Moore's Introduction to the Musculoskeletal System PDF
Document Details
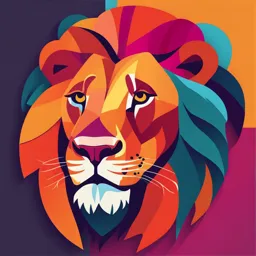
Uploaded by ReasonableMimosa
West Visayas State University
Moore
Tags
Summary
This book provides an introduction to the musculoskeletal system, covering various body systems and their functions, such as cardiovascular, nervous system, and lymphatic systems. It also explores different approaches to studying anatomy, including regional and systemic methods.
Full Transcript
CLINICAL BOX: Skeletal Muscles Cardiac Striated Muscle Smooth Muscle CLINICAL BOX: Cardiac and Smooth Muscle CARDIOVASCULAR SYSTEM Vascular Circuits Blood Vessels CLINICAL BOX: Cardiovascular System LYMPHOID SYSTEM CLINICAL BOX: Lymphoid System NERVOUS SYSTEM Central Nervous System Peripher...
CLINICAL BOX: Skeletal Muscles Cardiac Striated Muscle Smooth Muscle CLINICAL BOX: Cardiac and Smooth Muscle CARDIOVASCULAR SYSTEM Vascular Circuits Blood Vessels CLINICAL BOX: Cardiovascular System LYMPHOID SYSTEM CLINICAL BOX: Lymphoid System NERVOUS SYSTEM Central Nervous System Peripheral Nervous System CLINICAL BOX: CLINICAL Central BOX and Peripheral Nervous Systems KEY Somatic Nervous System Autonomic Nervous System TABLE 1.2. Functions of Autonomic Nervous System (ANS) MEDICAL IMAGING TECHNIQUES Anatomical Diagnostic Life Cycle Surgical Procedures Trauma Pathology Conventional Variations Radiography Procedures Computed Tomography Ultrasonography Magnetic Resonance Imaging Nuclear Medicine Imaging APPROACHES TO STUDYING ANATOMY Anatomy is the setting (structure) in which the events (functions) of life occur. This book deals mainly with functional human gross anatomy—the examination of structures of the human body that can be seen without a microscope. The three main approaches to studying anatomy are regional, systemic, and clinical (or applied), reflecting the body’s organization and the priorities and purposes for studying it. Regional Anatomy Regional anatomy (topographical anatomy) considers the organization of the human body as major parts or segments (Fig. 1.1): a main body, consisting of the head, neck, and trunk (subdivided into thorax, abdomen, back, and pelvis/perineum), and paired upper limbs and lower limbs. All the major parts may be further subdivided into areas and regions. Regional anatomy is the method of studying the body’s structure by focusing attention on a specific part (e.g., the head), area (the face), or region (the orbital or eye region); examining the arrangement and relationships of the various systemic structures (muscles, nerves, arteries, etc.) within it; and then usually continuing to study adjacent regions in an ordered sequence. ALGRAWANY FIGURE 1.1. Major parts of body and regions of lower limb. Anatomy is described relative to the anatomical position illustrated here. This book follows a regional approach, and each chapter addresses the anatomy of a major part of the body. This is the approach usually followed in anatomy courses that have a laboratory component involving dissection. When studying anatomy by this approach, it is important to routinely put the regional anatomy into the context of that of adjacent regions, of parts, and of the body as a whole. Regional anatomy also recognizes the body’s organization by layers: skin, subcutaneous tissue, and deep fascia covering the deeper structures of muscles, skeleton, and cavities, which contain viscera (internal organs). Many of these deeper structures are partially evident beneath the body’s outer covering and may be studied and examined in living individuals via surface anatomy. Surface anatomy is an essential part of the study of regional anatomy. It is integrated into each chapter of this book in “surface anatomy sections” that provide knowledge of what lies under the skin and what structures are perceptible to touch (palpable) in the living body at rest and in action. We can learn much by observing the external form and surface of the body and by observing or feeling the superficial aspects of structures beneath its surface. The aim of this method is to visualize (recall distinct mental images of) structures that confer contour to the surface or are palpable beneath it and, in clinical practice, to distinguish any unusual or abnormal findings. In short, surface anatomy requires a thorough understanding of the anatomy of the structures beneath the surface. In people with stab wounds, for example, a physician must be able to visualize the deep structures that may be injured. Knowledge of surface anatomy can also decrease the need to memorize facts because the body is always available to observe and palpate. Physical examination is the clinical application of surface anatomy. Palpation is a clinical technique, used with observation and listening for examining the body. Palpation of arterial pulses, for instance, is part of a physical examination. Radiographic and sectional imaging (radiographic anatomy) provides useful information about normal structures in living individuals, demonstrating the effect of muscle tone, body fluids and pressures, and gravity that cadaveric study does not. Diagnostic radiology reveals the effects of trauma, pathology, and aging on normal structures. In this book, most radiographic and many sectional images are integrated into the chapters where appropriate. The medical imaging sections at the end of each