate alpha phase cannot be thought of simply as distribution, Why? Significant elimination occurs as well. Two-compartment computer models are available for therapeutic drug monitoring. If care taken to avoid obtaining samples in distribution phase, Very similar pharmacokinetic interpretations are usually arrived at using simpler one-compartment model. LEARNING OUTCOMES At the end of the chapter the student will be able to: Adjust the administration of doses depending on the target organs location. To distinguish between significant and insignificant compartment modelling.