Comparative Analysis of Bacillus cereus Group Isolates' Resistance Using Disk Diffusion and Broth Microdilution (PDF)
Document Details
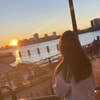
Uploaded by Mariana20
The Pennsylvania State University
2022
Emma Mills,Erin Sullivan,Jasna Kovac
Tags
Summary
This article details a comparative analysis of Bacillus cereus group isolates focusing on their antimicrobial resistance using disk diffusion and broth microdilution techniques. The research explores the correlation between antimicrobial resistance phenotypes and genotypes, highlighting the poor correlation between zones of inhibition and minimum inhibitory concentrations (MICs) for most tested antibiotics.
Full Transcript
FOOD MICROBIOLOGY Comparative Analysis of Bacillus cereus Group Isolates' Resistance Using Disk Diffusion and Broth Microdilution and the Correlation between Antimicrobial Resistance Phenotypes and Genotypes Emma Mills,a Erin Sullivan,a Jasna Kovaca Department of Food Science, The Pennsylv...
FOOD MICROBIOLOGY Comparative Analysis of Bacillus cereus Group Isolates' Resistance Using Disk Diffusion and Broth Microdilution and the Correlation between Antimicrobial Resistance Phenotypes and Genotypes Emma Mills,a Erin Sullivan,a Jasna Kovaca Department of Food Science, The Pennsylvania State University, University Park, Pennsylvania, USA a ABSTRACT Bacillus cereus group isolates (n = 85) were screened for phenotypic resist- ance to 18 antibiotics using broth microdilution and CLSI M45 Bacillus spp. breakpoints. The susceptibility to 9 out of 18 antibiotics was also tested using disk diffusion method and M100 Staphylococcus spp. breakpoints when available. Overall, a high prevalence of susceptibility to clinically relevant antibiotics was identified using broth microdilution. For most tested antibiotics, a poor correlation was found between zones of inhibition and MICs. Using the broth microdilution results as a reference for comparison, we identi- fied high error rates and low categorical agreement between results produced using disk diffusion and broth microdilution for the seven tested antibiotics with defined breakpoints. This suggests that disk diffusion should be avoided for AST of B. cereus group isolates. Further, we detected antimicrobial resistance genes with ARIBA and ABRIcate to calculate the sensitivity and specificity for predicting phenotypic resistance determined using broth microdilution based on the presence of detected antimicrobial resistance genes (ARGs). ARGs with poor sensitivity and high specificity included rph (rifampicin, 0%, 93%), mph (erythromycin, 0%, 99%), bla1 (penicillin, 29%, 100%), and Downloaded from https://journals.asm.org/journal/aem on 22 November 2024 by 24.157.36.115. blaZ (penicillin, 56%, 100%). Compared to penicillin, bla1 and blaZ had lower specificity for the prediction of ampicillin resistance. Overall, none of the ARGs had both high sen- sitivity and specificity, suggesting the need for further study of the mechanisms underly- ing phenotypic antimicrobial resistance in the B. cereus group. IMPORTANCE Bacillus cereus group includes human pathogens that can cause severe infec- tions requiring antibiotic treatment. Screening of environmental and food isolates for anti- microbial resistance can provide insight into what antibiotics may be more effective thera- peutic options based on the lower prevalence of resistance. Currently, the comparison of antimicrobial susceptibility testing results using the disk diffusion method is complicated by the fact that many previous studies have used Staphylococcus spp. breakpoints to inter- pret their results. In this study, we compared the results of disk diffusion interpreted using the Staphylococcus spp. breakpoints against the results of broth microdilution interpreted using Bacillus spp. breakpoints. We demonstrated that the disk diffusion method does not Editor Christopher A. Elkins, Centers for produce reliable results for B. cereus group isolates and should therefore be avoided. This Disease Control and Prevention study also provides new insight into poor associations between the presence of antimicro- Copyright © 2022 Mills et al. This is an open- access article distributed under the terms of bial resistance genes and resistance phenotypes for the B. cereus group. the Creative Commons Attribution 4.0 International license. KEYWORDS Bacillus cereus group, antimicrobial resistance, antimicrobial resistance Address correspondence to Jasna Kovac, genes, disk diffusion, broth microdilution, sensitivity, specificity [email protected]. The authors declare no conflict of interest. B Received 20 November 2021 acillus cereus group, also known as Bacillus cereus sensu lato (s.s.), comprises spore- Accepted 1 February 2022 forming, Gram-positive rod-shaped bacteria commonly found in the environment Published 22 March 2022 and food (1–5). The B. cereus group is composed of eight genomospecies that are March 2022 Volume 88 Issue 6 e02302-21 Applied and Environmental Microbiology aem.asm.org 1 Mills et al. Applied and Environmental Microbiology differentiated among each other based on the non-overlapping genome average nu- cleotide identity (ANI) of 95.2 (6). The B. cereus group genomospecies include B. pseu- domycoides, B. paramycoides, B. mosaicus, B. cereus sensu stricto (s.s.), B. toyonensis, B. mycoides, B. cytotoxicus, B. luti (6). These eight genomospecies are phylogenetically separated into eight phylogenetic groups, defined based on the panC gene polymor- phisms, that are consistent with the genomospecies taxonomy (7). The B. cereus group species vary in their cytotoxicity toward human cells, which can be used as an indicator of pathogenicity. Bacillus cereus species (Bacillus cereus s.s.), which is known as a foodborne pathogen, has been reported to be overrepresented by strains with cytotoxic capability (8, 9). Scallan et al. (2011) estimated that 63,400 cases of Bacillus cereus foodborne illness occur in the United States annually (3). The prevalence of infections with B. cereus group bacteria may be underestimated due to the nature of foodborne illnesses and the passive surveillance of B. cereus. Although foodborne illness cases caused by B. cer- eus tend to be self-limiting, severe infections reported in the past required hospitaliza- tion and were lethal (10–13). For example, a 2003 outbreak linked to pasta salad conta- minated with B. cereus consisting of 5 children led to one fatality (10). In 2008, another fatal case of B. cereus infection was reported due to the consumption of contaminated pasta (11). B. cereus was also reported to cause other types of severe infections. In a ret- rospective study conducted at a French university hospital between 2008 and 2012, they found that nearly 30% of 57 patients hospitalized due to B. cereus infection had bacteremia and 28% had skin infection (14). Of the 57 hospitalized patients, 12% had died (14). Others have also reported B. cereus group infections associated with the hos- pital environment and supplies, including catheter-associated bloodstream infections in children in Japan and contaminated health care kits associated with cutaneous an- thrax-like infections in newborns in India (15, 16). Although most B. cereus group infec- tions may not require antimicrobial treatment, it is important to prepare for those that warrant critical care, particularly if novel infectious strains emerge within the B. cereus group. The Centers for Disease Control and Prevention recommend ciprofloxacin, doxycy- cline, and b -lactam antibiotics for treatment of inhalation exposure to B. anthracis (genomospecies B. mosaicus biovar Anthracis = B. Anthracis) (17). Severe non-anthrax Downloaded from https://journals.asm.org/journal/aem on 22 November 2024 by 24.157.36.115. Bacillus cereus infections are commonly treated with vancomycin, gentamicin, linezolid, levofloxacin, and clindamycin antibiotics (18–21). These antibiotics are widely used clini- cally due to the high prevalence of b -lactamases found among Bacillus cereus group iso- lates (22–24), although specific b -lactams (e.g., carbapenems) are also used in combination for the treatment of clinical cases (18, 25). Notably, there are reports of poor patient out- comes due to carbapenem resistance (12, 26). Recent studies have shown a high preva- lence of resistance against penicillin, early cephalosporins, and 3rd and 4th generation cephalosporins among environmental