chapter provide an introduction to the techniques of radiographic and sectional imaging and include series of sectional images that apply to the chapter. Endoscopic techniques (using a flexible fiber-optic device inserted into one of the body’s orifices or through a small surgical incision [“portal”] to examine internal structures, such as the interior of the stomach) also demonstrate living anatomy. The detailed and thorough learning of the three- dimensional anatomy of deep structures and their relationships is best accomplished initially by dissection. In clinical practice, surface anatomy, radiographic and sectional images, endoscopy, and your experience from studying anatomy will combine to provide you with knowledge of your patient’s anatomy. The computer is a useful adjunct in teaching regional anatomy because it facilitates learning by allowing interactivity and manipulation of two- and three-dimensional graphic models. Prosections, carefully prepared dissections for the demonstration of anatomical structures, are also useful. However, learning is most efficient and retention is highest when didactic study is combined with the experience of firsthand dissection—that is, learning by doing. During dissection, you observe, palpate, move, and sequentially reveal parts of the body. In 1770, Dr. William Hunter, a distinguished Scottish anatomist and obstetrician, stated: “Dissection alone teaches us where we may cut or inspect the living body with freedom and dispatch.” Systemic Anatomy Systemic anatomy is the study of the body’s organ systems that work together to carry out complex functions. The basic systems and the field of study or treatment of each (italics in parentheses) are as follows: The integumentary system (dermatology) consists of the skin (L. integumentum, a covering) and its appendages—hairs, nails, and sweat glands, for example—and the subcutaneous tissue just beneath it. The skin, an extensive sensory organ, forms the body’s outer, protective covering and container. The skeletal system (osteology) consists of bones and cartilage; it provides our basic shape ALGRAWANY and support for the body and is what the muscular system acts on to produce movement. It also protects vital organs such as the heart, lungs, and pelvic organs. The articular system (arthrology) consists of joints and their associated ligaments, connecting the bony parts of the skeletal system and providing the sites at which movements occur. The muscular system (myology) consists of skeletal muscles that act (contract) to move or position parts of the body (e.g., the bones that articulate at joints), or smooth and cardiac muscle that propels, expels, or controls the flow of fluids and contained substance. The nervous system (neurology) consists of the central nervous system (brain and spinal cord) and the peripheral nervous system (nerves and ganglia, together with their motor and sensory endings). The nervous system controls and coordinates the functions of the organ systems, enabling the body’s responses to and activities within its environment. The sense organs, including the olfactory organ (sense of smell), eye or visual system (ophthalmology), ear (sense of hearing and balance—otology), and gustatory organ (sense of taste), are often considered with the nervous system in systemic anatomy. The circulatory system (angiology) consists of the cardiovascular and lymphatic systems, which function in parallel to transport the body’s fluids. The cardiovascular system (cardiology) consists of the heart and blood vessels that propel and conduct blood through the body, delivering oxygen, nutrients, and hormones to cells and removing their waste products. The lymphatic system is a network of lymphatic vessels that withdraws excess tissue fluid (lymph) from the body’s interstitial (intercellular) fluid compartment, filters it through lymph nodes, and returns it to the bloodstream. The alimentary or digestive system (gastroenterology) consists of the digestive tract from the mouth to the anus, with all its associated organs and glands that function in ingestion, mastication (chewing), deglutition (swallowing), digestion, and absorption of food and the elimination of the solid waste (feces) remaining after the nutrients have been absorbed. The respiratory system (pulmonology) consists of the air passages and lungs that supply oxygen to the blood for cellular respiration and eliminate carbon dioxide from it. The diaphragm and larynx control the flow of air through the system, which may also produce tone in the larynx that is further modified by the tongue, teeth, and lips into speech. The urinary system (urology) consists of the kidneys, ureters, urinary bladder, and urethra, which filter blood and subsequently produce, transport, store, and intermittently excrete urine (liquid waste). The genital (reproductive) system (gynecology for females; andrology for males) consists of the gonads (ovaries and testes) that produce oocytes (eggs) and sperms, the ducts that transport them, and the genitalia that enable their union. After conception, the female reproductive tract nourishes and delivers the fetus. The endocrine system (endocrinology) consists of specialized structures that secrete hormones, including discrete ductless endocrine glands (such as the thyroid gland), isolated and clustered cells of the gut and blood vessel