MSCI Principles 1 & 2 - Marine Science Fall 2024 - PDF
Document Details
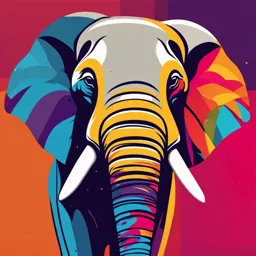
Uploaded by AffableLeibniz
Connections Academy
2024
Mrs. Hunter
Tags
Summary
These notes cover the introductory concepts of Marine Science, also known as Oceanography. They focus on the structure and properties of water, including salinity and geologic features of ocean basins. The material is likely intended for an undergraduate-level course, and is a summary of the first two principles from the Fall 2024 semester.
Full Transcript
Marine Science Fall 2024 - Mrs. Hunter Let's go! Introduction What is Marine Science? Marine Science is the process of discovering facts, processes and unifying principles that explains the nature of oceans and their associated life forms. Oceanography is another name for Marine Sci...
Marine Science Fall 2024 - Mrs. Hunter Let's go! Introduction What is Marine Science? Marine Science is the process of discovering facts, processes and unifying principles that explains the nature of oceans and their associated life forms. Oceanography is another name for Marine Science. Essentially, it is the study of the oceans and seas. Start Course Principle 1 Structure and Properties Salts and Salinity of Water Geologic Features Ocean Circulation Sea Level Principle 2 Structure and Properties of Water Structure of Water Water is made up of two elements: Hydrogen Oxygen The chemical symbol for water is H2O Water is a polar molecule because it This means that each has an uneven distribution of molecule of water is charges composed of two hydrogen The oxygen atom attracts the atoms held together by a shared electrons close towards covalent bond (sharing its large nucleus – making it electrons). slightly more negative (-) The two hydrogen atoms have more protons than electrons – making them slightly positive (+) Like dissolves like examples. De nitions Hydrogen Bonds Water Molecules form Hydrogen Bonds Water’s polarity allows it to bond with many other molecules – including itself. Hydrogen Bonds form between water molecules because the slightly positive hydrogen end of one water molecule is attracted to the slightly negative oxygen end of another water molecule Hydrogen bonds are much weaker than covalent bonds. De nitions Chemical Oceanography - the study of elements, compounds, chemical reactions that occur in sea water Structure and Properties of Properties of Water Water Due to its polarity, water has special properties: Water as a liquid Cohesion/Adhesion Viscosity Surface Tension Ice Floats Structure and Properties of Properties of Water Water Cohesion Surface Tension Water molecules stick to each other Adhesion The polar nature of water allows it to form a “skin” Water sticks to other Caused by hydrogen bonds materials holding the water molecules Water molecules are together attracted to positive and Many smaller animals use negative forces of other surface tension and weight substances distribution to “walk on water” Structure and Properties of Ice Floats? Water As water cools enough to turn from a liquid to a solid, the hydrogen bonds spread the molecules into a crystal structure that takes up more space than liquid water With more volume, ice is less dense than liquid water so it oats. This property has a huge effect on Earth By oating, ice insulates the water below, allowing to retain heat and remain a liquid – if ice sank the oceans would be entirely frozen or at least much colder – this would drastically change the Earth’s climate Structure and Properties of Water as a Solvent Water Salt dissolves in water due to Universal Solvent water’s polarity Water’s polarity pulls Substances that do not separate into ions apart/dissociates the salt and can still dissolve in water through other crystals (NaCl) mechanisms (sugar in water) In the process, the Because so many substances dissolve in dissociated sodium and water, it is known as the “universal solvent” chloride become charged A few substances do NOT dissolve in water… particles/ions and are Non-polar substances like oil do not attracted to the positive dissolve in water hydrogen atoms and the negative oxygen atoms of water molecules. These bonds tend to keep salt in the solution. Salts and Salinity De nitions and Why are the Properties of Determining oceans salty? Seawater What salts are found in the Learn more about the De ne and nd salinity, ocean, where is it coming properties of seawater. temperature, and depth from, and is the ocean getting saltier? Salts and Salinity - De nitions and Determining Salts and Salinity Salinity: The total concentration of all dissolved inorganic solids (ions) in seawater. Salinity is expressed in parts per thousand (‰) because even very small variations are signi cant To convert parts per thousand into percent you divide by 10, so How do scientists measure that 35 ‰ = 3.5% Ocean Salinity salinity? Methods vary but usually involve the conduction of Major Sea Salts electricity Major Sea Salts Only six elements and compounds comprise about 99% of sea salts: chlorine (Cl-) sodium (Na+) sulfur (SO4-2) magnesium (Mg+2) calcium (Ca+2) potassium (K+) The chlorine ion makes up 55% of the salt in seawater. Salts and Salinity - De nitions and Determining CTD Determining Salinity, Temperature, and Depth Scientists measure salinity, temperature, and depth using special instruments and procedures: Salinometer: Determines the electrical conductivity of water Conductivity, Temperature, and Depth Sensor (CTD): Sensor that can be attached to a submersible or deployed by itself to pro le temperature, depth and salinity. Data are transmitted to a ship/vessel Temperature and salinity are used to determine density Salts and Salinity - Why are the oceans salty? Salinity of Ocean Water This map shows the average salinity in the various areas of Earth's oceans. Salts and Salinity - Why are the oceans salty? Why are the Are the oceans oceans salty? ge ing saltier? Weathering/Erosion Evaporation Hydrothermal Vents Biological Processes Volcanic Activity Salts and Salinity - Why are the oceans salty? Salinity, Temperature, and Water Density Most of the ocean’s surface has an average salinity of 3.5% Waves, tides, and currents mix Rainwater and water owing from freshwater waters of varying salinity and rivers lowers salinity while evaporation make them more uniform – so, increases salinity even surface salinity varies with Salinity and temperature also vary by depth the season, weather (especially Density differences cause water to rainfall and evaporation), and separate into layers location High density layers lie beneath lower density layers Warmer, lower density surface waters are separated from cool, high density deep waters by the thermocline Salts and Salinity - Properties of Seawater The Properties of Seawater are: Raised Boiling Decreased Heat Point Capacity The boiling point of seawater is It takes less heat to raise the slightly higher than pure fresh Electrical temperature of seawater than water. Conductivity to raise freshwater Salts act like conductors and Decreased conduct electricity Slowed Freezing Temp Evaporation The freezing point of seawater The attraction between salt is slightly lower than that of ions and water keeps seawater pure fresh water from evaporating as fast as freshwater Salts and Salinity - Properties of Seawater Acidity and Alkalinity Pure seawater has a pH of 7 (neutral) Typical seawater has a pH range of 7.8 - 8.3 Carbon dioxide in seawater acts as a buffer and prevents changes in the pH of the ocean. Geologic Features Geologic Features Ocean basins are composed of the sea floor and all of its geological features; and vary in size, shape, and features due to the movement of Earth's crust (the lithosphere). Geologic Features Layers of the Earth The Core: Inner Core Made up of iron (90%) and nickel with Theorized to be solid due to intense some silicon, sulfur, and other heavy pressure elements New evidence suggests that the Temperature of 5,500 °C (9032 °F) inner core may get as hot as The total core (inner and outer 6,600°C (11,912°F) at its center together) have an estimated radius of hotter than the surface of the sun 3470km Outer Core 31.5% of the Earth’s mass and 16% of Less pressure than inner core it is the Earth’s volume theorized to be a dense liquid Mantle Crust Geologic Features The Mantle Contains silicon and oxygen with some iron and magnesium Estimated to be about 2,900km thick Made up of the upper and lower mantle 68% of the Earth’s mass and 83% of the Earth’s volume Temperature range between 500 to 900 °C (932 to 1,652 °F) at the upper boundary with the crust; to over 4,000°C (7,230 °F) at the boundary with the core. Geologic Features The Mantle Upper Mantle Made up of two layers 1. Asthenosphere asthenes =weak): The layer that is partially melted slowly owing magma below the lithosphere extending to a depth of 350- 650km 2. Lithosphere ( lithos =rock): Earth’s cool rigid outer layer that is 100-200km thick Made up of the rigid solid upper portion of the mantel and the crust Lower Mantle Extends to the core More dense and ows more slowly. Geologic Features The Crust Thin solid outermost layer of the Earth Relatively cool temperature 0.4% of Earth’s mass and 1% of Earth’s volume Composed of oxygen, silicon, magnesium, and iron. The temperature of the crust increases with depth, the range from about 200°C (392°F) to 400°C (752°F) Oceanic Crust Thin and made up of mostly basalt (heavy dark colored) denser than granite Continental Crust Thicker and made up of mostly granite (light colored rock) Geologic Features Theory of Continental Dri In 1912, a 32-year-old German scientist named Alfred Wegner proposed the theory of continental drift, which explains how continents move over time. Wegner theorized that continents have the ability to plow through oceans and collide into each other. Wegner speculated that all of Earth’s continents were once joined in one single landmass, which he named Pangea. Meet Alfred Wegner Wegner rst proposed the theory of continental drift in 1912. Like many scientists before him, when looking at a map of the world, he noticed how the eastern coast of South America looked like a puzzle piece