isolates (2, 27–29). There have also been reports of a high prevalence of trimethoprim-sulfamethoxazole resistance in environmental isolates from Germany, China, and Ghana (2, 27, 29). Understanding the prevalence of antimicrobial resistance among B. cereus group isolates can inform the selection of antibiotics for thera- peutic use and potentially improve treatment outcomes. In addition to understanding the prevalence of antimicrobial nonsusceptibility phe- notypes, the information about underlying mechanisms of phenotypic antimicrobial resistance is critical for the development of rapid diagnostic assays and for the surveil- lance of antimicrobial resistance. Studies on other foodborne pathogens, such as Salmonella and Campylobacter, have demonstrated the existence of a strong relationship between antimicrobial resistance genes (ARG) or resistance mutations in housekeeping genes and phenotypic antibiotic resistance (30, 31). However, this relationship is yet to be characterized for the broad Bacillus cereus group, beyond B. anthracis (17). To study the correlation between individual ARGs and phenotypic resistance, iso- lates’ susceptibility needs to be determined using standard methods and resistance interpretation breakpoints. Two commonly used methods for phenotypic antimicrobial March 2022 Volume 88 Issue 6 e02302-21 aem.asm.org 2 Resistance of Bacillus cereus Group Isolates Applied and Environmental Microbiology susceptibility testing include disk diffusion and broth microdilution, which provide in- formation on the zones of inhibition or MICs, respectively. The interpretation of the results of disk diffusion for B. cereus isolates is challenged by the fact that current CLSI M45 guidelines only provide breakpoints for broth microdilution for Bacillus spp. (excluding B. anthracis). When utilizing the disk diffusion method, many have therefore applied Staphylococcus spp. CLSI M100 breakpoints for the interpretation of zones of inhibition for B. cereus group isolates (27, 29, 32). It is unclear whether the disk diffu- sion results interpreted using the S. aureus resistance breakpoints (CLSI M100) produce results consistent with the results of broth microdilution (i.e., gold standard method) interpreted using Bacillus spp. breakpoints defined in the CLSI M45. Hence, we sought to evaluate the consistency of resistance interpretation for 85 B. cereus isolates using disk diffusion results interpreted using S. aureus breakpoints (CLSI M100) and broth microdilution results interpreted using Bacillus spp. breakpoints (CLSI M45). We further assessed the relationship between the detected ARGs and broth microdilution-based antimicrobial resistance phenotypes to determine whether individual antimicrobial re- sistance genes are sensitive and specific markers of phenotypic resistance. RESULTS AND DISCUSSION The tested isolate collection was phylogenetically diverse and represented by five B. cereus group species. A total of 85 Bacillus cereus group isolates were available in our culture collection and were included in this study. These isolates had been previ- ously collected from dairy-associated environments (65%), food processing environ- ments (27%), and natural environments (7%) between 2002 to 2014, mostly from New York state (89%). All isolates and the corresponding metadata are listed in Table S1. Whole-genome sequences for 85 isolates were obtained from the NCBI SRA database and were assembled (8, 33, 34). The average N50 of assembled genomes was 217 Kbp, the average genome size was 5.6 Mbp, and the average number of contigs longer than 1,000 bp was 129 contigs (supplemental material, Table S1). Isolates were taxonomically identified using the average nucleotide identity (ANI) method implemented in the BTyper3 (36). Isolates belonged to 5 different species and the most abundant species were B. mosaicus (33%), B. mycoides (27%), and B. cereus s.s. (24%) (Table S1). Consistent with the species identification, most isolates were classi- Downloaded from https://journals.asm.org/journal/aem on 22 November 2024 by 24.157.36.115. fied in panC phylogenetic groups VI mycoides/paramycoides (31%), II mosaicus/luti (26%), and IV cereus sensu stricto (26%) (supplemental material, Table S1). There was a high MLST sequence type (ST) diversity among isolates, with 62 unique ST identified (sup- plemental material, Table S1). Among these, 80% of identified STs were singletons. The most abundant STs were 1099 (n = 6), 1272 (n = 4), 222 (n = 4) and 410 (n = 3), and 1346 (n = 3), which belonged to phylogenetic groups IV cereus s.s., II mosaicus/luti, VI mycoides/ paramycoides, and I pseudomycoides, respectively (supplemental material, Table S1). The 5 isolates carrying Bt toxin-encoding genes (i.e., defined as biovar Thuringiensis) belonged to three species, B. cereus s.s. biovar Thuringiensis (n = 2), B. mycoides biovar Thuringiensis (n = 2), and B. mosaicus biovar Thuringiensis (n = 1). In addition to genetic diversity, we also examined the prevalence of genes encoding for three major enterotoxins that have previously been associated with toxicoinfection caused by B. cereus group isolates (35). These include the nonhemolytic enterotoxin encoded by an operon nheABC, the hemolysin BL encoded by an operon hblABCD, and the cytotoxin K encoded by cytK, all of which are proteinaceous pore-forming enterotoxins (35). All isolates carried at least one gene from the nonhemolytic enterotoxin nheABC operon, which is simi- lar to reports from Ghana and China where they had also reported a high prevalence of nhe genes in isolates obtained from dairy-associated environments and ready-to-eat foods, respectively (2, 36) (supplemental material, Table S1). In 13 isolates’ genomes, we did not detect any hbl genes, whereas 65 isolates carried hblABCD, 6 carried hblCD, and only one carried hblACD (Table S1), alike abundance reported in previous studies (2, 36). A total of 40% of tested isolates harbored a gene encoding cytotoxin K-2, which is similar to the prevalence reported in Germany (37%) (29). It is important to note that the prevalence of March 2022 Volume 88 Issue 6 e02302-21 aem.asm.org 3 Mills et al. Applied and Environmental Microbiology virulence genes may be underreported in our study due to the use of draft genomes for gene detection. Poor correlation between zones of inhibition and MICs demonstrates that the disk diffusion method is unsuitable for Bacillus cereus group antimicrobial susceptibility testing. Nine antibiotics (i.e., ampicillin, ceftriaxone, ciprofloxacin, erythromycin, genta- micin, rifampicin, tetracycline, trimethoprim-sulfamethoxazole, and vancomycin) were tested and compared using disk diffusion and broth microdilution assays to determine the relationship between these two methods (Fig. 1). We found an overall poor correla- tion between the results of the two methods, which was reflected in low R2 values for nine tested antibiotics. R2 values ranged from the lowest of 3.9 1025 for gentamicin to the highest of 0.1238 for tetracycline. Since all MIC values for trimethoprim in com- bination with sulfamethoxazole were.4/76 (m g/mL), the R2 could not be calculated for this combination of antibiotics. Although the disk diffusion method has often been used for determining antimicrobial susceptibility of Bacillus cereus group isolates (27, 29, 36, 37), the CLSI M45 manual only recommends the broth dilution method for the most reliable susceptibility testing (38). Our findings are consistent with this guideline as we found a poor correlation between zones of inhibition obtained with disk diffu- sion and MICs obtained with broth microdilution. We, therefore, advise against the use of the disk diffusion method for susceptibility testing of B. cereus group isolates. High error rates suggest that the disk diffusion test with results interpreted using Staphylococcus spp. breakpoints should be avoided when testing the antimicrobial susceptibility of B. cereus group isolates. Seven antibiotics (i.e., ampicillin, ciprofloxacin, erythromycin, gentamicin, rifampicin, tetracycline, trimethoprim-sulfamethoxazole) for which CLSI M100 Staphylococcus spp. and CLSI M45 Bacillus spp. breakpoints have been defined, were included in the analysis to determine the error rates and categorical agree- ment between disk diffusion and broth microdilution methods (Table 1). The results of broth microdilution interpreted with CLSI M45 Bacillus spp. breakpoints were used