walls, and specialized nerve endings. Hormones are organic molecules that are carried by the circulatory system to distant effector cells in all parts of the body. The influence of the endocrine system is thus as broadly distributed as that of the nervous system. Hormones influence metabolism and other processes, such as the menstrual cycle, pregnancy, and parturition (childbirth). None of the systems functions in isolation. The passive skeletal and articular systems and the active muscular system collectively constitute a super system, the locomotor system or apparatus (orthopedics), because they must work together to produce locomotion of the body. Although the structures directly responsible for locomotion are the muscles, bones, joints, and ligaments of the limbs, other systems are indirectly involved as well. The brain and nerves of the nervous system stimulate them to act; the arteries and veins of the circulatory system supply oxygen and nutrients to and remove waste from these structures; and the sensory organs (especially vision and equilibrium) play important roles in directing their activities in a gravitational environment. In this chapter, an overview of several systems significant to all parts and regions of the body is provided before Chapters 2 through 9 cover regional anatomy in detail. Chapter 10 also presents systemic anatomy in reviewing the cranial nerves. Clinical Anatomy Clinical anatomy (applied anatomy) emphasizes aspects of bodily structure and function important in the practice of medicine, dentistry, and the allied health sciences. It incorporates the regional and systemic approaches to studying anatomy and stresses clinical application. Clinical anatomy often involves inverting or reversing the thought process typically followed when studying regional or systemic anatomy. For example, instead of thinking, “The action of this muscle is to... ,” clinical anatomy asks, “How would the absence of this muscle’s activity be manifest?” Instead of noting, “The... nerve provides innervation to this area of skin,” clinical anatomy asks, “Numbness in this area indicates a lesion of which nerve?” Clinical anatomy is exciting to learn because of its role in solving clinical problems. The Clinical Boxes (popularly called “blue boxes,” appearing on a blue background) throughout this book describe practical applications of anatomy. Case studies, demonstrating the application of anatomy in clinical practice, are integral parts of the clinical approach to studying anatomy. SEX AND GENDER Sex, male or female, is assigned genetically. Females have 46 chromosomes that include two X chromosomes, and males have 46 chromosomes that include both an X and a Y chromosome. There are also uncommon genetically based conditions where the number of chromosomes varies, such as Klinefelter syndrome, which includes 47 chromosomes (XXY) and Jacob syndrome, which includes 47 chromosomes (XYY). Gender identity is an individual’s intrinsic sense of their own gender, which may or may not be consistent with the sex assigned chromosomally (or genetic sex). Gender is not binary but ALGRAWANY rather a spectrum that may be expressed through appearance, personality, behaviors, and relationships or not be outwardly expressed at all. The difference between sex and gender is important for clinical practice in order to be able to understand and form a trusting relationship with patients. Clinically, gender dysphoria is the significant distress an individual may undergo due to a mismatch between their gender identity and their genetic sex. While some children see their anatomy as having a different meaning to the way society sees it, many individuals may not express related feelings and behaviors until puberty or much later. ANATOMICOMEDICAL TERMINOLOGY Anatomical terminology introduces and makes up a large part of medical terminology. To be understood, you must express yourself clearly, using the proper terms in the correct way. Although you are familiar with common, colloquial terms for parts and regions of the body, you must learn the international anatomical terminology (e.g., axillary fossa instead of armpit and clavicle instead of collarbone) that enables precise communication among health care professionals and scientists worldwide. Health professionals must also know the common and colloquial terms people are likely to use when they describe their complaints. Furthermore, you must be able to use terms people will understand when explaining their medical problems to them. The terminology in this book conforms to the new International Anatomical Terminology. Terminologia Anatomica (TA) and Terminologia Embryologica (TE) list terms both in Latin and as English equivalents (e.g., the common shoulder muscle is musculus deltoideus in Latin and deltoid in English). Most terms in this book are English equivalents. Official terms are available at the International Federation of Associations of Anatomists website (https://www4.unifr.ch/ifaa). Unfortunately, the terminology commonly used in the clinical arena may differ from the official terminology. Because this discrepancy may be a source of confusion, this text clarifies commonly confused terms by placing the unofficial designations in parentheses when the terms are first used—for example, pharyngotympanic tube (auditory tube, eustachian tube) and internal thoracic artery (internal mammary artery). Eponyms, terms incorporating the names of people, do not conform to an international standard, provide no information about the type or location of the structures involved, and are frequently historically inaccurate in terms of identifying the original person to describe a structure or assign its function. Notwithstanding, commonly used eponyms appear in parentheses throughout the book when these terms are first introduced—such as sternal angle (angle of Louis)—since you will surely encounter them in your clinical years. Structure of terms. Anatomy is a descriptive science and provides names for the many structures and processes of the body. Because most terms are derived from Latin and Greek, medical language may seem difficult at first; however, as you learn the origin of terms, the words will make sense. Many terms provide information about a structure’s shape, size, location, or function or about the resemblance of one structure to another. For example, some muscles have descriptive names to indicate their main characteristics. The deltoid muscle, which covers the point of the shoulder, is triangular, like the symbol for delta, the fourth letter of the Greek alphabet. The suffix -oid means “like”; therefore, deltoid means like delta. Biceps means two-headed and triceps means three-headed. Some muscles are named according to their shape—the piriformis muscle, for example, is pear shaped (L. pirum, pear + L. forma, shape or form). Other muscles are named according to their location. The temporalis muscle is in the temporal region (temple) of the cranium (skull). In some cases, actions are used to describe muscles—for example, the levator scapulae elevates the scapula (L. for shoulder blade). If you learn the derivations of anatomical terms and consider them as you read and dissect, it will be easier to remember them. Abbreviations. Abbreviations of terms are used for brevity in medical histories and in this and other books, such as in tables of muscles, arteries, and nerves. Clinical abbreviations are used in discussions and descriptions of signs and symptoms. Learning to use these abbreviations also speeds note taking. Common anatomical and clinical abbreviations are provided in this text when the corresponding term is introduced—for example, temporomandibular joint (TMJ). Lists of common medical abbreviations can be found online. Anatomical Position All anatomical descriptions are expressed in relation to one consistent position, ensuring that descriptions are not ambiguous (Figs. 1.1 and 1.2). One must visualize this position in the mind when describing patients (or cadavers), whether they are lying on their sides, supine (recumbent, lying on the back, face upward), or prone (lying on the abdomen, face downward). The anatomical position refers to the body position as if the person were standing upright with the: Head, gaze (eyes), and toes directed anteriorly (forward) Arms adjacent to the sides with the palms facing anteriorly Lower limbs close together with the feet parallel ALGRAWANY FIGURE 1.2. Anatomical position and anatomical planes. The main planes of the body. This position is adopted globally for anatomicomedical descriptions. By using this position and appropriate terminology, you can relate any part of the body precisely to any other part. It should also be kept in mind, however, that gravity causes a downward shift of internal organs (viscera) when the upright position is assumed. Since people are typically examined in the supine position, it is often necessary to describe the position of the affected organs when supine, making specific note of this exception to the anatomical position. Anatomical Planes Anatomical descriptions are based on four imaginary planes (median, sagittal, frontal, and transverse) that intersect the body in the anatomical position (Fig. 1.2): The median plane (median sagittal plane) is the vertical anteroposterior plane passing longitudinally through the midlines of the head, neck, and trunk where it intersects the surface of the body, dividing it into right and left halves (Fig. 1.2A). Midline is often erroneously used as a synonym for the median plane. Sagittal planes are vertical planes passing through the body parallel to the median plane. “Parasagittal” is commonly used but is unnecessary because any plane parallel to and on either side of the median plane is sagittal by definition. However, a plane parallel and near to the median plane may be referred to as a paramedian plane. Frontal (coronal) planes are vertical planes passing through the body at right angles to the median plane, dividing the body into anterior (front) and posterior (back) parts (Fig. 1.2B, C). Transverse planes are horizontal planes passing through the body at right angles to the median and frontal planes, dividing the body into superior (upper) and inferior (lower) parts (Fig. 1.2C). Radiologists refer to transverse planes as transaxial, which is commonly shortened to axial planes. Since the number of sagittal, frontal, and transverse planes is unlimited, a reference point (usually a visible or palpable landmark or vertebral level) is necessary to identify the location or level of the plane, such as a “transverse plane through the umbilicus.” Sections of the head, neck, and trunk in precise frontal and transverse planes are symmetrical, passing