that t perfectly into the western Coast of Africa. Take a look at a map. Can you see the connection, too? This, combined with the fact that fossils of the same ancient plants and animals had been found on different continents, intrigued him. For example, fossils of a reptile known as Mesosaurus have only been found in South America and in southern Africa. This was an odd discovery because Mesosaurus were fresh-water reptiles, which means there is no way they could have swum across the Atlantic Ocean from South America to Africa! The Cynognathus was another ancient animal whose fossils were found in both South America and Africa. The Cynognathus was strictly a land-dwelling, mammal-like reptile, so the idea of this animal’s crossing of the Atlantic was even more outrageous. Fossilized tropical plants were also found in Antarctica. The equator has always been the hottest and most tropical area of Earth. Therefore, it was perplexing to nd tropical plants in one of the coldest climates on Earth. In addition, the Appalachian Mountains of eastern North America appeared to link to the mountains of the Scottish Highlands as they were composed of the same ancient sediment, and the ancient rock layers of both ranges were identical. If you were Alfred Wegner and you were confronted with all this scienti c data, what conclusion would you draw? Geologic Features Pangea This singular landmass, commonly referred to as the supercontinent, existed on Earth for approximately 100 million years. Pangea is believed to have formed 200–300 million years ago from the collision of the ancient continents of Gondwana—which comprised what we now know as South America, India, Africa, Australia, and Antarctica—and Euramerica, which was a combination of North America and Europe. Eventually, other smaller continents like Angaran, which included what is now Siberia, made their way to the giant supercontinent, forming a single landmass with a single ocean known as Panthalassa. Pangea started to break up about 200 million years ago, eventually splitting into the continents we now know. Scientists believe this happened because of rift zones. Rift zones are areas where the Earth’s plates move away from one another, leaving room for magma from Earth’s mantle to rise to the surface, cool, and solidify, in turn creating new oceanic or continental crust. The idea of Pangea explains how Mesosaurus and Cynognathus fossils were found both in South America and Africa and how tropical plants could have once survived in Antarctica. Geologic Features Wegner's Biggest Theoretical Flaw While Wegner’s theory explained the data, it did have one major aw: He could not explain how or why the continents moved. So, the majority of the scienti c community rejected Wegner’s theory because he could not explain the mechanisms that drove continental drift. Did the continents follow a pattern? Was the movement gradual or abrupt? Did something pull or push the continents together? Will it happen again? While Wegner attempted to explain the phenomenon by suggesting the rotation of the Earth played a role, there was no evidence to reinforce this explanation. Alfred Wegner was on to something when he developed the theory of continental drift, but he didn’t quite have all the pieces in place. However, his work still inspired scientists after him to build on his theory as they explored how ancient land masses may have moved. After Wegner’s death in 1930, technological advances increased rapidly. New technology helped scientists explore the ocean oor, which revealed that geological features like volcanoes, mountain ranges, and earthquakes were found on distinct lines around the world! These lines would later be de ned as the edges of tectonic plates. A tectonic plate is an incredibly large piece of solid rock that is composed of the crust and upper mantle of the Earth. This revelation provided data that rekindled the scienti c community’s interest in Wegner’s theory. Geologic Features Geologic Features Geologic Features Geologic Features Geologic Features Geologic Features Geologic Features Plate Tectonics The lithosphere is broken up into 7 major plates and many minor plates that are constantly being recycled. Geologic Features Geologic Features Geologic Features Geologic Features Geologic Features Geologic Features Geologic Features Geologic Features Features of the Sea Floor Continental Margin Continental Margin - the area where continental crust meets oceanic crust. The margin consists of the continental shelf, the continental slope, the continental rise, and the abyssal plain. All of these features characterize how continental crust transforms into oceanic crust. Continental Shelf - part of the continent that extends into ocean about 200km and is really part of the continent even though it is under water. The shallow, submerged edge of the continent; made of granitic continental crust; area where rivers transport sediments to the shore. Continental Slope - the steep slope at edge of the continental shelf that drops off sharply. The transition between the gently descending continental shelf (granite) and the deep-ocean floor (basalt); true edge of continent. Continental Rise - gentle transition between continental slope and abyssal plain; accumulated sediments found at the base of the continental slope. Ocean Basin - consists of ridges, Ocean Basin trenches, abyssal plain, abyssal hill, volcanic islands, seamounts, Features guyots, and atolls. Continental Margin Continental Margin is the area where continental crust meets oceanic crust. The margin consists of the continental shelf, the continental slope, the continental rise, and the abyssal plain. All of these features characterize how continental crust transforms into oceanic crust. There are two types of continental margins: active - In an active continental margin, the boundary between land and sea is also a tectonic plate boundary. An example of this is the West Coast of the United States, where the Paci c Plate and the North American Plate meet. Passive - A passive continental margin occurs where the boundary between land and sea is not associated with a tectonic plate boundary. An example of this is the East Coast of the United States, where the plate boundary between the North American Plate and the Eurasian Plate do not meet until the Mid-Atlantic Ridge, far from the coastline. You’ll nd much less geological activity happening at passive continental margins, which is why the East Coast tends to have fewer earthquakes and volcanoes than the West Coast. Ocean Basin Features Ridges- underwater mountain trains Trenches Abyssal Plain- at plain that extends seaward from the base of the continental slope Abyssal Hill- hills less than 1000m high on the abyssal plain (most are volcanic in nature) Volcanic Islands Seamounts- Steep sided volcanoes on abyssal plain rising abruptly and sometimes breaking the surface to become islands Guyots- Submerged at topped seamounts Atolls- When a seamount disappears below the surface and the coral reef is left as a ring Geologic Features Continental Margins Submarine Canyons - feature of some continental margins. Formed when earthquakes cause underwater avalanches of sediments (turbidity currents). Geologic Features Geologic Features Geologic Features Geologic Features Geologic Features Geologic Features An oceanic ridge is a mountainous chain of young, basaltic rock at an active spreading center of an ocean. Oceanic ridges are not always at the center of ocean basins – less than 60% Ridges canʼt expand evenly on the surface of a sphere, so the lines are irregular, marked by transform faults Geologic Features Geologic Features Geologic Features Geologic Features Geologic Features Geologic Features Seamount Seamounts move with the lithosphere outward and downward away from spreading centers. They are isolated mountains. Not attached to an ocean ridge. Geologic Features Geologic Features Trenches Trenches are arc-shaped depressions in the ocean floor caused by the subduction of a converging ocean plate. They are located in the deepest parts of the ocean. Geologic Features Geologic Features Island Arcs Island Arcs, chains of volcanic islands and seamounts, are usually found parallel to the edges of ocean trenches. Material melts as the plate sinks and magma rises to the surface Great earthquakes that cause tsunamis are also associated with trenches. (left) As two oceanic plates converge, an island arc is formed by volcanic activity. Ocean Circulation Ocean Circulation Ocean water is in constant motion. We will look at the role currents, the water cycle, waves, and tides play in ocean circulation. Ocean Circulation Waves Tides Water Cycle Waves are a disturbance of Tides are the periodic rise Currents water that transfer a large and fall of surface water Water circulates Water circulates between amount of energy over a level. throughout the ocean due the ocean and atmosphere long distance, but with to wind-driven and through the water cycle. very little horizontal density-driven currents. movement of water. Waves Waves When at the beach, you will see waves crash along the shore. Waves not only help shape our coastline, but they move enormous amounts of energy around the world. Waves are energy moving through water until they become breakers, at which point they become energy moving water forward. These breakers (also called whitecaps) are the white waves we see at the shoreline when we’re observing the ocean from the beach. There is little horizontal water movement in most waves, think of seagulls you have seen bobbing up and down in the ocean as a wave passes under it. The bird does not move forward with the wave. Instead, waves move water in a circular motion below the surface. Parts of a Wave Parts of a Wave The Crest – the highest point of a Wave height – the distance between the crest and wave. trough. The Trough – the lowest point of the Wave Steepness – the ratio between the wave wave. height and length. The steepness is what determines Wavelength – the distance between whether a wave will break. When the steepness the crests of two consecutive waves. exceeds a ratio of 1:7, the wave will form a breaker. Waves What Causes