as a ref- erence when comparing with the results of disk diffusion interpreted with M100 Staphylococcus spp. breakpoints. Minor, major, and very major error rates were calculated. A minor error is an error that occurs when one method reports an intermediate result, and the other method reports a susceptible or resistant outcome. A major error occurs when disk diffusion testing reports a resistant result, and the broth microdilution reports a sus- Downloaded from https://journals.asm.org/journal/aem on 22 November 2024 by 24.157.36.115. ceptible result. A very major error occurs when the disk diffusion reports a susceptible result, and the broth microdilution reports a resistant result. The minor and major errors occurred most frequently when isolates were tested for susceptibility to tetracycline (35%) and rifampicin (34%), respectively. A very major error occurred most frequently when iso- lates were tested for susceptibility to ampicillin (35%). Consistent with these findings, the categorical agreement (i.e., % of isolates for which both methods produced the same result) was lowest for rifampicin (39%), tetracycline (54%), and ampicillin (65%). In contrast, the highest categorical agreement was obtained for gentamicin (88%), ciprofloxacin and trimethoprim-sulfamethoxazole (78%), and erythromycin (74%). Overall, these results indi- cate that the application of Staphylococcus spp. breakpoints, although commonly used for determining antimicrobial susceptibility of Bacillus cereus group isolates (27, 29, 36, 37), can cause inaccurate interpretation of antimicrobial susceptibility and should therefore be avoided. Instead, the broth microdilution method is advised to be used for antimicrobial susceptibility testing of B. cereus group isolates. The results of broth microdilution may be interpreted using the CLSI M45 guideline (38). The prevalence of resistance to clinically relevant antibiotics levofloxacin, clindamycin, and linezolid was low. A total of 12 of the 18 antibiotics tested using broth microdilution had defined clinical breakpoints in either CLSI M45 (i.e., ampicillin, ciprofloxacin, clindamycin, erythromycin, gentamicin, levofloxacin, penicillin, rifampi- cin, tetracycline, trimethoprim-sulfamethoxazole, and vancomycin) or EUCAST v12.0 (i.e., linezolid) for Bacillus spp. All AST results are reported in the Supplemental Material, Table S2 and the distributions of MICs for these antibiotics are shown in the Supplemental Material, Fig. S1. Susceptibility testing revealed no or low prevalence of resistance to antibi- otics clindamycin (0%), ciprofloxacin (0%), erythromycin (0%), gentamicin (8%), levofloxacin March 2022 Volume 88 Issue 6 e02302-21 aem.asm.org 4 Resistance of Bacillus cereus Group Isolates Applied and Environmental Microbiology Downloaded from https://journals.asm.org/journal/aem on 22 November 2024 by 24.157.36.115. FIG 1 Scatterplots showing the analysis of zones of inhibition produced by disk diffusion method (Y axis) and MICs produced by broth microdilution method (X axis) for antibiotics (A) ampicillin, (B) ceftriaxone, (C) ciprofloxacin, (D) erythromycin, (E) gentamicin, (F) rifampicin, (G) tetracycline, (H) trimethoprim-sulfamethoxazole, and (I) vancomycin. R2 values for each antibiotic tested are shown below an antibiotic name. March 2022 Volume 88 Issue 6 e02302-21 aem.asm.org 5 Mills et al. Applied and Environmental Microbiology TABLE 1 Error rates and categorical agreement for antimicrobial susceptibility testing results of B. cereus group isolates obtained using disk diffusion and broth microdilution (reference) methods Percent (count) Minor Major Very Categorical Antibiotic error error major error agreement (%) Total Ampicillin 0 0 35 (30) 65 (55) 85 Ciprofloxacin 19 (16) 1 (1) 2 (2) 78 (66) 85 Erythromycin 26 (22) 0 0 74 (63) 85 Gentamicin 4 (3) 0 8 (7) 88 (75) 85 Rifampicin 24 (20) 34 (29) 4 (3) 39 (33) 85 Tetracycline 35 (30) 11 (9) 0 54 (46) 85 Trimethoprim-sulfamethoxazole 7 (6) 0 15 (13) 78 (66) 85 (0%), linezolid (2%), rifampicin (8%), tetracycline (4%), and vancomycin (2%) (Table 2). The seven gentamicin and seven rifampicin-resistant isolates were diverse and belonged to dif- ferent phylogenetic groups and MLST sequence types (Fig. 2). Two of the three tetracy- cline-resistant isolates belonged to phylogenetic group II mosaicus/luti (Fig. 2). The two iso- lates resistant to linezolid and vancomycin belong to phylogenetic groups II mosaicus/luti and V toyonensis. Although no isolates were identified as resistant to clindamycin, 39% (n = 33) were found to be intermediate (Table 2). Nearly half (42%) of all clindamycin inter- mediate isolates belonged to phylogenetic group IV cereus sensu stricto (Fig. 2). Similarly, there were no erythromycin-resistant isolates, but 20% were identified as intermediate and were found among different phylogenetic groups (Fig. 2). All or nearly all isolates were re- sistant to ampicillin (98%), penicillin (100%), and trimethoprim-sulfamethoxazole (100%) (Table 2). While we describe to which phylogenetic groups resistant isolates belong, we did not carry out a statistical analysis of associations, because our data set does not meet the conditions for the validity of chi-square analysis. Specifically, the expected counts were less than 5 in some categories due to some phylogenetic groups being represented by fewer than 5 isolates (e.g., group 1, 5) and/or specific resistance genes or phenotypes being observed in fewer than 5 isolates per clade. Downloaded from https://journals.asm.org/journal/aem on 22 November 2024 by 24.157.36.115. In terms of resistance prevalence, the prevalence of resistance to clinically relevant antibiotics, such as vancomycin, levofloxacin, clindamycin, and linezolid was low. This is consistent with the reports that the use of these antibiotics for the treatment of B. cereus group infections leads to positive clinical outcomes (19–21, 39). A group in Ghana used broth microdilution to test antimicrobial susceptibility of 96 dairy-associ- ated Bacillus cereus group isolates, which allows for direct comparison of their report TABLE 2 Prevalence of antimicrobial resistance among 85 tested B. cereus group isolates Percentage (count) Antibiotic Interpretation breakpoints Ra Ib Sc Linezolid EUCAST v12.0 Bacillus spp. 2 (2) 0 98 (83) Ampicillin M45 CLSI Bacillus spp. 98 (83) 0 2 (2) Ciprofloxacin M45 CLSI Bacillus spp. 0 2 (2) 98 (83) Clindamycin M45 CLSI Bacillus spp. 0 39 (33) 61 (52) Erythromycin M45 CLSI Bacillus spp. 0 20 (17) 80 (68) Gentamicin M45 CLSI Bacillus spp. 8 (7) 4 (3) 88 (75) Levofloxacin M45 CLSI Bacillus spp. 0 0 100 (85) Penicillin M45 CLSI Bacillus spp. 100 (85) 0 0 Rifampicin M45 CLSI Bacillus spp. 8 (7) 2 (2) 89 (76) Tetracycline M45 CLSI Bacillus spp. 4 (3) 0 96 (82) Trimethoprim/Sulfamethoxazole M45 CLSI Bacillus spp. 100 (85) 0 0 Vancomycin M45 CLSI Bacillus spp. 2 (2) 0 (0) 98 (83) aR, resistant. bI,intermediate. cS, susceptible. March 2022 Volume 88 Issue 6 e02302-21 aem.asm.org 6 Resistance of Bacillus cereus Group Isolates Applied and Environmental Microbiology Downloaded from https://journals.asm.org/journal/aem on 22 November 2024 by 24.157.36.115. FIG 2 Phylogenetic tree for 85 B. cereus group isolates, annotated with the panC phylogenetic grouping (panC), MLST sequence type (ST), phenotypic resistance to ampicillin (AMP), penicillin (PEN), ciprofloxacin (CIP), levofloxacin (LVX), clindamycin (CLI), erythromycin (ERY), gentamicin (GEN), linezolid (LZD), tetracycline (TET), trimethoprim-sulfamethoxazole (SXT), and vancomycin (VAN). Phenotypic resistance was tested using the broth microdilution assay and interpreted using CLSI M45 Bacillus spp. breakpoints, except for linezolid for which EUCAST v12.0 Bacillus spp. breakpoints were used. The genes listed next to each antibiotic have previously been linked with phenotypic resistance to these antibiotics. with the results from the present study (2). Similar to our results, Owusu-Kwarteng and colleagues identified all isolates susceptible to ciprofloxacin, gentamicin, rifampin, tet- racycline, and vancomycin, and all isolates resistant to ampicillin and penicillin (2). They identified high trimethoprim-sulfamethoxazole resistance prevalence (80%), how- ever the resistance prevalence in our study was greater (2). Lastly, higher prevalence of susceptibility to clindamycin (100%) was identified among isolates studied in Owusu- Kwarteng et al., compared to isolates