through both the right and left members of paired structures, allowing some comparison. The main use of anatomical planes is to describe sections (Fig. 1.3): Longitudinal sections run lengthwise or parallel to the long axis of the body or of any of its parts, and the term applies regardless of the position of the body (Fig. 1.3A). Although median, sagittal, and frontal planes are the standard (most commonly used) longitudinal sections, there is a 180° range of possible longitudinal sections. Transverse sections, or cross sections, are slices of the body or its parts that are cut at right angles to the longitudinal axis of the body or of any of its parts (Fig. 1.3B). Because the long axis of the foot runs horizontally, a transverse section of the foot lies in the frontal plane (Fig. 1.2C). Oblique sections are slices of the body or any of its parts that are not cut along the previously listed anatomical planes (Fig. 1.3C). In practice, many radiographic images and anatomical sections do not lie precisely in sagittal, frontal, or transverse planes; often, they are slightly oblique. FIGURE 1.3. Sections of limbs. Sections may be obtained by anatomical sectioning or medical imaging techniques. Anatomists create sections of the body and its parts anatomically, and clinicians create them by planar imaging technologies, such as computed tomography (CT), to describe and display internal structures. Terms of Relationship and Comparison Various adjectives, arranged as pairs of opposites, describe the relationship of parts of the body or compare the position of two structures relative to each other (Fig. 1.4). Some of these terms are specific for comparisons made in the anatomical position, or with reference to the anatomical planes: ALGRAWANY FIGURE 1.4. Anatomical position and terms of relationship and comparison. These terms describe the position of one structure relative to another. Superior refers to a structure that is nearer the vertex, the topmost point of the cranium (Mediev. L., skull). Cranial relates to the cranium and is a useful directional term, meaning toward the head or cranium. Inferior refers to a structure that is situated nearer the sole of the foot. Caudal (L. cauda, tail) is a useful directional term that means toward the feet or tail region, represented in humans by the coccyx (tail bone), the small bone at the inferior (caudal) end of the vertebral column. Posterior (dorsal) denotes the back surface of the body or nearer to the back. Anterior (ventral) denotes the front surface of the body. Rostral is often used instead of anterior when describing parts of the brain; it means toward the rostrum (L., beak); however, in humans, it denotes nearer the anterior part of the head (e.g., the frontal lobe of the brain is rostral to the cerebellum). Medial is used to indicate that a structure is nearer to the median plane of the body. For example, the 5th digit of the hand (little finger) is medial to the other digits. Conversely, lateral stipulates that a structure is farther away from the median plane. The 1st digit of the hand (thumb) is lateral to the other digits. Dorsum usually refers to the superior aspect of any part that protrudes anteriorly from the body, such as the dorsum of the tongue, nose, penis, or foot. It is also used to describe the posterior surface of the hand, opposite the palm. Because the term dorsum may refer to both superior and posterior surfaces in humans, the term is easier to understand if one thinks of a quadrupedal plantigrade animal that walks on its palms and soles, such as a bear. The sole is the inferior aspect or bottom of the foot, opposite the dorsum, much of which is in contact with the ground when standing barefoot. The surface of the hands, the feet, and the digits of both corresponding to the dorsum is the dorsal surface, the surface of the hand and fingers corresponding to the palm is the palmar surface, and the surface of the foot and toes corresponding to the sole is the plantar surface. Combined terms describe intermediate positional arrangements: Inferomedial means nearer to the feet and median plane—for example, the anterior parts of the ribs run inferomedially; superolateral means nearer to the head and farther from the median plane. Other terms of relationship and comparisons are independent of the anatomical position or the anatomical planes, relating primarily to the body’s surface or its central core: Superficial, intermediate, and deep describe the position of structures relative to the surface of the body or the relationship of one structure to another underlying or overlying structure. External means outside of or farther from the center of an organ or cavity, while internal means inside or closer to the center, independent of direction. Proximal and distal are used when contrasting positions nearer to or farther from the attachment of a limb or the central aspect of a linear structure, respectively. Terms of Laterality Paired structures having right and left members (e.g., the kidneys) are bilateral, whereas those occurring on one side only (e.g., the spleen) are unilateral. Designating whether you are referring specifically to the right or left member of bilateral structures can be critical and is a good habit to begin at the outset of one’s training to become a health professional. Something occurring on the same side of the body as