Waves? Waves form due to a disturbance of some kind. This disturbance is normally a result of one of the following: wind, gravity, or a natural disaster. Waves which cause the rise and fall of sea level and which are caused by the gravitational pull of the Sun and the Moon are called tides. In the open ocean, as crests rise, gravity pulls them downwards and back into the trough. This downward movement causes the former trough to move upward, eventually forming a new crest. Water moves in circles under the surface as waves pass through, and looks more like an up-and-down movement from above the surface. Water generally does not move forward until the trough of a wave hits the sea oor, forming a breaker. Parts of a Wave Waves Understanding Waves When a wave travels to the shore, it changes because the trough of the wave meets the ocean or lake oor. The wave touching the ocean or lake oor causes friction and forces the wave to slow down, allowing the waves behind it to catch up. This decreases wavelength. At this point, the wave still has the same energy as it did before touching the ocean or lake oor, so while wavelength has decreased, the wave height has increased (getting taller). Once the wave passes the 1:7 ratio, the wave will break and start to curl forward, forming a whitecap that will crash against the shore. Measuring Wave Speed Parts of a Wave Wind Speed A wave’s size and height are affected by wind speed, wind duration, and the distance over water that the wind blows in a certain direction (the fetch). We can calculate the speed of a wave by multiplying the wavelength (the distance between crests on two consecutive waves) by the wave frequency, which is the number of waves passing a given point in a certain amount of time. Therefore, the longer the wavelength, the faster the wave is. Waves Wind-Driven Waves Wind-driven waves, commonly referred to as surface waves, are the most common wave type. Capillary waves are surface waves that have extremely short wavelengths, causing the restoring forces of the waves to experience surface tension. These look like gentle ripples that begin when a light breeze blows across the surface of the water. These ripples generate larger surface waves as wind speed increases. Once the wavelength of a capillary wave exceeds 1.7cm, it becomes a surface wave. Parts of a Wave Waves Hazardous Waves Underwater disturbances like earthquakes and Hazardous waves are primarily caused by severe volcanic eruptions cause extremely large waves weather (intense winds) and natural disasters. called tsunamis. Hurricanes and strong storms can produce long People often confuse tidal waves and tsunamis, wave series called storm surges. These waves begin but tidal waves are caused by the gravitational pull in deep water and intensify as they near the shore. of the Sun and the Moon, not seismic disturbances (earthquakes). Parts of a Wave Waves Climate Change and Waves As we know, rising temperatures on Earth and atmospheric events have caused hydrological impacts like melting ice sheets and glaciers at the poles, which causes sea levels to rise. Since the 1980s, wave power has continuously grown in the Southern Hemisphere primarily because the heat from the Sun is increasing the energy in our oceans. More energy means larger, more powerful waves. How exactly this will impact coastlines depends on how the energy will affect ocean waves. Greater wave height will increase coastal erosion, which, as we know, will contribute to the loss of coastlines around the globe. However, if waves become longer, then this may become a bene cial phenomenon as the waves will transport sand from deeper water to the coast, which should help coastlines keep up with rising sea levels. Parts of a Wave Tides Tides Tides are the periodic rise and fall of surface water level. Tides are primarily caused by gravitational attraction of the sun and moon on Earth’s ocean, and by the spinning of Earth. Tides Tides As the Earth rotates, the Moon’s strong gravitational force pulls water toward a point on the Earth’s surface which is closest to the moon. This is called tidal force (the variable gravitational energy exerted on the Earth’s oceans by the Moon and Sun). The tidal force causes Earth’s water to bulge out on the side closest to the Moon and the side farthest from the Moon. Tides Tides The Sun’s gravitational pull also affects ocean tides, speci cally when the Earth, Moon, and Sun line up (during a full Moon or new Moon). When this lineup happens, lunar tides reinforce solar tides, causing extreme tides called spring tides. Tide Classi cations De nitions of Tides Tidal force – the variable gravitational energy exerted on the Earth’s oceans by the Moon and Sun. Lunar tides – the part of a terrestrial tide due to the mutual attraction between earth and moon. Solar tides – the part of a tide due to the tide-producing force of the sun Spring tides – tides occurring near the times of the new and full Moon, when the range of the tide is greatest. Neap tides – tides occurring near the times of the rst and last quarters of the Moon, when the range of the tide is least. Diurnal tide – one high tide and one low tide each day Semidiurnal tide – a tide with two high waters and two low waters each day. Mixed tide – type of tide in which large inequalities between the two high waters and the two low waters occur in a tidal day. Currents Currents On January 10, 1992, a cargo ship traveling from Hong Kong to the United States encountered a storm in the northern Paci c Ocean. Numerous cargo containers tumbled overboard, one of them spilling nearly 28,000 rubber ducks and other plastic bath toys into the ocean. Normally, rubber bath toys would have been manufactured with holes in their bottoms, so over time, a rubber duck would have been ooded with water, increasing the duck’s density and eventually making the toy sink into the ocean. However, these speci c rubber ducks were manufactured without holes, allowing them to oat in the ocean inde nitely! These infamous ducks have been found on costal shorelines around the world, including those of the United States, Canada, Japan, Australia, New Zealand, and South America. Some ducks reportedly made it to the Atlantic Ocean, while others have even been found trapped in Arctic ice! How did these duckies travel so far and in such different directions? How are some of them still out there today when others washed ashore years ago? Well, the short answer is ocean currents! Currents What is a Current? A current is the movement of a uid (a liquid or gas) in a de ned direction. Currents are continuous, predictable movements of water resulting from a variety of factors, including gravity, wind, water density, tides, and natural events such as earthquakes and storms. Water currents are also abiotic, which means they are nonliving components of an ecosystem. Currents River and Lake Currents Rivers ow from high areas to low areas, ending in a large body of water (normally, the ocean). The main cause of river currents is simply gravity. These currents can be in uenced by: the amount of water owing in a river the steepness of the river’s decline the geological features of the river such as sandbars, dams, and river deltas. Lakes experience both surface currents and deepwater currents and are They are in uenced primarily by: the weather the in ow and out ow of various water sources the geographical features of the lake bottom and shoreline. Ocean Currents Currents Ocean currents are great systems of owing water that follow predictable paths near the ocean’s surface and far below it. Some currents cross entire oceans, and some reach great depths while others ow relatively short distances. There are many factors that in uence ocean currents. The primary factors are: wind tides water density the rotation of the Earth the geography of the ocean oor surrounding land masses. There are two main types of ocean currents: surface currents and deepwater currents. Ocean surface currents account for currents in the top 300 meters of the ocean, and ocean deepwater currents are any currents below that Surface Currents Currents Like surface waves, ocean surface currents are driven by the wind as well as by a phenomenon called the Coriolis effect, which forces currents above the equator to bend to the right and currents below the equator to bend to the left. This happens, in part, from the Earth’s rotation on its axis from west to east. Surface Currents Currents For example, the trade winds are predictable winds that blow from east to west just above the equator. These winds create surface currents that ow to the west. However, the Coriolis effect de ects these currents, causing them to then bend to the right, heading north. Eventually, a different set of winds called the westerlies ends up pushing the currents back to the east, producing a closed clockwise loop. In the open sea, the surface ow is de ected at a 45° angle from the wind direction The EAC East Australian Current (EAC) What Is The East Australian Current? | Australia's O… O… Finding Nemo Marlin Meets Crush Ekman Spiral Currents Wind-driven surface water sets the water immediately below it in motion. But because of low-friction coupling in the water, this next deeper layer moves more slowly than the surface layer and is de ected to the right (Northern Hemisphere) or left (Southern Hemisphere) of the surface- layer direction. The same is true for the next layer down and the next. The result is a spiral in which each deeper layer moves more slowly and with a greater angle of de ection to the surface ow. This current spiral is called the Ekman spiral, after physicist V. Walfrid Ekman, who developed its mathematical relationship. The spiral extends to a depth of approximately 100-150 m (330-500ft), where the much reduced current will be moving in the opposite direction to the surface current. Over the depth of the spiral, the average ow of the water set in motion by the wind, or the net ow (Ekman transport), moves 90° to the right or left of the surface wind, depending on the hemisphere. The Gulf Stream Currents The Gulf Stream, a surface current that runs along the eastern coast of the United States and