studied here (61%) (2). The 6 remaining antibiotics (i.e., ceftriaxone, daptomycin, gatifloxacin, oxacillin, qui- nupristin-dalfopristin, and streptomycin) tested with broth microdilution did not have defined clinical breakpoints for Bacillus spp. in CLSI or EUCAST guidelines. Definitions of ecological cutoffs were attempted using EUCAST ECOFFinder; however, due to our skewed distributions and the fact that our data set does not meet the criteria outlined March 2022 Volume 88 Issue 6 e02302-21 aem.asm.org 7 Mills et al. Applied and Environmental Microbiology by EUCAST, ecological cutoff values were left undetermined (40, 41). The distributions of MICs for these antibiotics are shown in Supplemental Material, Fig. S2. ABRIcate in conjunction with MEGARes 2.0 database resulted in the detection of the largest number of antimicrobial resistance genes. Antimicrobial resistance genes were detected using both unassembled reads with ARIBA v2.14.6 and assembled genomes with ABRIcate v1.01., in conjunction with both MEGARes 2.0 and ResFinder 4.1 databases. ARGs detected in the 85 B. cereus group isolates are reported in Supplemental Material, Table S3. ABRIcate in conjunction with MEGARes 2.0 and ResFinder detected a median of 4 and 2 ARGs per isolate, respectively (supplemental material, Table S3). A search with ARIBA in conjunction with MEGARes 2.0 and ResFinder 4.1 databases resulted in a median of 2 and 0 detected ARGs per isolate, respectively. Given that the largest number of ARGs was detected using ABRIcate in conjunction with MEGARes 2.0 database, the genes identified by this program and database combination were used in further analysis (supplemental material, Table S3). The detection of acquired antimicrobial resistance genes by two different programs in conjunction with two different databases resulted in a different number of detected genes. Premkrishnan et al. also identified discrepancies in the number of detected anti- microbial resistance genes when using different methods for the detection of ARGs (42). This finding shows the importance of using multiple ARG detection tools to improve the detection of antimicrobial resistance genes (42). b-lactamase-encoding genes were detected in most isolates while other resistance genes such as tet and mph were less prevalent. Nearly all isolates (93%) harbored intrinsic antimicrobial resistance genes Bc (n = 53) and/or a BcII (n = 76) that encode zinc metallo- b -lactamases. These genes are known to confer resistance to pen- icillins, carbapenems, and cephalosporins in B. cereus group species (Fig. 1) (23, 43, 44). Of the six isolates that did not have these genes detected, five belonged to panC phy- logenetic group I (pseudomycoides) (Fig. 2). Within these five group I (pseudomycoides) isolates, the only gene identified was rph (rifampin phosphotransferase). The effect of this gene on rifampicin resistance in Bacillus cereus is still unclear. Three additional b -lactamase-encoding genes were identified among 85 tested isolates, including bla1 (n = 25), blaZ (n = 48), and blaTEM (n = 1, ST-365) (Supplemental Material, Table S3). bla1 was only identified in half of the panC clades including II mosaicus/luti, III mosai- Downloaded from https://journals.asm.org/journal/aem on 22 November 2024 by 24.157.36.115. cus, VI mycoides/paramycoides (Fig. 2). blaZ was sporadically scattered among most clades and sequence types (Fig. 2). All isolates carried a fosB, a thiol transferase-encod- ing gene that was previously associated with fosfomycin resistance in other bacterial species (22) (Supplemental Material, Table S3); however, the phenotypic resistance to fosfomycin was not tested as this antibiotic was not included in the Sensititre plate an- tibiotic panel. Erythromycin resistance-conferring genes mphB (n = 2) and mph (n = 11) were mostly identified in groups III mosaicus (n = 4), IV cereus sensu stricto (n = 4), and VI toyonensis (n = 3) (Fig. 2) (29). Only two isolates carried tetracycline predicting genes, tet45 or tetL, found in groups III mosaicus and II mosaicus/luti respectively (Fig. 2). Although van genes, vanRA (n = 9), vanSA (n = 9), vanYA (n = 1), and vanYF (n = 57), were identified in our collection, none were associated with vancomycin resistance (Fig. 2) (45). In order to confer vancomycin resistance an isolate must have all vanM/ vanB glycopeptide resistance genes present (46). Only a few published studies have focused on characterizing the presence and abun- dance of acquired ARGs in B. cereus group isolates (29, 47, 48). Bianco et al. investigated the prevalence of ARG in a collection of 17 B. cereus group clinical blood isolates (47). Similar to what we report for our isolate collection, they reported a high prevalence of b -lactamase-encoding genes, specifically bla-1 and bla-2 (48). In our isolates collection, we found a substantially higher prevalence of the blaZ compared to the prevalence of this gene among the 17 clinical isolates studied in Bianco et al. (47). In our study, the Bianco et al. study, and the Fielder et al. study, fosB was identified in all isolates (29, 47). While Bianco et al. (47) identified a relatively high number of isolates carrying a specific mphB allele (erythromycin resistance-conferring), we found a low prevalence of the mphB allele (2%), but higher prevalence of the mph allele (13%). Similarly to our findings, Bianco et al. March 2022 Volume 88 Issue 6 e02302-21 aem.asm.org 8 Resistance of Bacillus cereus Group Isolates Applied and Environmental Microbiology TABLE 3 Sensitivity and specificity of antimicrobial resistance genes to predict phenotypic resistance Antibiotic AGRa Sensitivity (%)b Specificity (%)b Ampicillin bla1 28.92 50.00 blaZ 56.63 50.00 Penicillin bla1 29.41 100.00 blaZ 56.47 100.00 Erythromycin mph 0.00 98.52 Rifampicin rph 0.00 93.42 aARG, antimicrobial resistance gene. bSensitivity and specificity were calculated based on the broth microdilution results interpreted with CLSI M45 Bacillus spp. breakpoints. Only susceptible and resistant isolates, but not intermediate isolates, were included in the calculation. detected tetL in just a single clinical isolate (47). Tetracycline resistance-conferring gene tet45 was not identified among the clinical blood isolates studied in by Bianco et al. (47) but was abundant among isolates studied by Zhu et al. (48). Zhu et al. (48) identified 8 of the 17 isolates carrying tet45 as B. cereus (n = 7) and B. thuringiensis (n = 1). In comparison, we identified a single B. moscaicus isolate that carried tet45. Comparison of antimicrobial phenotypes and genotypes revealed insufficient sensitivity and specificity for predicting antimicrobial resistance phenotypes based on the presence of ARGs. Sensitivity and specificity were calculated for genes that were found in five isolates or more (bla1, blaZ, mph, and rph) detected by ABRIcate/ MEGARes 2.0 and using broth microdilution results interpreted with CLSI M45 Bacillus spp. breakpoints for the corresponding antibiotics. Bc and BcII were excluded from the analysis due to their intrinsic resistance nature and the well characterized effect on b -lactam resistance (23, 43, 44). Further, all van genes identified were excluded from analysis as individual van genes are insufficient to confer phenotypic resistance to vanco- mycin (45, 46). ARGs that did not meet the abundance threshold (n $ 5) were excluded from the sensitivity and specificity analysis but were investigated to assess their effect on the MICs for their respective antibiotics. The single blaTEM isolate was phenotypically resistant to both ampicillin (.16 m g/mL) and penicillin (8 m g/mL). The isolate carrying mphB was susceptible to erythromycin (MIC ,0.25 m g/mL). Two isolates carrying tetracy- Downloaded from https://journals.asm.org/journal/aem on 22 November 2024 by 24.157.36.115. cline resistance genes tetL and tet45 were phenotypically resistant, both with MICs of.16 m g/mL. The isolates carrying tet genes had a higher MICs when compared to the MICs of isolates without tet genes. However, since only two isolates did not carry tet genes, this observation was not statistically assessed. Sensitivity and specificity were calculated for bla1 and blaZ genes' effect on ampicil- lin and penicillin resistance. blaZ had a higher sensitivity (56%) for prediction of both ampicillin and penicillin phenotypic resistance compared to bla1 (29%) (Table 3). Of the two ampicillin susceptible isolates (MIC # 0.25 m g/mL), each carried either a bla1 or blaZ, resulting in a specificity of 50% for both of these ARGs. Since no isolates were susceptible to penicillin, bla1 and blaZ showed a 100% specificity. Isolates that carried these genes had an average ampicillin MIC of.16 m g/mL and an average penicillin MIC of. 8 m g/mL, which was the same as the average MICs of the isolates that did not carry these genes. There was no erythromycin resistance identified, yielding a sensitivity of 0% for mph (macrolide phosphotransferase) (Table 3). Further, of the 11 isolates carrying mph, only one isolate was susceptible (MIC was ,0.25 m g/mL). This resulted in a high speci- ficity of 99% (Table 3). The remaining 10 isolates carrying the mph gene were interme- diate isolates. Average MICs were statistically compared between isolates with mph and without mph using a t test to investigate the effect of this gene on the MIC. The average MIC of mph-positive isolates (2.3 m g/mL) was significantly greater than the av- erage MIC of isolates without the mph (0.4 m g/mL) (P , 0.05). The five isolates carrying the rph gene were all susceptible to rifampicin and had an MIC ,0.5 m g/mL, yielding a sensitivity of 0% and specificity of 93% (Table 3). The MICs March 2022 Volume 88 Issue 6 e02302-21 aem.asm.org 9 Mills et al. Applied and Environmental Microbiology of the isolates carrying rph (all ,0.5 m g/mL) had a lower MIC when compared to isolates without the rph gene (average MIC was 0.88 m g/mL). Point mutations and potentially impaired function of rpoB due to premature stop codons were investigated for the seven rifampicin-resistant isolates. However, the point mutations identified in the resistant iso- lates’ rpoB sequence were also present in the susceptible isolates (Supplemental Material, Table S4). Further, no resistant isolates carried previously reported rpoB point mutations associated with rifampicin resistance (16). Previous studies have not reported associations between antimicrobial resistance gene presence and phenotypic resistance of isolates from the B. cereus group species. Further, only two studies reported the presence of acquired antimicrobial resistance genes among studied isolates in combination with AST results (47, 48). Although the Bianco et al. study did not calculate the associations between presence of ARGs and phenotypic resistance, they did describe both (47). Bianco et al. identified b -lactamases in all isolates and further characterized all isolates as phenotypically resistant to penicillin G (47). Although they did not report the sensitivity and specificity, it is clear that the relationship between b -lacta- mase presence and phenotypic resistance was strong, which is similar to our findings (47). Bianco et al. identified a single isolate that carried the tetL gene and this isolate was identi- fied as intermediately resistant to tetracycline. They had not identified any isolates as resist- ant. In contrast, all tet-positive isolates from our study were found to be phenotypically re- sistant (47). The gene mphB was identified in six isolates by Bianco et al., with 4 isolates intermediately resistant to erythromycin, suggestihg that macrolide phosphotransferases may have an effect on phenotypic resistance to macrolides, similar to our findings (47). Lastly, Gly-vanR-M, Gly-vanZF-Pp, and vanR-B genes were identified in 17, 15, and 2 out of 17 isolates in the Bianco et al. study; however, all isolates were phenotypically susceptible to vancomycin, as observed in our study (47). This is not surprising, given that an isolate must have all vanM/vanB glycopeptide resistance genes present in order to confer pheno- typic resistance to vancomycin (45, 46). Multiple van genes that did not predict vancomy- cin resistance were identified also in our study. However, we did not detect van genes that have been previously associated with vancomycin resistance in Enterococcus (i.e., vanA, vanB, vanC) among the tested B. cereus isolates (46). Although we identified rph in our col- lection, it is clear it did not affect rifampicin resistance in the studied Bacillus cereus group isolates. Our findings, as well as Bianco et al. findings, show the need to further char- Downloaded from https://journals.asm.org/journal/aem on 22 November 2024 by 24.157.36.115. acterize the B. cereus group resistome and its effect on phenotypic resistance. Both of our studies investigated associations between the presence of acquired antimicrobial resistance genes and the phenotypic resistance and did not study the (over)expres- sion of detected genes, which is required to detect phenotypic resistance. Further, some of the acquired genes must be highly conserved in order to result in a pheno- typic resistance. Hence, the detection of ARG’s divergent variants may not be suffi- cient for expression of phenotypic resistance. Our findings show that more research is required to better understand the underlying mechanisms of the B. cereus group isolates’ antimicrobial resistance. MATERIALS AND METHODS Bacterial cultures. A collection of 85 B. cereus group isolates that had been previously isolated from dairy foods and dairy-associated environments were included in this study (8, 33, 34). A list of all isolates used in this study and their corresponding metadata is available in Supplemental Material, Table S1. Isolates were resuscitated from cryo stocks preserved at 280°C by streaking a loopful of each cryo stock onto brain heart infusion (BHI) agar (BD Difco) and incubating a streaked plate for 15–18 h at 32°C. After completed incubation, a single isolated colony was sub-streaked onto a fresh BHI plate and incubated at the same conditions as outlined above. Subsequently, five isolated colonies were collected using a ster- ile loop and inoculated into 5 mL of BHI broth. Inoculated broths were incubated at 32°C for 15–18 h (49). The culture adjusted to 1–2 108 was used for a disk diffusion assay described in the following section. Disk diffusion assay. Disk diffusion assay was performed on each isolate by following the M100 Clinical and Laboratory Standards Institute (CLSI) guidelines (49). A total of nine antimicrobials applied on sterile disks were tested: ampicillin (10 m g/disk), ceftriaxone (30 m g/disk), ciprofloxacin (5 m g/disk), erythromycin (15 m g/disk), gentamicin (10 m g/disk), rifampicin (5 m g/disk), tetracycline (30 m g/disk), tri- methoprim and sulfamethoxazole (1.25 1 23.75 m g/disk), and vancomycin (30 m g/disk) (49). Disks were produced by applying an antibiotic onto a sterile disk to achieve the defined mass or were purchased March 2022 Volume 88 Issue 6 e02302-21 aem.asm.org 10 Resistance of Bacillus cereus Group Isolates Applied and Environmental Microbiology pre-made (HardyDisk AST, Hardy Diagnostics). Ampicillin, erythromycin, gentamicin, and rifampicin (Hardy Diagnostics) antibiotics were applied onto disks in the lab by preparing stock concentrations and aseptically applying 20 m l of each antibiotic onto a disk to achieve the target mass of antibiotic per disk. Disks were first let dry in a biosafety cabinet for 20 min and were then stored at 220°C until use. Ceftriaxone, ciprofloxacin, tetracycline, trimethoprim-sulfamethoxazole, and vancomycin antibiotics were purchased pre-applied on the disks and were stored at 220°C until use. Bacterial cultures prepared as described in the previous section were streaked in a lawn onto Muller Hinton (MH) agar (Becton, Dickinson) using a sterile cotton swab (Medline Industries, Inc.) (49). Antimicrobial disks were placed onto the inoculated agar within 15 min of inoculation using sterile forceps (49). A maxi- mum three disks were applied on each individual agar plate to avoid overlaps in zones of inhibition that could compromise the accuracy of zone measurement. Inoculated plates with antibiotic disks were incubated at 35°C for 16–20 h (49). Bacillus cereus type strain ATCC 14579 was included in each batch of tests for quality control. An uninoculated MH agar was also included in each batch of tests to control for medium sterility. Inhibition zones were measured in millimeters using a ruler and interpreted using the guidelines and recommendations from CLSI M100 Staphylococcus spp. breakpoints (49) and guidelines were fol- lowed in cases when it was difficult to interpret zones of inhibition due to unclear edges (50). Examples include cases when some colonies grew within the zone of inhibition, when double