another structure is ipsilateral; the right thumb and right great (big) toe are ipsilateral, for example. Contralateral means occurring on the opposite side of the body relative to another structure; the right hand is contralateral to the left hand. Terms of Movement Various terms describe movements of the limbs and other parts of the body (Fig. 1.5). Most ALGRAWANY movements are defined in relationship to the anatomical position, with movements occurring within, and around axes aligned with, specific anatomical planes. While most movements occur at joints where two or more bones or cartilages articulate with one another, several nonarticulated structures exhibit movement (e.g., tongue, lips, eyelids, and hyoid bone in the neck). It is often advantageous to consider movements in antagonistic (opposing) pairs. FIGURE 1.5. Terms of movement. A–M. Terms describing movements of the limbs and other parts of the body. Most movements take place at joints, where two or more bones or cartilages articulate with one another. Flexion and extension movements generally occur in sagittal planes around a transverse axis (Fig. 1.5A, B). Flexion indicates bending or decreasing the angle between the bones or parts of the body. For joints above the knee, flexion involves movement in an anterior direction. Extension indicates straightening or increasing the angle between the bones or parts of the body. Extension usually occurs in a posterior direction. The knee joint, rotated 180° to more superior joints, is exceptional in that flexion of the knee involves posterior movement and extension involves anterior movement. Dorsiflexion describes flexion at the ankle joint, as occurs when walking uphill or lifting the front of the foot and toes off the ground (Fig. 1.5I). Plantarflexion bends the foot and toes toward the ground, as when standing on your toes. Extension of a limb or part beyond the normal limit—hyperextension (overextension)—can cause injury, such as ALGRAWANY “whiplash” (i.e., hyperextension of the neck during a rear-end automobile collision). Abduction and adduction movements generally occur in a frontal plane around an anteroposterior axis (Fig. 1.5E, G). Except for the digits, abduction means moving away from the median plane (e.g., when moving an upper limb laterally away from the side of the body) and adduction means moving toward it. In abduction of the digits (fingers or toes), the term means spreading them apart—moving the other fingers away from the neutrally positioned 3rd (middle) finger or moving the other toes away from the neutrally positioned 2nd toe. The 3rd finger and 2nd toe medially or laterally abduct away from the neutral position. Adduction of the digits is the opposite—bringing the spread fingers or toes together, toward the neutrally positioned 3rd finger or 2nd toe. Right and left lateral flexion (lateral bending) are special forms of abduction for only the neck and trunk (Fig. 1.5J). The face and upper trunk are directed anteriorly as the head and/or shoulders tilt to the right or left side, causing the midline of the body itself to become bent sideways. This is a compound movement occurring between many adjacent vertebrae. As you can see by noticing the way the thumbnail faces (laterally instead of posteriorly in the anatomical position), the thumb is rotated 90° relative to the other digits (Fig. 1.5F). Therefore, the thumb flexes and extends in the frontal plane and abducts and adducts in the sagittal plane. Circumduction is a circular movement that involves sequential flexion, abduction, extension, and adduction (or in the opposite order) in such a way that the distal end of the part moves in a circle (Fig. 1.5H). Circumduction can occur at any joint at which all the above-mentioned movements are possible (e.g., the shoulder and hip joints). Rotation involves turning or revolving a part of the body around its longitudinal axis, such as turning one’s head to face sideways (Fig. 1.5J). Medial rotation (internal rotation) brings the anterior surface of a limb closer to the median plane, whereas lateral rotation (external rotation) takes the anterior surface away from the median plane (Fig. 1.5G). Pronation and supination are the rotational movements of the forearm and hand that swing the distal end of the radius (the lateral long bone of the forearm) medially and laterally around and across the anterior aspect of the ulna (the other long bone of the forearm) while the proximal end of the radius rotates in place (Fig. 1.5D). Pronation rotates the radius medially so that the palm of the hand faces posteriorly and its dorsum faces anteriorly. When the elbow joint is flexed, pronation moves the hand so that the palm faces inferiorly (e.g., placing the palms flat on a table). Supination is the opposite rotational movement, rotating the radius laterally and uncrossing it from the ulna, returning the pronated forearm to the anatomical position. When the elbow joint is flexed, supination moves the hand so that the palm faces superiorly. (Memory device: You can hold soup in the palm of your hand when the flexed forearm is supinated but are prone [likely] to spill it if the forearm is then pronated!) Eversion moves the sole of the foot away from the median plane, turning the sole laterally (Fig. 1.5I). When the foot is fully everted, it is also dorsiflexed. Inversion moves the sole of the foot