Canada, jets across the Atlantic to Iceland, the United Kingdom, and northern Europe, and it brings warm water from the Gulf of Mexico to the North Atlantic Ocean. England enjoys a much warmer climate than areas of Canada that are relatively the same distance from the equator, thanks to the warm water of the Gulf Stream. Gyres Currents Surface currents tend to form large, circular ocean current systems called gyres. Gyres form in both the northern and southern hemispheres and can be made up of many different currents. In fact, the Gulf Stream is a part of the North Atlantic Gyre. Ocean gyres spin clockwise in the northern hemisphere and counterclockwise in the southern hemisphere, bringing cold water from high latitudes to west coasts of continents and warm water to east coasts. Deep-Ocean Currents Currents Now that we’re starting to understand how currents form and move, we can move on to deeper ocean currents, which, unlike wind-driven surface currents, are fueled by differences in sea water density. These currents move nutrients, heat, and important chemicals like oxygen, nitrogen, and carbon dioxide throughout the ocean. The process that creates deep-ocean currents is called thermohaline circulation. Let’s break this term down! Water density is affected by temperature (thermo-) and salinity (haline). The colder and saltier the water is, the denser and heavier it is. Cold, dense water sinks, whereas warm, buoyant water rises. Deep-Ocean Currents Currents When surface currents bring warm water from the equator to the poles, the water will cool and become denser. These deep-ocean currents can also be called abyssal currents as they move predominantly throughout the abyssal plains of the ocean oor. The North Atlantic is quite cold, meaning surface water easily loses heat to the atmosphere and becomes denser. Additionally, when ocean water freezes and becomes ice, it leaves its salt behind, which causes the surrounding water to become saltier and, in turn, denser. That cold, salty, dense water sinks to the bottom of the ocean and starts traveling toward the equator while warm water from the equator ows into its place at the surface. This is the beginning of the global conveyor belt, a connected global system of deepwater and surface-water currents that circulate water between the equator and the poles. Global Conveyor Belt Currents This system is essential to Earth’s climate, ocean ecosystems, and global food webs because it regulates global temperatures, moves essential nutrients and chemicals throughout the world’s oceans, and disperses reproductive cells, which are vital to replenishing a population. Mobile marine life like sh and mammals will often move to different locations if the locations of their ecosystems are not bene ting their overall survival, which is why nutrient-poor areas tend to have lower marine life populations. These currents play an important role in circulating heat to the poles and regulating water temperature. Antarctic Circumpolar Current Currents The Antarctic Circumpolar Current (ACC) encircles the continent of Antarctica owing east. While this current may be relatively slow in comparison to others around the world, it moves more water than any other current on Earth. It spans from the surface to 4,000 meters deep (nearly the ocean oor!), so it is technically both a surface and deepwater current. It can reach widths of 2, 000 kilometers, and it can carry more than 100 times the water volume of all the rivers on Earth combined. In addition to its great volume, the ACC also ows through the Atlantic, Indian, and Paci c Oceans, occasionally touching the southernmost tip of Africa. The ACC is what keeps the waters of Antarctica cold while keeping the coastal waters of South Africa much colder than you might expect. Loop Current Currents The loop current is a well-known current that moves warm water from the Caribbean Sea, up past the Yucatan Peninsula, through the Gulf of Mexico, past the Florida Keys, and up the east coast of Florida. In fact, the loop current is one of the fastest currents in the entire Atlantic Ocean. It is also technically both a surface and deepwater current as it can reach depths of 800 meters. Depending on the time of year, the temperature of the water, and the intensity of surface winds, the direction of the loop current can vary. Some years, it barely enters the Gulf of Mexico, whereas during other years, it has nearly touched the coast of Louisiana! Tidal Currents Currents As we learned previously, tides rise and fall throughout the day. This is why some beaches have tide pools during low tide where you can explore the mesmerizing sea creatures that are fully submerged in sea water during high tide. This tidal movement causes currents near the shore. As the tide rises, it creates a ood current that moves water toward the shore. As the tides fall, an ebb current is created that moves water away from the shore. As the ebb current pulls water away from the shore, some sea water can get caught in little pockets and depressions within the intertidal zone, forming those lovely little tide pools! How Currents Cause Change Currents As we learned previously, the sea oor is a vast landscape with underwater mountains, valleys, ridges, and continental margins. When deepwater currents run into some of these geological features, the currents can become stronger or weaker. Currents are quite adaptable as they can turn and wind through underwater topography. Ocean currents play an essential role in controlling the climate by moving heat from the equator toward the poles. Currents also transport nutrients, sea life, reproductive cells, and chemicals such as oxygen and carbon dioxide throughout the world’s oceans. Many marine species rely on ocean currents to bring them food and nutrients and to distribute their reproductive cells, making currents very important for marine ecosystems. Turbidity Hitching a Ride! Currents Turbidity Currents Turbidity currents are rapid downward ows of water that are heavily packed with loose sediment and that move along continental margins. Turbidity currents can form on continental margins from underwater landslides on the continental shelf. These currents can even produce enough force to carve out underwater canyons and wipe out underwater telephone lines! Hitching a Ride! Many marine species rely on ocean currents to bring them food and nutrients and to distribute their reproductive cells, making currents very important for marine ecosystems. Also, the currents are a great way for juvenile animals to drift for long periods as they grow. One example of this is baby Loggerhead turtles, a type of sea turtle most commonly found off of Florida’s coasts. Female loggerheads like to lay their nests along the sands of both the Atlantic and Gulf beaches of Florida and after about 60 days of incubation, the little turtles wriggle their way out of the sand and down into the water. Then, they rely on ocean currents to get them to natural habitats like the North Atlantic Subtropical Gyre (kind of a lazy river that goes in a huge circle around the Atlantic) or the Sargasso Sea (the calm, seaweed-rich center of this circle). There they can grow up protected from predators until they are big enough to go back to the beach where they hatched. Without these important ocean currents, the population of loggerhead turtles that survive till adulthood would certainly be much lower! Upwelling Currents Upwelling is a type of current process in which deep, cold water rises to the surface. This normally occurs in one of two ways. The rst way upwelling occurs is along continental margins or lake shorelines where wind coming off land pushes surface water away from the shore. This causes cold, nutrient-rich water from the deep to rise to the surface and replace the water that was pushed away. As we know, a major component of uid dynamics is the characteristics of a uid. Typically, denser water does not rise, but when there is a void at the surface, deeper dense water has no choice but to ow into that area and ll the void. Currents Upwelling in Open Ocean The second cause of upwelling occurs in the open ocean. The Coriolis effect causes currents on either side of the equator to move in opposing directions. As these currents rush past each other, surface water is pulled in opposite directions, leaving a void right along the equator where deeper water can rush to the surface. Upwelling is a crucial part of the global food chain as it brings essential nutrients like nitrogen, phosphate, and carbon to the surface. These nutrients help the growth of phytoplankton and seaweed, which form the energy base for many organisms higher in the food chain like sh, marine mammals, and even humans! Many of the most productive ocean areas on Earth are in upwelling zones. Case in Point: Peru Open Ocean Upwelling - Peru The coast of Peru has some of the most nutrient- rich waters in the world, thanks to the oceanic upwelling that occurs in that area. Because of this, the Peruvian coast is considered one of the most productive oceanic zones in the world and is the largest exporter of anchoveta, a sh species similar to anchovies. Until 2009, there were little to no regulations on how many anchoveta shing boats could catch (which led to the near extinction of the species). Currents Longshore Currents Barrier islands, or long deposits of sediment off the coast of the mainland that eventually form islands, tend to create longshore currents along their coastlines. These currents are produced by waves that crash on shore. Do you remember how we talked about the energy of a wave approaching the shore? As the wave reaches the shore, it releases a burst of energy, which results in a current that runs the length of the shoreline. Waves often approach the shoreline at an angle, and as we learned, when waves travel ashore, they slow down. The steeper the angle at which a wave reaches the shore or the higher the wave crest is as it reaches the shore, the more intense the longshore current becomes. Increased Erosion Currents Changing Marine Environments To put it simply, increased ocean temperatures mean weaker currents. When the water at the poles gets