zones of inhibition were observed, and when the antibiotic trimethoprim-sulfamethoxazole produced atypical and chal- lenging to interpret inhibition zones (50). Broth microdilution assay. Broth microdilution assays were performed on each isolate following the CLSI M45:ED3 guideline (32). A total of 18 antimicrobials were tested: ampicillin (0.12-16 m g/mL), cef- triaxone (8-64 m g/mL), ciprofloxacin (0.5-2 m g/mL), clindamycin (0.12-2 m g/mL), daptomycin (0.25-8 m g/ mL), erythromycin (0.25-4 m g/mL), gatifloxacin (1-8 m g/mL), gentamicin (2-500 m g/mL), levofloxacin (0.25-8 m g/mL), linezolid (0.5-8 m g/mL), oxacillin 1 2% NaCl (0.25-8 m g/mL), penicillin (0.06-8 m g/mL), quinupristin & dalfopristin (0.12-4 m g/mL), rifampicin (0.5-4 m g/mL), streptomycin (1000 m g/mL), tetracy- cline (2-16 m g/mL), trimethoprim & sulfamethoxazole (0.5/9.5-4/76 m g/mL), and vancomycin (1-64 m g/ mL). The Sensititre GPN3F 96-well plates (Thermo Scientific) pre-loaded with antibiotics were used for broth microdilution testing. Bacillus cereus group isolates’ inocula were prepared from overnight cultures (see “Bacterial cultures” section) by first adjusting the culture concentration to 1–2 108 CFU/mL using a spectrophotometer (Eppendorf BioPhotometer 6131). A previously established OD-CFU standard curve was used to estimate the CFU/mL based on the OD reading. The adjusted inoculum was then further diluted to achieve ;5 105 CFU/mL and dispensed into wells of a microtiter plate, 50 m L per well. Each plate included a positive control (no antibiotic added) and a negative control (just MH broth, no culture added). Inoculated plates were covered with a sealing tape provided with the Sensititre plates and incu- bated at 35°C for 18–24 h. To verify the inoculum concentration, dilutions of each inoculum were spread plated onto BHI agar and incubated at 30°C for 18–24 h. The plates were counted to ensure that the con- centration of each inoculum loaded into the Sensititre plates were between 105 and 106 CFU/mL. MICs were determined based on the guidelines and recommendations from CLSI. CLSI M07-A9 guideline was utilized in instances of unclear growth interpretations (51). This commonly included cases when a well had a very small amount of bacterial growth. In such cases, the bacterial pellets were measured in millimeters Downloaded from https://journals.asm.org/journal/aem on 22 November 2024 by 24.157.36.115. using a ruler and considered valid growth only if the pellet button was $ 2 mm (51). The MICs for antibiotics ampicillin, ciprofloxacin, clindamycin, erythromycin, gentamicin, levofloxacin, penicillin, rifampicin, tetracy- cline, trimethoprim-sulfamethoxazole, and vancomycin were interpreted with CLSI M45:ED3 Bacillus spp. guidelines (38). Linezolid breakpoints were not defined for Bacillus spp. in CLSI M45; hence, EUCAST v12.0 Bacillus spp. breakpoints were applied (21). The remaining antibiotics, ceftriaxone, gatifloxacin, oxacillin 12% NaCl, quinupristin-dalfopristin, daptomycin, and streptomycin did not have clinical breakpoints defined for Bacillus spp. in either CLSI M45:ED3 or EUCAST v12.0. ECOFFinder of EUCAST was attempted to be used to determine ecological cutoff values (ECVs), however our data distributions did not meet the criteria for deter- mination of EUCAST ECVs (40, 41). Comparative analysis of disk diffusion and broth microdilution results. The results for nine anti- biotics (i.e., ampicillin, ceftriaxone, ciprofloxacin, erythromycin, gentamicin, rifampicin, tetracycline, tri- methoprim-sulfamethoxazole, and vancomycin) analyzed with disk diffusion and broth microdilution assays were compared to investigate the correlation between zones of inhibition and MICs. This analysis was visualized via scatterplots, plotted in R and R Studio v4.0.4 using packages base v4.0.4, graphics v4.0.4, ggplot v3.3.3, grDevices v4.0.4, methods v4.0.4, stats v4.0.4, and scales v1.1., and R2 was calcu- lated to quantify the correlations between the results obtained by these two methods (52). Further, minor, major, and very major errors, and categorical agreement were calculated as outlined by Patel et al. (53). Errors were calculated to assess the reliability of disk diffusion results interpreted with CLSI M100 Staphylococcus spp. breakpoints compared to the results obtained using the gold standard method, broth microdilution, interpreted with CLSI M45 Bacillus spp. breakpoints. This analysis was applied to the seven antibiotics (i.e., ampicillin, ciprofloxacin, erythromycin, gentamicin, rifampicin, tetracycline, and tri- methoprim-sulfamethoxazole) that had defined breakpoints in CLSI M100 Staphylococcus spp. and CLSI M45 Bacillus spp. guidelines. Genotyping, phylogenetic analysis, and detection of antimicrobial resistance genes using whole-genome sequencing. Sequencing reads for the 85 Bacillus cereus group isolates that had been previously sequenced were obtained from the NCBI SRA database (8, 33, 34). Sequence accession numbers are listed in the Supplemental Material, Table S1. The quality of sequences was examined with FASTQC v0.11.8 using default settings (54). Poor quality bases and adaptors were removed with Trimmomatic v0.39 using default settings and Nextera PE adapter sequences (55). Trimmed sequences were then March 2022 Volume 88 Issue 6 e02302-21 aem.asm.org 11 Mills et al. Applied and Environmental Microbiology assembled using SPAdes v3.15 with the kmer sizes of 99 and 127 and the --careful option (56). Assembled genomes were checked for quality using QUAST v5.0.2 using the default parameters (57). Draft genomes and processed reads were analyzed using ABRIcate v1.01 and ARIBA v2.14.6, respec- tively, to detect the presence of acquired resistance genes (58, 59). MEGARes 2.0 and ResFinder 4.1 data- bases were used with both programs. Gene presence was determined with each program using their re- spective default $ 90% percent coverage and identity. Point mutations in rpoB were analyzed through creating a consensus alignment of all 85 isolates and tracking amino acid changes. BTyper3 v3.3.1 was used for taxonomic identification, genotyping (i.e., panC, rpoB, MLST typing) and identification of virulence genes using default settings (60, 61). PROKKA v1.14.6 was used to annotate genomes and produce GFF files that were then used for the analysis of pangenome with Roary v3.11.2 (62, 63). The core genome alignment produced by Roary was used for phylogenetic inference with RAxML v8.2.12 (63, 64). RAxML was run with 1,000 bootstrap repetitions and the tree with the highest likelihood was used for visualization of phylogenetic relationships among isolates. The code for the bio- informatic analysis workflow outlined above is available at https://github.com/EmmaMills101/Bacillus -Cereus-Workflow. Data visualization and statistical analyses. Distributions of disk diffusion zones of inhibition and broth microdilution MICs for each antibiotic were plotted in R and R Studio v4.0.4 using packages base v4.0.4, graphics v4.0.4, ggplot v3.3.3, grDevices v4.0.4, methods v4.0.4, and stats v4.0.4 (58). Phylogenetic tree, sequence types, virulence genes, antibiotic susceptibility testing results, and ARGs were visualized using iTOL v5 (65). The average MICs of select antibiotics were compared using a t test with 5% significance. The sensi- tivity and specificity for predicting phenotypic antibiotic resistance based on detected ARGs were calculated using the guidelines outlined in Trevethan, 2017 (66). SUPPLEMENTAL MATERIAL Supplemental material is available online only. SUPPLEMENTAL FILE 1, PDF file, 1.6 MB. ACKNOWLEDGMENTS This work was supported by the Penn State College of Agricultural Sciences Undergraduate research grants awarded to Emma Mills and Erin Sullivan, and by the USDA National Institute of Food and Agriculture Hatch Appropriations under Project #PEN04646 and Accession #1015787. REFERENCES 1. Carroll LM, Wiedmann M, Mukherjee M, Nicholas DC, Mingle LA, Dumas NB, poisoning varies according to phylogenetic affiliation (Groups I to VII) Cole JA, Kovac J. 2019. Characterization of emetic and diarrheal bacillus cer- rather than species affiliation. J Clin Microbiol 48:3388–3391. https://doi Downloaded from https://journals.asm.org/journal/aem on 22 November 2024 by 24.157.36.115. eus strains from a 2016 foodborne outbreak using whole-genome sequenc-.org/10.1128/JCM.00921-10. ing: addressing the microbiological, epidemiological, and bioinformatic chal- 10. Dierick K, Van Coillie E, Swiecicka I, Meyfroidt G, Devlieger H, Meulemans lenges. Front Microbiol 10. https://doi.org/10.3389/fmicb.2019.00144. A, Hoedemaekers G, Fourie L, Heyndrickx M, Mahillon J. 2005. Fatal family 2. Owusu-Kwarteng J, Wuni A, Akabanda F, Tano-Debrah K, Jespersen L. outbreak of Bacillus cereus-associated food poisoning. J Clin Microbiol 43: 2017. Prevalence, virulence factor genes and antibiotic resistance of Bacil- 4277–4279. https://doi.org/10.1128/JCM.43.8.4277-4279.2005. lus cereus sensu lato isolated from dairy farms and traditional dairy prod- 11. Naranjo M, Denayer S, Botteldoorn N, Delbrassinne L, Veys J, Waegenaere ucts. BMC Microbiol 17:65. https://doi.org/10.1186/s12866-017-0975-9. J, Sirtaine N, Driesen RB, Sipido KR, Mahillon J, Dierick K. 2011. Sudden 3. Scallan E, Hoekstra RM, Angulo FJ, Tauxe RV, Widdowson M-A, Roy SL, Jones death of a young adult associated with Bacillus cereus food poisoning. J JL, Griffin PM. 2011. Foodborne illness acquired in the United States—major Clin Microbiol 49:4379–4381. https://doi.org/10.1128/JCM.05129-11. pathogens. Emerg Infect Dis 17:7–15. https://doi.org/10.3201/eid1701.p11101. 