toward the median plane (facing the sole medially). When the foot is fully inverted, it is also plantarflexed. Pronation of the foot actually refers to a combination of eversion and abduction that results in lowering of the medial margin of the foot (the feet of an individual with flat feet are pronated), and supination of the foot generally implies movements resulting in raising the medial margin of the foot, a combination of inversion and adduction. Opposition is the movement by which the pad of the 1st digit (thumb) is brought to another digit pad (Fig. 1.5C). This movement is used to pinch, button a shirt, and lift a teacup by the handle. Reposition describes the movement of the 1st digit from the position of opposition back to its anatomical position. Protrusion is a movement anteriorly (forward) as in protruding the mandible (chin), lips, or tongue (Fig. 1.5L). Retrusion is a movement posteriorly (backward), as in retruding the mandible, lips, or tongue. The similar terms protraction and retraction are used most commonly for anterolateral and posteromedial movements of the scapula on the thoracic wall, causing the shoulder region to move anteriorly and posteriorly (Fig. 1.5M). Elevation raises or moves a part superiorly, as in elevating the shoulders when shrugging, the upper eyelid when opening the eye, or the tongue when pushing it up against the palate (roof of mouth) (Fig. 1.5K). Depression lowers or moves a part inferiorly, as in depressing the shoulders when standing at ease, the upper eyelid when closing the eye, or pulling the tongue away from the palate. ANATOMICAL VARIATIONS Structural variation occurs to differing degrees of severity ranging from normal to incompatible with life. Anatomical variation usually has no effect on normal function. Anatomical variations are often discovered during imaging or surgical procedures, at autopsy, or during anatomical study in individuals who had no awareness of or adverse effects from the variation. A congenital anomaly or birth defect is a variation often evident at birth or soon afterward due to aberrant form or function. Birth defects also can range from mild to severe. Although many birth defects can be treated, others are fatal. It is important to know how such variations and anomalies may influence physical examinations, diagnosis, and treatment. Anatomy textbooks describe (initially, at least) the structure of the body as it is most often observed in people, that is, the most common pattern. However, occasionally, a particular structure demonstrates so much variation within the normal range that the most common pattern is found less than half the time! Beginning students are frequently frustrated because the bodies they are examining or dissecting do not conform to the atlas or text they are using (https://www.anatomyatlases.org; Tubbs et al., 2016). Often, students ignore the variations or inadvertently damage them by attempting to produce conformity. However, you should expect anatomical variations when you dissect or inspect prosected specimens. In a random group of people, individuals obviously differ superficially from each other in physical appearance. The bones of the skeleton vary not only in size but more subtly in their basic shape and in lesser details of surface structure. A wide variation is found in the size, shape, and form of the attachments of muscles. Similarly, considerable variation exists in the patterns of branching of neurovascular structures (veins, arteries, and nerves). Veins demonstrate the greatest degree of variation and nerves the least. Individual variation must be considered in physical examination, diagnosis, and treatment. ALGRAWANY CLINICAL BOX ANATOMICAL VARIATIONS Clinically Significant Variations and Birth Defects Most descriptions in this text assume a normal range of variation. However, the frequency of variation often differs among human groups, and variations collected in one population may not apply to members of another population. Some variations, such as those occurring in the origin and course of the cystic artery to the gallbladder, are clinically significant (see Chapter 5, Abdomen). Being aware of these variations is essential in medical practice, particularly surgery. Clinically significant variations are described in clinical correlation (blue) boxes identified with an Anatomical Variation icon (at left above). Humans exhibit considerable genetic variation beyond sexual and racial differences, such as polydactyly (extra digits) or dextrocardia (heart on left). Approximately 3% of newborns show one or more significant birth defects (Moore et al., 2020). Other defects (e.g., atresia or blockage of the intestine) are not detected until symptoms occur. Discovering anatomical variations in cadavers is actually one of the many benefits of firsthand dissection because it enables students to develop an awareness of the occurrence of variations and a sense of their frequency. INTEGUMENTARY SYSTEM Because the skin (L. integumentum, a covering) is readily accessible and is one of the best indicators of general health, careful observation of it is important in physical examinations. It is considered in the differential diagnosis of almost every disease. The skin provides: Protection of the body from environmental effects, such as abrasions, fluid loss, harmful substances, ultraviolet radiation, and