warmer, it becomes less dense and doesn’t circulate as well. The melting of continental ice sheets and glaciers is not only increasing sea levels but is also adding fresh water to the oceans, making the water at the poles less salty. This, as we know, causes the water to be less dense. The reduced density lowers the overall transfer of oxygen and carbon dioxide to the deep ocean. What’s the big deal about that? Well, if oxygen is no longer able to reach the deep ocean, deepwater organisms won’t be able to thrive. The deep ocean is also the largest carbon dioxide reservoir on Earth and absorbs about 30 percent of atmospheric CO2. Ocean currents are essential to many global systems. However, they will change as Earth continues to change. Ocean Increased Acidi cation Erosion Ocean Acidi cation When thermohaline circulation slows, carbon dioxide builds up in surface waters, which leads to ocean acidi cation and other negative effects. Ocean acidi cation is the increase in overall water acidity in the ocean because of increased oceanic CO2 absorption. The ocean is a massive carbon reservoir. When CO2 dissolves in sea water, it forms carbonic acid (H2CO3), which breaks down into hydrogen and bicarbonate ions. This process decreases the pH of the ocean, making it more acidic. Since the dawn of the Industrial Revolution over 200 years ago, increased atmospheric CO2 has increased ocean acidity by about 30 percent. This sounds bad on its own, but what effect has this had on marine life? Take clown sh (like Nemo), for example. Studies have shown that clown sh lose their ability to detect predators in more acidic water, putting their population at risk for increased predation. On top of that, clown sh larvae appear to have more dif culty nding a suitable habitat as pH levels decrease in their environment. Water Cycle The Water Cycle The Water Cycle demonstrates the continuous circulation of water from the Earth’s surface to its atmosphere. Let’s start with the basic circular water cycle. In this circular cycle, liquid water evaporates into water vapor, water vapor condenses in the atmosphere to form clouds, those clouds eventually form precipitation, and the water falls back to Earth’s surface. Even though all of these processes are parts of the water cycle, they simplify the many ways in which water moves. Water Cycle Increased De nitions Erosion De nitions Evaporation - changing of water from liquid to gas Condensation – changing of water from gas to liquid Precipitation – Rain, snow, sleet or hail that falls to the ground. Runoff – Stormwater, melt water or other sources ow over the earth’s surface. Transpiration – Water evaporating from plant leaves Percolation – water moving through the soil Respiration- Animals take in oxygen and food, and release CO2 and water. Sublimation- snow and ice (solid) changing into water vapor (gas) in the air without rst melting into liquid water. De-sublimation- occurs when water vapor (gas) turns directly into solid ice or snow. Water Cycle The water cycle describes the circulation of water as it evaporates from land, water, and organisms; enters the atmosphere; condenses and is precipitated to the earth’s surfaces; and moves underground by in ltration or overland by runoff into lakes, rivers, and seas. Increased Erosion Water Cycle Importance of the Water Cycle As we know, water is essential to life on Earth. When the water cycle is interrupted by extreme climate events like oods and droughts, the entire ecosystem suffers the consequences. This is why it’s crucial that we protect water accessibility for human and environmental health. There are many factors that are currently hindering the water cycle from operating successfully. These include pollution, population growth, industrial development, and climate change. Increased Erosion Water Cycle Feedback Loops in the Water Cycle A feedback loop is a process that can occur within a system that will either decrease or increase the changes in that system. Therefore, some or all of the system’s output will either enhance or buffer that same system. Water vapor is considered the most abundant greenhouse gas, which means it’s a gas in the Earth’s atmosphere that can trap heat. Normally, water vapor only survives for a few days in the atmosphere. However, when the atmosphere warms, the amount of water vapor increases. More water vapor in the atmosphere increases the greenhouse effect, meaning that more heat gets trapped in the atmosphere. The greater the greenhouse effect, the warmer the atmosphere becomes, which again increases the amount of water vapor released to the atmosphere through evaporation. Do you see the loop here? A product of Feedback Loop the water cycle is water vapor. However, that water vapor will increase the greenhouse effect, which, in turn, will destabilize the water cycle. This is a De nitions Increased positive feedback loop in action. We will learn more about effects like this Erosion that cause climate change later on. Feedback Loop De nitions Positive Feedback Loop - The product of a system will increase a response. Positive feedback tends to move a system away from equilibrium and make it unstable. Negative Feedback Loop - The product of a system will decrease a response. Negative feedback tends to hold a system at equilibrium, making it more stable. The Water Cycle Water Cycle Rain Sky Ocean Stream River Wetland Lake Spring Land Increased Erosion Sea Level Sea Level is the average height of the ocean relative to land. It is the base level for measuring elevation and depth on Earth. Since the ocean is one continuous body of water, its surface tends to be at the same level throughout the world. However, winds, currents, river discharges, and variations in gravity and temperature prevent the sea surface from being truly level. In the Unites States, the average sea level is determined by taking hourly measurements of sea levels over a period of 19 years at various locations, and then averaging them all together. Sea Level Sea level varies from place to place, and changes over time. Differences in atmospheric pressure and prevailing winds affect the height of the sea level in different regions. The differences in the height of sea level is a factor that sets currents in motion. The movement of lithospheric plates can change the volume of ocean basins and the height of the land. Global temperature changes can bring about sea level change by causing ice caps to melt or grow, and by causing sea water to warm and expand, or cool and contract. During past ice ages, sea level was much lower because the climate was colder and more water was frozen in glaciers and ice sheets. Global warming is causing glaciers and ice sheets to melt. Melting ice sheets cause an elevation in sea level. This is known as sea-level rise. Sea-level rise affects low-lying areas around the world, especially island nations. A signi cant rise in sea-level could see a move in coastlines of the U.S. and other countries/continents. Currents The Webquest will Currents WebQuest be submitted to the Dropbox at the end of Unit 2 Lesson 3. Complete the Ocean Currents Webquest. Below is a link to the webquest and if you click on the image to the right it will take you to the site you will use to answer the questions in the webquest. You will submit your completed webquest to the dropbox. Introduction Principle 2 The ocean and life in the ocean shape the features of Earth. Rock Cycle and Plate Tectonics All the rocks on land will end up in the ocean due to weathering and erosion. The continual formation and breakdown of rocks constitutes the rock cycle. Biogeochemical Cycles The ocean plays a major role in the biogeochemical cycles that are fundamental to life on Earth. All elements are present in ocean water at various concentrations. Many elements in the ocean are needed by all living organisms. These include C, P, N, S, O and many metals such as Fe, Zn, Ca, Na, K. Other elements Si, Sr) are needed by some select organisms. Start Course Principle 2 Rock Cycle and Plate Biogeochemical Cycles Tectonics Quiz Rock Cycle and Plate Tectonics The Rock Cycle The rock cycle is a series of processes that transform one rock type into another. These processes create three main types of rocks: sedimentary, metamorphic, and igneous. Rocks are constantly being broken down and recycled through weathering, erosion, and processes associated with plate tectonics, such as subduction and uplift. Rocks are constantly being formed through accretion, sedimentation, volcanism, and igneous processes. Rock Cycle and Plate Tectonics Sedimentary Metamorphic Igneous Sedimentary rocks are formed Metamorphic rocks are formed Igenous rocks are formed when from pre-existing rocks or when rocks are subjected to molten hot material cools and pieces of once-living organisms. high heat, high pressure, hot solidi es. If they are formed They form from deposits that mineral-rich uids, or more inside the Earth they are called accumulate on the Earth's commonly, some combination of intrusive igneous. If they are surface. these factors. formed outside or on top of Earth's crust, they are called extrusive igneous rocks. Rock Cycle and Plate Tectonics Rock Cycle and the Ocean All rocks in Earth’s crust are constantly being recycled through the rock cycle. The rock cycle is the transition of rocks among three different rock types over millions of years of geologic time. Igneous rock is formed by the cooling and crystallization of molten magma at volcanoes and mid-ocean ridges, where new crust is generated. Examples of igneous rock are basalt, granite, and andesite. Over time, igneous rocks may experience weathering and erosion from exposure to water and the atmosphere to produce sediments. The deposition and hardening of these sediments forms sedimentary rocks. Both igneous and sedimentary rock types can transform physically and chemically into a third rock type. Metamorphic rocks are formed when igneous or sedimentary rocks are exposed to conditions of high heat and pressure. Examples of metamorphic rock include marble, slate, schist, and gneiss. Metamorphic rocks can also transform to sedimentary rocks through weathering, erosion, and sediment