12. Colaco CMG, Basile K, Draper J, Ferguson PE. 2021. Fulminant Bacillus cer- 4. Kim Y-J, Kim H-S, Kim K-Y, Chon J-W, Kim D-H, Seo K-H. 2016. High occur- eus food poisoning with fatal multi-organ failure. BMJ Case Rep 14: rence rate and contamination level of Bacillus cereus in organic vegeta- e238716. https://doi.org/10.1136/bcr-2020-238716. bles on sale in retail markets. Foodborne Pathog Dis 13:656–660. https:// 13. Glasset B, Herbin S, Granier SA, Cavalié L, Lafeuille E, Guérin C, Ruimy R, doi.org/10.1089/fpd.2016.2163. Casagrande-Magne F, Levast M, Chautemps N, Decousser J-W, Belotti L, 5. Kotzekidou P. 2013. Microbiological examination of ready-to-eat foods Pelloux I, Robert J, Brisabois A, Ramarao N. 2018. Bacillus cereus, a serious and ready-to-bake frozen pastries from university canteens. Food Micro- cause of nosocomial infections: Epidemiologic and genetic survey. PLoS biol 34:337–343. https://doi.org/10.1016/j.fm.2013.01.005. One 13:e0194346. https://doi.org/10.1371/journal.pone.0194346. 6. Carroll LM, Wiedmann M, Kovac J. 2020. Proposal of a taxonomic nomen- 14. Veysseyre F, Fourcade C, Lavigne J-P, Sotto A. 2015. Bacillus cereus infec- clature for the Bacillus cereus group which reconciles genomic definitions tion: 57 case patients and a literature review. Med Mal Infect 45:436–440. of bacterial species with clinical and industrial phenotypes. mBio 11: https://doi.org/10.1016/j.medmal.2015.09.011. e00034-20. https://doi.org/10.1128/mBio.00034-20. 15. Yamada K, Shigemi H, Suzuki K, Yasutomi M, Iwasaki H, Ohshima Y. 2019. 7. Carroll LM, Cheng RA, Kovac J. 2020. No assembly required: Using BTyper3 Successful management of a Bacillus cereus catheter-related bloodstream to assess the congruency of a proposed taxonomic framework for the Ba- infection outbreak in the pediatric ward of our facility. J Infect Chemother cillus cereus group with historical typing methods. Front Microbiol 11: 25:873–879. https://doi.org/10.1016/j.jiac.2019.04.013. 580691. https://doi.org/10.3389/fmicb.2020.580691. 16. Saikia L, Gogoi N, Das PP, Sarmah A, Punam K, Mahanta B, Bora S, Bora R. 8. Miller RA, Jian J, Beno SM, Wiedmann M, Kovac J. 2018. Intraclade variability 2019. Bacillus cereus-attributable primary cutaneous anthrax-like infec- in toxin production and cytotoxicity of Bacillus cereus group type strains and tion in newborn infants, India. Emerg Infect Dis 25:1261–1270. https://doi dairy-associated isolates. Appl Environ Microbiol 84:e02479-17. https://doi.org/10.3201/eid2507.181493..org/10.1128/AEM.02479-17. 17. Gargis AS, McLaughlin HP, Conley AB, Lascols C, Michel PA, Gee JE, 9. Guinebretière M-H, Velge P, Couvert O, Carlin F, Debuyser M-L, Nguyen- Marston CK, Kolton CB, Rodriguez-R LM, Hoffmaster AR, Weigel LM, Sue The C. 2010. Ability of Bacillus cereus group strains to cause food D. 2018. Analysis of whole-genome sequences for the prediction of March 2022 Volume 88 Issue 6 e02302-21 aem.asm.org 12 Resistance of Bacillus cereus Group Isolates Applied and Environmental Microbiology penicillin resistance and b -Lactamase activity in Bacillus anthracis. mSys- 35. Dietrich R, Jessberger N, Ehling-Schulz M, Märtlbauer E, Granum PE. 2021. tems 3:e00154-18. https://doi.org/10.1128/mSystems.00154-18. The food poisoning toxins of Bacillus cereus. Toxins 13:98. https://doi.org/ 18. Aygun FD, Aygun F, Cam H. 2016. Successful treatment of Bacillus cereus 10.3390/toxins13020098. bacteremia in a patient with propionic acidemia. Case Rep Pediatr 2016: 36. Yu S, Yu P, Wang J, Li C, Guo H, Liu C, Kong L, Yu L, Wu S, Lei T, Chen M, Zeng 6380929. https://doi.org/10.1155/2016/6380929. H, Pang R, Zhang Y, Wei X, Zhang J, Wu Q, Ding Y. 2020. A study on preva- 19. Ikeda M, Yagihara Y, Tatsuno K, Okazaki M, Okugawa S, Moriya K. 2015. lence and characterization of Bacillus cereus in ready-to-eat foods in China. Clinical characteristics and antimicrobial susceptibility of Bacillus cereus Front Microbiol 10:3043. https://doi.org/10.3389/fmicb.2019.03043. blood stream infections. Ann Clin Microbiol Antimicrob 14:43. https://doi 37. Yu P, Yu S, Wang J, Guo H, Zhang Y, Liao X, Zhang J, Wu S, Gu Q, Xue L,.org/10.1186/s12941-015-0104-2. Zeng H, Pang R, Lei T, Zhang J, Wu Q, Ding Y. 2019. Bacillus cereus isolated 20. Schaefer G, Campbell W, Jenks J, Beesley C, Katsivas T, Hoffmaster AR, Mehta from vegetables in China: Incidence, genetic diversity, virulence genes, SR, Reed S. 2016. Persistent Bacillus cereus bacteremia in 3 persons who inject and antimicrobial resistance. Front Microbiol 10:948. https://doi.org/10 drugs, San Diego, California, USA. Emerg Infect Dis 22:1621–1623. https://doi.3389/fmicb.2019.00948..org/10.3201/eid2209.150647. 38. Hindler J, Richter S, Bernard K, Bodeis-Jones S, Castanheira M, Citron DM, 21. 2022. EUCAST: Clinical breakpoints and dosing of antibiotics v12.0. Eur Couturier MR, Fritsche TR, Humphries RM, Jorgensen JH, Killian SB, Kohner P, Comm Antimicrob Susceptibility Test - EUCAST 202. https://www.eucast McDermott P, Patel S. 2016. CLSI M45 ED3:2016 methods for antimicrobial.org/clinical_breakpoints. dilution and disk susceptibility testing of infrequently isolated or fastidious 22. Thompson MK, Keithly ME, Harp J, Cook PD, Jagessar KL, Sulikowski GA, bacteria. Malvern, PA: Clinical and Laboratory Standards Institute. Armstrong RN. 2013. Structural and chemical aspects of resistance to the 39. Kassar R, Hachem R, Jiang Y, Chaftari A-M, Raad I. 2009. Management of antibiotic fosfomycin conferred by FosB from Bacillus cereus. Biochemistry Bacillus bacteremia: The need for catheter removal. Medicine 88:279–283. 52:7350–7362. https://doi.org/10.1021/bi4009648. https://doi.org/10.1097/MD.0b013e3181b7c64a. 23. Karsisiotis AI, Damblon CF, Roberts GCK. 2013. Solution structures of the 40. EUCAST. 2020. EUCAST: ECOFFinder program. https://www.eucast.org/ Bacillus cereus metallo- b -lactamase BcII and its complex with the broad eucast_news/news_singleview/?tx_ttnews%5Btt_news%5D=355% spectrum inhibitor R-thiomandelic acid. Biochem J 456:397–407. https:// 26cHash=c5de09d6e0956b53114e45958f784eb1. doi.org/10.1042/BJ20131003. 41. EUCAST. 2019. Standard operating procedure MIC distributions and the 24. Savic D, Miljkovic-Selimovic B, Lepšanovic Z, Tambur Z, Konstantinovic S, setting of epidemiological cut- off (ECOFF) values v10.1. EUCAST. https:// Stankovic N, Ristanovic E. 2016. Antimicrobial susceptibility and b -lacta- www.eucast.org/fileadmin/src/media/PDFs/EUCAST_files/EUCAST_SOPs/ mase production in Bacillus cereus isolates from stool of patients, food 2021/EUCAST_SOP_10.2_MIC_distributions_and_epidemiological_cut- and environment samples. Vojnosanit Pregl 73:904–909. https://doi.org/ off_value__ECOFF__setting_20211202.pdf. 10.2298/VSP150415134S. 42. Premkrishnan BNV, Heinle CE, Uchida A, Purbojati RW, Kushwaha KK, 25. Shimoyama Y, Umegaki O, Ooi Y, Agui T, Kadono N, Minami T. 2017. Bacil- Putra A, Santhi PS, Khoo BWY, Wong A, Vettath VK, Drautz-Moses DI, lus cereus pneumonia in an immunocompetent patient: a case report. JA Junqueira ACM, Schuster SC. 2021. The genomic characterisation and comparison of Bacillus cereus strains isolated from indoor air. Gut Pathog Clin Rep 3:25. https://doi.org/10.1186/s40981-017-0096-3. 26. Katsuya H, Takata T, Ishikawa T, Sasaki H, Ishitsuka K, Takamatsu Y, 13:6. https://doi.org/10.1186/s13099-021-00399-4. 43. The Comprehensive Antibiotic Resistance Database. https://card.mcmaster Tamura K. 2009. A patient with acute myeloid leukemia who developed.ca/ontology/39312. Accessed on February 9 2021. fatal pneumonia caused by carbapenem-resistant Bacillus cereus. J Infect 44. Rasia RM, Vila AJ. 2004. Structural determinants of substrate binding to Chemother 15:39–41. https://doi.org/10.1007/s10156-008-0654-8. Bacillus cereus metallo- b -lactamase. J Biol Chem 279:26046–26051. 27. Zhang Y, Chen M, Yu P, Yu S, Wang J, Guo H, Zhang J, Zhou H, Chen M, https://doi.org/10.1074/jbc.M311373200. Zeng H, Wu S, Pang R, Ye Q, Xue L, Zhang S, Li Y, Zhang J, Wu Q, Ding Y. 45. Arthur M, Molinas C, Courvalin P. 1992. The VanS-VanR two-component 2020. Prevalence, virulence feature, antibiotic resistance and MLST typing regulatory system controls synthesis of depsipeptide peptidoglycan pre- of Bacillus cereus isolated from retail aquatic products in China. Front cursors in Enterococcus faecium BM4147. J Bacteriol 174:2582–2591. Microbiol. https://doi.org/10.1128/jb.174.8.2582-2591.1992. 28. Shawish R, Tarabees R. 2017. Prevalence and antimicrobial resistance of Downloaded from https://journals.asm.org/journal/aem on 22 November 2024 by 24.157.36.115. 46. Patel R, Uhl JR, Kohner P, Hopkins MK, Cockerill FR. 1997. Multiplex PCR Bacillus cereus isolated from beef products in Egypt. Open Vet J 7: detection of vanA, vanB, vanC-1, and vanC-2/3 genes in enterococci. J Clin 337–341. https://doi.org/10.4314/ovj.v7i4.9. Microbiol 35:703–707. https://doi.org/10.1128/jcm.35.3.703-707.1997. 29. Fiedler G, Schneider C, Igbinosa EO, Kabisch J, Brinks E, Becker B, Stoll DA, 47. Bianco A, Capozzi L, Monno MR, Del Sambro L, Manzulli V, Pesole G, Cho G-S, Huch M, Franz CMAP. 2019. Antibiotics resistance and toxin pro- Loconsole D, Parisi A. 2021. Characterization of Bacillus cereus group isolates files of Bacillus cereus-group isolates from fresh vegetables from German from human bacteremia by whole-genome sequencing. Front Microbiol 11: retail markets. BMC Microbiol 19:250. https://doi.org/10.1186/s12866-019 3273. -1632-2. 48. Zhu K, Hölzel CS, Cui Y, Mayer R, Wang Y, Dietrich R, Didier A, Bassitta R, 30. Zhao S, Tyson GH, Chen Y, Li C, Mukherjee S, Young S, Lam C, Folster JP, Märtlbauer E, Ding S. 2016. Probiotic Bacillus cereus strains, a potential Whichard JM, McDermott PF. 2016. Whole-genome sequencing analysis risk for public health in China. Front Microbiol 7:718. https://doi.org/10 accurately predicts antimicrobial resistance phenotypes in Campylobacter.3389/fmicb.2016.00718. spp. Appl Environ Microbiol 82:459–466. https://doi.org/10.1128/AEM 49. Weinstein M, Lewis J, Bobenchick A, Campeau S, Cullen S, Galas M, Gold H,.02873-15. Humphries R, Kirn T, Limbago B, Mathers A, Mazzulli T, Richter S, Satlin M, 31. Carroll LM, Wiedmann M, den Bakker H, Siler J, Warchocki S, Kent D, Schuetz A, Simner P. 2021. CLSI M100 ED31:2021 Performance Standards for Lyalina S, Davis M, Sischo W, Besser T, Warnick LD, Pereira RV. 2017. Antimicrobial Susceptibility Testing, 31st ed. http://em100.edaptivedocs.net/ Whole-genome sequencing of drug-resistant Salmonella enterica isolates GetDoc.aspx?doc=CLSI%20M100%20ED31:2021&scope=user. from dairy cattle and humans in New York and Washington States reveals 50. EUCAST. 2019. Reading guide EUCAST disk diffusion method for antimicro- source and geographic associations. Appl Environ Microbiol 83:e00140- bial susceptibility testing. European Comm Antimicrob Susceptibility Test 17. https://doi.org/10.1128/AEM.00140-17. 6.0. https://www.google.com/url?sa=t&rct=j&q=&esrc=s&source=web&cd=& 32. Frenzel E, Kranzler M, Stark TD, Hofmann T, Ehling-Schulz M. 2015. The en- cad=rja&uact=8&ved=2ahUKEwiD8Pufw9f1AhU8pnIEHfQ2D5YQFnoECAQQ dospore-forming pathogen Bacillus cereus exploits a small colony variant- AQ&url=https%3A%2F%2Fwww.eucast.org%2Feucast_news%2Fnews_ based diversification strategy in response to aminoglycoside exposure. singleview%2F%3Fdebug%3D1%25253FtipUrl%25253Dhttp%25253A mBio 6:e01172-15–e01115. https://doi.org/10.1128/mBio.01172-15. %25252F%25252Fwww.escmid.org%25252Fnews_discussion%25252 33. Kovac J, Miller RA, Carroll LM, Kent DJ, Jian J, Beno SM, Wiedmann M. 2016. Fnews%25252F%25253Fdebug%25253D1%26tx_ttnews%255Btt_news Production of hemolysin BL by Bacillus cereus group isolates of dairy origin is %255D%3D35%26cHash%3D7d099ca7ac2b3f88faa828b8e9c8dcd1& associated with whole-genome phylogenetic clade. BMC Genomics 17:581. usg=AOvVaw17ym8e-_2Hl4Pzirk4_UE7. https://doi.org/10.1186/s12864-016-2883-z. 51. Adler J, Cockerill F, Dudley M, Eliopoulos G, Hardy D, Hecht D, Hindler J, Patel 34. Miller RA, Beno SM, Kent DJ, Carroll LM, Martin NH, Boor KJ, Kovac J. 2016. Ba- J, Powell M, Swenson J, Thomson R, Traczewksi M, Turnidge J, Weinstein M, cillus wiedmannii sp. nov., a psychrotolerant and cytotoxic Bacillus cereus Wikler M, Zimmer B. 2016. CLSI M07 ED09:2012 Methods for dilution antimi- group species isolated from dairy foods and dairy environments. Int J Syst crobial susceptibility tests for bacteria that grow aerobically (Approved Evol Microbiol 66:4744–4753. https://doi.org/10.1099/ijsem.0.001421. Standard, Ninth ed.). CLSI, Wayne, PA. March 2022 Volume 88 Issue 6 e02302-21 aem.asm.org 13 Mills et al. Applied and Environmental Microbiology 52. RStudio. 2015. RStudio: Integrated Development for R. RStudio (1.1.456). 60. Carroll LM, Kovac J, Miller RA, Wiedmann M. 2017. Rapid, high-through- Vienna, Austria: RStudio. https://www.rstudio.com/. put identification of anthrax-causing and emetic Bacillus cereus group ge- 53. Patel JB, Sharp S, Novak-Weekley S. 2013. Verification of antimicrobial sus- nome assemblies via BTyper, a computational tool for virulence-based ceptibility testing methods: A practical approach. Clin Microbiol Newsl classification of Bacillus cereus group isolates by using nucleotide 35:103–109. https://doi.org/10.1016/j.clinmicnews.2013.06.001. sequencing data. Appl Environ Microbiol 83. https://doi.org/10.1128/AEM 54. Babraham Bioinformatics. FastQC: A quality control tool for high throughput.00140-17. sequence data. https://www.bioinformatics.babraham.ac.uk/projects/fastqc/. 61. Carroll L. 2021. lmc297/BTyper3. https://github.com/lmc297/BTyper3. Accessed on February 9 2022. 62. Seemann T. 2014. Prokka: rapid prokaryotic genome annotation. Bioinfor- 55. Bolger AM, Lohse M, Usadel B. 2014. Trimmomatic: A flexible trimmer for matics 30:2068–2069. https://doi.org/10.1093/bioinformatics/btu153. Illumina sequence data. Bioinformatics 30:2114–2120. https://doi.org/10 63. Page AJ, Cummins CA, Hunt M, Wong VK, Reuter S, Holden MTG, Fookes.1093/bioinformatics/btu170. M, Falush D, Keane JA, Parkhill J. 2015. Roary: rapid large-scale prokaryote 56. Bankevich A, Nurk S, Antipov D, Gurevich AA, Dvorkin M, Kulikov AS, pan genome analysis. Bioinformatics 31:3691–3693. https://doi.org/10 Lesin VM, Nikolenko SI, Pham S, Prjibelski AD, Pyshkin AV, Sirotkin AV,.1093/bioinformatics/btv421. Vyahhi N, Tesler G, Alekseyev MA, Pevzner PA. 2012. SPAdes: a new ge- nome assembly algorithm and its applications to single-cell sequencing. J 64. Stamatakis A. 2014. RAxML version 8: a tool for phylogenetic analysis and Comput Biol 19:455–477. https://doi.org/10.1089/cmb.2012.0021. post-analysis of large phylogenies. Bioinformatics 30:1312–1313. https:// 57. Gurevich A, Saveliev V, Vyahhi N, Tesler G. 2013. QUAST: quality assess- doi.org/10.1093/bioinformatics/btu033. ment tool for genome assemblies. Bioinformatics 29:1072–1075. https:// 65. Letunic I, Bork P. 2016. Interactive tree of life (iTOL) v3: an online tool for doi.org/10.1093/bioinformatics/btt086. the display and annotation of phylogenetic and other trees. Nucleic Acids 58. Seemann T. 2021. tseemann/abricate. https://github.com/tseemann/abricate. Res 44:W242–245. https://doi.org/10.1093/nar/gkw290. 59. Hunt M, Mather AE, Sánchez-Busó L, Page AJ, Parkhill J, Keane JA, Harris 66. Trevethan R. 2017. Sensitivity, specificity, and predictive values: Founda- SR. 2017. ARIBA: rapid antimicrobial resistance genotyping directly from tions, pliabilities, and pitfalls in research and practice. Front Public Health sequencing reads. Microb Genom 3(10):e000131. 5:307. https://doi.org/10.3389/fpubh.2017.00307. Downloaded from https://journals.asm.org/journal/aem on 22 November 2024 by 24.157.36.115. March 2022 Volume 88 Issue 6 e02302-21 aem.asm.org 14