deposition. All three rock types in the earth’s crust—igneous, sedimentary, and metamorphic—can also be recycled back to their original molten magma form. This process occurs when oceanic crust is pushed back into the mantle at subduction zones. As old oceanic crust is subducted and melted into magma, new oceanic crust in the form of igneous rock is formed at mid-ocean ridges and volcanic hotspots. This recycling accounts for the recycling of 60 percent of Earth’s surface every 200 million years, making the oldest recorded oceanic crust rock roughly the same age. Because of this recycling, the age of the oceanic crust varies depending on location. Areas where new crust is being formed at mid-ocean ridges are much younger than zones further away (Fig. 7.58). By contrast, continental crust is rarely recycled and is typically much older. The oldest recorded rocks on Earth are all located on continental crust in northern Canada and western Australia and date to approximately 3.8 to 4.4 billion years old. Rock Cycle and Plate Tectonics Rock Cycle and the Ocean Many products of weathering and erosion enter the ocean via rivers and atmospheric deposition. All matter remains in the ocean for different lengths of time (residence time). The ocean continually batters the edge of lang, eroding it, breaking down the rock and transporting it out to sea. Chemical and biological processes in the ocean also ensure that there is a build-up of sediment at the bottom of the ocean. Over millions of years, these sediments are physically and Oceanic plates are more dense than continental plates. When they chemically changed into sedimentary rock. collide, the oceanic plate subducts beneath the continental, causing the continental plate to be lifted. Material can be scraped off of the oceanic plate and added to the continental plate (accretion). Subduction can result in the addition of oceanic rocks and sediments to the upper mantle or to the edge of the continent. There are several geologic features found in the ocean associated with subducted plates: ocean trenches, island arcs, stratovolcanoes, and some mountain ranges. Some parts of the ocean (e.g. the Paci c Rim) are dominated by subducted plate boundaries (Continental-Oceanic). Rock Cycle and Plate Tectonics More details Ocean Crust Production New ocean crust is produced at mid-ocean ridges, where two plates diverge from each other. Here, in response to the divergent motions, mantle material rises up to ll the void and as it rises, part of it melts. This molten material, magma, erupts on the sea oor and cools in place below the sea oor to form new oceanic crust. The new crust adheres in equal amounts to each of the two plates -- this is the way that oceanic plates grow. The rocks formed by this process are basalts and gabbros. The rate of formation is a function of the length of mid-ocean ridges and the average rate of plate motions, both of which may change over time. Initially, we will set this process to be a constant rate, equal to the present day value; a sensitivity to plate tectonic variations will be added later. Ocean Crust Subduction Ocean crust is destroyed through the process of subduction -- the sinking of cold, dense slabs of oceanic plates along plate boundaries where plate converge on each other. The subducted oceanic crust undergoes metamorphism and partial melting as it descends; the molten material then rises to form volcanoes while the metamorphosed remnants eventually remix with the rest of the mantle. These plate boundaries are associated with deep, narrow trenches and they are clearly delineated in three dimensions by the locations of earthquakes, which occur within and along the edges of the subducting plates. In general, almost all of the oceanic crust gets subducted, with the exception of protruding seamounts, plateaus, and other irregularities. Most of the sediment lying on top of the oceanic crust is also carried down into the mantle, undergoing metamorphism on its way to partially melting at depths of around 100 to 300 km below the surface. Like the production of oceanic crust, the destruction is also dependent on things like the length of subduction zones (which are generally about equal to the length of mid-ocean ridges) and the average rate of plate motions. This process will be de ned in the same manner as the ocean crust production. Rock Cycle and Plate Tectonics More details Arc Volcanism As mentioned above, when subduction occurs, molten material is generated; this magma has a different composition than the materials it is derived from, leading to the formation of rocks like andesite an even granite -- the starting materials for continental crust. The magma rises up to the surface forming volcanoes like Mt. St. Helens that occur in a line or an arc, paralleling subduction zones, giving rise to the term volcanic arcs. Volcanic arcs occur where the subducting plate reaches depths between 100 and 300 km; this is where the temperatures and pressures necessary for melting occur. We can think of these volcanic arcs as the places where new continental crustal material is formed and it turns out that this is the principle mode of formation; many other processes rework and reshape this arc material, but we can still think of the volcanic arc as the primary source of continental crust. The rate of arc volcanism is largely controlled by the length of subduction zones; the rate of subduction seems not to matter much. This process will be de ned in the same manner as the ocean crust production and subduction. Rock Cycle and Plate Tectonics More details Weathering of Continental Igneous Rocks When continental igneous rocks are exposed at the surface, they suffer chemical corrosion and physical abuse that we collectively call weathering. These rocks, many of which form far below the surface, are exposed as a result of uplift and erosion, which strips away overlying rocks. The most signi cant uplift and erosion occurs when are where large mountains ranges form -- at places where two large continents collide with each other (the Alps and Himalayas are examples of this kind of mountain chain). Weathering breaks the rocks down into a variety of products -- some material is carried away in solution and eventually ends up in the oceans; some material is in the form of new minerals such as clays (which commonly result from the alteration of feldspars), and some of the material is in the form of small rock and mineral particles in which there has been no mineralogical alteration from the parent rock. The non-soluble weathering products are carried away from the site of weathering by winds and running water; once these are liberated from the parent rock, they effectively become sediment particles and enter a new reservoir in our model. As mentioned above, this rate is tied to mountain-building activities, but it is also tied to simply the amount of continental igneous rocks that exist at any one time. For instance, if there are no continental igneous rocks, there will certainly be very little weathering of them. Conversely, if they make up a huge portion of the crustal rocks, then the weathering of them will be quite high. So, this process has some similarities to a typical draining ow and that is how we will represent it in our initial model. Rock Cycle and Plate Tectonics More details Lithi cation When sediment particles nally come to rest and are buried, they begin a process called lithi cation that will eventually turn them into sedimentary rocks. This is generally a slow process and requires pressure (supplied by the weight of overlying sediments) and usually some form of cement to bind together the different particles that make up the sedimentary rock. Cementation of many limestones occurs just after the particles are deposited on the sea oor, but many other types of sediment are not really lithi ed until they are buried to depths of up to a kilometer or more. The rate of lithi cation can be expected to vary according to how much sediment there is, so this ow will also be represented as being dependent on the reservoir it drains. Weathering of Sedimentary Rocks After sedimentary rocks form, they may undergo metamorphism or remain as sedimentary rocks, near the surface, for a long time, or they may be exposed and subjected to weathering processes. Rocks like limestones and dolostones and evaporites readily undergo dissolution and their products are carried away in solution by streams, eventually returning to the oceans. Other sedimentary rocks like sandstones and shales weather into sediment particles (often the same ones used to make the original sedimentary rock) and these particles then undergo transport and are eventually deposited to form new sedimentary rocks. Like the weathering of continental igneous rocks, this weathering ow will be de ned so as to make it dependent on the reservoir it drains; when there is more sedimentary rock, there will be more weathering products from sedimentary rocks. Rock Cycle and Plate Tectonics More details Metamorphism of Sedimentary Rocks When sedimentary rocks are buried to depths greater than about 5 to 10 km, they experience high enough pressures and temperatures to metamorphose into new rocks. Metamorphism is generally just a rearrangement of all the elements that make up the minerals found in a rock, in order to produce a new set of minerals that are closer to being in equilibrium at these elevated temperatures and pressures. This rearrangement occurs by slow, solid-state diffusion of atoms -- no melting is involved in this process. It happens that at these high temperatures and pressures, the rocks become weaker and any forces acting on the rocks will cause them to deform, and this deformation leads to the alignment of minerals. This alignment gives the rocks a fabric in the form of foliated, banded, or lineated textures. Deep burial is one way of obtaining the heat necessary for metamorphism; heat from magma is another important agent in metamorphism and since magma production on the continents is associated with volcanic arcs and subduction zones, a great deal of metamorphism also occurs near convergent plate boundaries. The rate of this process is probably related in part on plate tectonics to the extent that plate tectonics controls the rate of arc volcanism and the occurrence of continental collisions that force some rocks to be buried to great depths. But, this process is also sensitive to the amount of sedimentary rock that exists, so it too can be represented in part as a typical draining ow. Rock Cycle and Plate Tectonics Metamorphism of Sedimentary Rocks More details Metamorphism of Continental Igneous Rocks Sedimentary rocks are not the only kinds of rocks to undergo metamorphism. volcanic rocks, like sedimentary rocks, form at or near the surface, and so they generally require burial to undergo metamorphism. Plutonic igneous rocks, like granite, also undergo metamorphism, but unlike other rocks, they generally experience little in the way of mineralogical changes since the minerals found in granites are relatively stable at high temperatures because they formed at fairly high temperatures. But, the later heating of a rock like granite will cause it to become weak and susceptible to deformation. The deformation leads to the formation of a metamorphic fabric like gneissic banding. As with the metamorphism of sedimentary rocks, this process is tied in part to plate tectonics, and in part to the amount of rock material in the form of continental igneous rock; to begin with, it will be de ned as a standard draining process. Melting of Metamorphic Rocks Metamorphic rocks can either remain as metamorphic rocks, or they can follow one of three paths -- melting, uplift and weathering, or subduction. Melting is simply the result of continued heating and leads to the production of magma and thus new igneous rocks when the magma cools. This process, like many of the others, will be de ned so that it is dependent on the size of the reservoir it drains; later, we will also make it dependent on the relative intensity or activity of plate tectonics. Weathering of Metamorphic Rocks Just like igneous and sedimentary rocks, metamorphic rocks are commonly uplifted and exposed through erosion of overlying rocks, and then they are subjected to weathering. The products of this weathering are of course particles of sediment that then undergo transport and eventually are deposited to form sedimentary rocks. Similar to the other weathering ows, we will de ne this ow as a draining process -- dependent on the size of the metamorphic rock reservoir. Rock Cycle and Plate Tectonics More details Metamorphic Rock Subduction Any rocks that get caught up in the dynamics of a subduction zone may eventually get dragged down along with the subducting oceanic plate and as they get dragged down, they undergo metamorphism. Some sizable fraction of these rocks probably end up getting taken all the way down into the mantle, where they slowly mix with the rest of the mantle. This process is about the only way that rocks formed on the continents get recycled with the mantle. Like many of the other processes in this model, this one is probably controlled in part by the length of subduction plate boundaries and the rate of plate motions, but in part on the abundance of metamorphic rocks that exist or are being formed -- if the continents (all lumped together) were very small, for instance, there would be little continental rock adjacent to convergent plate margins where they could be subducted, whereas if there is a lot of continental material, then there is likely to be more continental material being subducted. To begin with, we will de ne this as a typical draining ow, and later we'll add in a sensitivity to variations in plate tectonics. Biogeochemical Cycles Biogeochemical Cycles Biogeochemical cycles inform how we understand the movement of essential elements like carbon, nitrogen, and water. These cycles involve the movement of various chemical elements through living and nonliving forms, from water to plants and soil to the atmosphere. Earth is a closed system, which means the amount of matter does not change, no matter how it is cycled through the atmosphere and the surface. We’ll see through looking at these cycles that matter does take on various forms, but the amount remains constant! Biogeochemical Cycles Carbon Cycle Phosphorus Nitrogen Cycle Silica Cycle Cycle All life on Earth depends on Some oceanic organisms nitrogen for amino acids (e.g., diatoms, radiolarian, The ocean is the largest All life on Earth depends on and proteins. Most of the sponges) use silica to reservoir of rapidly cycling phosphorus for important nitrogen (N) on Earth is in construct the hard parts of organic and inorganic compounds, e.g., ATP, DNA, the atmosphere as N2, their body, such as tests, carbon on Earth. and phospholipids. which cannot be used frustules, spines, and directly by most spicules. organisms. Carbon Cycle The Carbon Cycle Carbon is the chemical backbone of life on Earth. Carbon compounds regulate the Earth’s temperature, make up the food that sustains us, and provide energy that fuels our global economy. Most of Earth’s carbon is stored in rocks and sediments. The rest is located in the ocean, atmosphere, and in living organisms. These are the reservoirs through which carbon cycles. Terrestrial Path TERRESTRIAL CARBON CYCLE PATH Carbon moves from one storage reservoir to another through a variety of mechanisms. For example, in the food chain, plants move carbon from the atmosphere into the biosphere through photosynthesis. They use energy from the sun to chemically combine carbon dioxide with hydrogen and oxygen from water to create sugar molecules. Animals that eat plants digest the sugar molecules to get energy for their bodies. Respiration, excretion, and decomposition release the carbon back into the atmosphere or soil, continuing the cycle. Carbon Cycle The Carbon Cycle Carbon Cycle The Ocean's Role The ocean plays a critical role in carbon storage, as it holds about 50 times more carbon than the atmosphere. Two-way carbon exchange can occur quickly between the ocean’s surface waters and the atmosphere, but carbon may be stored for centuries at the deepest ocean depths. Rocks like limestone and fossil fuels like coal and oil are storage reservoirs that contain carbon from plants and animals that lived millions of years ago. When these organisms died, slow geologic processes trapped their carbon and transformed it into these natural resources. Uplift and accretion processes, as well as sea level changes, may relocate sedimentary rocks containing carbon onto land. Processes such as erosion release this carbon back into the atmosphere very slowly, while volcanic activity can release it very quickly. Burning fossil fuels in cars or power plants is another way this carbon can be released into the atmospheric reservoir quickly. Carbon Path + info OCEANIC CARBON CYCLE PATH EXAMPLE CO2 enters the ocean through diffusion, convective mixing, and bubble entrainment. Phytoplankton (photoautotrophs) convert the carbon through photosynthesis. Phytoplankton are consumed (eaten) by zooplankton. Zooplankton release carbon in three ways: in the form of CO2 through respiration, being consumed by another organism ( sh), or dyeing. Once dead, organisms decay and are eaten by decomposers who then release carbon through respiration, or become fossilized and may be used for fossil fuels. Carbon Cycle The Ocean's Role + info Carbon Cycle Changes to the Carbon Cycle Human activities have a tremendous impact on the carbon cycle. Burning fossil fuels (Releases excessive amounts of CO2 that is causing global warming/global climate change), changing land use (Deforestation and Farming Practices), Pollution, and using limestone to make concrete all transfer signi cant quantities of carbon into the atmosphere. As a result, the amount of carbon dioxide in the atmosphere is rapidly rising; it is already greater than at any time in the last 3.6 million years. The ocean absorbs much of the carbon dioxide that is released from burning fossil fuels. This extra carbon dioxide is lowering the ocean’s pH, through a process called ocean acidi cation. Ocean acidi cation interferes with the ability of marine organisms (including corals, Dungeness crabs, and snails) to build their shells and skeletons. Phosphorus Cycle The Phosphorous Cycle Of all the elements recycled in the biosphere, phosphorus is the scarcest and therefore the one most limiting in any given ecological system. It is indispensable to life, being intimately involved in energy transfer and in the passage of genetic information in the deoxyribonucleic acid (DNA) of all cells. Much of the phosphorus on Earth is tied up in rock and sedimentary deposits, from which it is released by weathering, leaching, and mining. Some of it passes through freshwater and terrestrial ecosystems via plants, grazers, predators, and parasites, to be returned to those ecosystems by death and decay. Much of it, however, is deposited in the sea, in shallow sediments, where it circulates readily, or in ocean deeps, whence it wells up only occasionally. Phosphorus is brought back to the land through sh harvests and through collection of guano deposited by seabirds. Although there are seasonal pulses of availability, there appears to be a steady loss of phosphorus to the ocean deeps. Phosphorus Cycle The Phosphorous Cycle Because of its high reactivity, phosphorus exists in combined form with other elements. Microorganisms produce acids that form soluble phosphate from insoluble phosphorus compounds. The phosphates are utilized by algae and terrestrial green plants, which in turn pass into the bodies of animal consumers. Upon death and decay of organisms, phosphates are released for recycling. Because of the steady diversion of phosphorus into the oceans, the element must be added (in fertilizers) to soils to maintain fertility and agricultural productivity. Phosphorus Cycle The Phosphorous Cycle Terrestrial weathering of rocks in the primary source of phosphorus in the ocean. Phosphorous is present in the ocean in dissolved inorganic forms, dissolved organic forms, particulate forms, and living and dead organisms. Phytoplankton and other primary producers take up phosphorous dissolved in seawater and convert it to biomass, which is consumed by heterotrophic organisms higher in the food chain (other animals). Dissolved and particulate phosphorous are converted back to its dissolved inorganic form through respiration and regeneration. Upwelling will bring inorganic phosphorous back up to the surface. Some organic and inorganic phosphorous will accumulate in ocean sediments, where it undergoes transformations and, over time, becomes part of sedimentary rocks. Phosphorus Cycle The Phosphorous Cycle Nitrogen Cycle Nitrogen Cycle Nitrogen is the most abundant element in our atmosphere. It is essential for the growth and development of living things on Earth. Much like the carbon cycle, nitrogen moves through both living and nonliving things and takes many different forms. Nitrogen exists in the atmosphere as a gas, represented by the symbol N2. While this form of nitrogen is abundant on Earth, it is relatively useless to plants and animals in its natural atmospheric form. Atmospheric nitrogen enters the soil through rain or snow and is then “ xed” by bacteria in the soil to form ammonia (NH3), which can then be used by plants for growth and development. Atmospheric nitrogen can also be xed into nitrogen dioxide (NO2) when lightning provides enough energy for oxygen and N2 to react. Like the carbon cycle, when dead plants and animals decay, they release nitrogen back into the surrounding soil. Various microorganisms in the soil take in nitrogen, removing nitrogen from the soil and storing it in their bodies. When humans and other animals eat nitrogen-containing plants, they absorb that nitrogen. Bacteria also convert nitrogen compounds in the soil back to gaseous nitrogen and return it to the atmosphere through a process called denitri cation. Nitrogen Cycle Nitrogen Cycle Nitrogen Cycle In the Marine Environment How does the nitrogen cycle relate to marine life? This same process happens in marine ecosystems, but in this case, marine bacteria cause nitrogen xation and denitri cation. When marine animals eat nitrogen- containing plants, they absorb the nitrogen from those plants. When we as humans eat marine animals, we inherit their nitrogen, and it moves its way up the food chain! Nitrogen-containing rocks and sediment also weather in marine environments because of waves and currents. This process releases nitrogen into the water and surrounding marine plants. In the open ocean, as on land, xed nitrogen is one of the most important growth-limiting nutrients for photosynthetic organisms (primary producers) such as algae and marine bacteria. Nitrogen can also serve as an energy source or as an oxidant for marine bacteria and archaea. (Archaea are single celled organisms that look similar to bacteria, but which are in an entirely separate biological domain). The ocean absorbs nitrogen gas from the atmosphere. In open-ocean areas with low concentrations of nutrients (“oligotrophic” regions), some of this nitrogen is taken up by microbes and transformed into various chemical compounds. The key steps in this process are listed on the "Steps tab" and shown in the simpli ed diagram at left (click on it to see a larger version). Steps STEPS OF THE NITROGEN CYCLE IN THE OCEAN 1. Nitrogen gas (N2) from the atmosphere dissolves into seawater at the ocean surface. Nitrogen gas is the most abundant form of nitrogen in the ocean, but is not useful to most living things. 2. Dissolved nitrogen gas is taken up by just a few types microbes, which convert the nitrogen into a much more useable form, known as ammonium (NH4+). This process, known as “nitrogen xation,” is vitally important. Without it, very little nitrogen would available for thousands of other organisms that live near the ocean surface. 3. Ammonium is the form of nitrogen that is most easily consumed by microorganisms. For this reason, ammonium is consumed almost as fast as it is produced, a process called “assimilation.” The result is that the nitrogen becomes incorporated into the cells of living organisms. 4. Some marine microbes consume nitrite and nitrate, another form of assimilation. 5. When microbes (and other organisms) die, they decompose, releasing ammonium and tiny particles containing particulate organic nitrogen (PON), as well as dissolved organic nitrogen (DON) into the surrounding seawater. 6. Some microbes convert ammonium to nitrite (NO2-) and then nitrite to nitrate (NO3-). This two-step process is called “nitri cation.” The result of this process is that nitrate is released into the ocean. 7. A host of organisms consume particulate organic nitrogen and dissolved organic nitrogen, converting some of the nitrogen back to ammonium. This process is called “remineralization.” 8. To complete this complex cycle (which begins to look more like a Celtic knot), some microbes convert nitrate and nitrite back to nitrogen gas through a process called “denitri cation.” Nitrogen Cycle Feedback Loops in the Nitrogen Cycle Even though some nitrogen is essential to life on Earth, too much of a good thing can actually be a bad thing! When excess nitrogen enters an aquatic system, it can trigger algae to grow faster than an ecosystem can handle, causing algal blooms. How does this happen? Well, excess nitrogen can enter a body of water in numerous ways: runoff from agricultural land where nitrogen is used in fertilizer, wastewater, runoff from land lls, drainage from urban areas, etc. When an algal bloom occurs, it can drastically reduce the amount of oxygen available in the water, which is extremely hazardous for marine life that require oxygen for survival. This phenomenon can also be harmful to humans as it produces toxins and bacteria that can make us sick if we encounter or ingest them. If we look at this phenomenon in terms of a feedback loop, algal blooms can cause mass death in marine species, speci cally sh. When those sh die, they decay on the sea oor, and as we’ve already learned, decaying plants and animals (organic matter) trigger the release of nitrogen into the surrounding sediment and water, which, in turn, continues to increase the amount of nitrogen in that body of water. Silica Cycle Silica Cycle Silica Cycle Silica Cycle The element silicon is everywhere! In fact, silicon is the second most abundant element in Earth’s crust. Silicon in rocks and minerals breaks down and is transported from rivers and streams into the world’s oceans. Many marine organisms need silicon as it is a crucial nutrient to build their skeletons. Silicon eventually reaches the sea oor, but its journey into the abyss is not straightforward due to biological, physical, and chemical processes. All these processes transport and transform silicon, creating a cycle that we call the marine silicon cycle. The silicon cycle is directly connected to the carbon cycle, making silicon a key player in the regulation of Earth’s climate Silica Cycle Silica Cycle The silicon cycle starts on land, via a process called rock weathering. Rocks on Earth are naturally broken apart by water from the rain and rivers, or by the wind. A common example is the coastline being destroyed after a big storm passes through, but this process also happens at a smaller scale on land, especially in the mountains. There, water from rain and rivers mixes with rocks made of silicon (called silicate rocks) and creates a chemical reaction that uses up the carbon dioxide (CO2) in the atmosphere. When the climate is warmer, there is more CO2 in the air. This makes it easier for CO2 to react with silicate rocks, which helps to remove CO2 from the atmosphere. In this way, silicate rocks and rain participate in the natural balance of our climate. This is what we call the silicate carbon sink. It helps to balance the amount of carbon in the atmosphere and oceans, and it can help to reduce the effects of human impact on climate change. Weathering is not only important for removing atmospheric CO2, but it also releases dissolved silicon into the waters owing into the rivers toward the ocean. This silicon is in a dissolved form (like when you put solid sugar or salt in water and it disappears), making it available as a nutrient for organisms on land and in the oceans. Silica Cycle Marine Silicon Cycle Because rivers, streams, and water within the ground eventually ow into the oceans, the dissolved silicon in these waters also ends up in the oceans. This is also where a new part of the silicon cycle begins— the marine silicon cycle. Silicon eventually makes it to the bottom of the oceans, but not by a direct path, and it takes a very long time— maybe as much as 8,000 years. In coastal areas, river waters and groundwaters supply the top layers of the ocean with dissolved silicon—a tasty new food for the organisms there. This ocean layer is home to many tiny organisms called phytoplankton that need sunlight to survive. Every spring and summer, the light from the sun and nutrients from the rivers create perfect growth conditions for a very important type of phytoplankton, called diatoms. Billions of diatoms grow in every ocean worldwide, but there is one special place on Earth where diatoms and other silicon-using marine organisms ourish, called the Southern Ocean. In the marine silicon cycle, silicon travels from the land into the oceans via rivers and groundwater. Near the ocean’s surface, silicon is taken up by phytoplankton like diatoms and incorporated into their skeletons. When these organisms die, they sink to the bottom of the ocean. Along the way, some of the skeletons dissolve and release silicon back into the water, but others end up buried in the sediment, along with the carbon they contain. Silica Cycle Marine Silicon Cycle Diatoms use silicon to build their armor-like skeletons, much like we use calcium to build strong bones. The skeleton of diatoms is very strong compared to other phytoplankton. As diatoms grow, they eat up all the dissolved silicon, but they also remove CO2 from the surface waters of the ocean and produce most of the oxygen we breathe. At the end of summer, diatoms die and sink toward the deeper layers of the ocean. Some diatom skeletons dissolve, and some continue their journey to the very bottom of the ocean. There, on the deep, dark sea oor, diatom skeletons get burie