invading microorganisms Containment for the body’s structures (e.g., tissues and organs) and vital substances (especially extracellular fluids), preventing dehydration, which may be severe when extensive skin injuries (e.g., burns) are experienced Thermal regulation through the evaporation of sweat and/or the dilation or constriction of superficial blood vessels Sensation (e.g., pain) by way of superficial nerves and their sensory endings Synthesis and storage of vitamin D The skin, the body’s largest organ, consists of the epidermis, a superficial cellular layer, and the dermis, a deep connective tissue layer (Fig. 1.6). FIGURE 1.6. Skin. Layers of the skin and some of its specialized structures. The epidermis is a keratinized epithelium—that is, it has a tough, horny superficial layer that provides a protective outer surface overlying its regenerative and pigmented deep or basal layer. The epidermis has no blood vessels or lymphatics. The avascular epidermis is nourished by the underlying vascularized dermis. The dermis is supplied by arteries that enter its deep surface to form a cutaneous plexus of anastomosing arteries. The skin is also supplied with afferent nerve endings that are sensitive to touch, irritation (pain), and temperature. Most nerve terminals are in the dermis, but a few penetrate the epidermis. The dermis is a dense layer of interlacing collagen and elastic fibers. These fibers provide skin tone and account for the strength and toughness of skin. The dermis of animals is removed and tanned to produce leather. Although the bundles of collagen fibers in the dermis run in all directions to produce a tough felt-like tissue, in any specific location most fibers run in the same direction. The predominant pattern of collagen fibers determines the characteristic tension and wrinkle lines in the skin. The tension lines (also called cleavage lines or Langer lines) tend to spiral longitudinally in the limbs and run transversely in the neck and trunk (Fig. 1.7). Tension lines at the elbows, knees, ankles, and wrists are parallel to the transverse creases that appear when the limbs are flexed. The elastic fibers of the dermis deteriorate with age and are not replaced; consequently, in older people, the skin wrinkles and sags as it loses its elasticity. ALGRAWANY FIGURE 1.7. Tension lines of skin. The dashed lines indicate the predominant direction of the collagen fibers of the dermis. The skin also contains many specialized structures (Fig. 1.6). The deep layer of the dermis contains hair follicles, with associated smooth arrector muscles and sebaceous glands. Contraction of the arrector muscles of hairs (L. musculi arrector pili) erects the hairs, causing goose bumps. Hair follicles are generally slanted to one side, and several sebaceous glands lie on the side the hair is directed toward (“points to”) as it emerges from the skin. Thus, contraction of the arrector muscles causes the hairs to stand up straighter, thereby compressing the sebaceous glands and helping them secrete their oily product onto the skin surface. The evaporation of the watery secretion (sweat) of the sweat glands from the skin provides a thermoregulatory mechanism for heat loss (cooling). Also involved in the loss or retention of body heat are the small arteries (arterioles) within the dermis. They dilate to fill superficial capillary beds to radiate heat (skin appears red) or constrict to minimize surface heat loss (skin, especially of the lips and fingertips, appears blue). Other skin structures or derivatives include the nails (fingernails, toenails), the mammary glands, and the enamel of teeth. Located between the overlying skin (dermis) and underlying deep fascia, the subcutaneous tissue (superficial fascia) is composed mostly of loose connective tissue and stored fat and contains sweat glands, superficial blood vessels, lymphatic vessels, and cutaneous nerves (Fig. 1.6). The neurovascular structures of the integument (cutaneous nerves, superficial vessels) course in the subcutaneous tissue, distributing only their terminal branches to the skin. The subcutaneous tissue provides for most of the body’s fat storage, so its thickness varies greatly, depending on the person’s nutritional state. In addition, the distribution of subcutaneous tissue varies considerably in different sites in the same individual. Compare, for example, the relative abundance of subcutaneous tissue evident by the thickness of the fold of skin that can be pinched at the waist or thighs with the anteromedial part of the leg (the shin, the anterior border of the tibia) or the back of the hand, the latter two being nearly devoid of subcutaneous tissue. Also consider the distribution of subcutaneous tissue and fat between the sexes: In mature females, it tends to accumulate in the breasts and thighs, whereas in males, subcutaneous fat accumulates especially in the lower abdominal wall. Subcutaneous tissue participates in thermoregulation, functioning as insulation, retaining heat in the body’s core. It also provides padding that protects the skin from compression by bony prominences, such as those in the buttocks. Skin ligaments (L. retinacula cutis), numerous small fibrous bands, extend through the subcutaneous tissue and attach the deep surface of the dermis to the underlying deep fascia (Fig. 1.6). The length and density of these ligaments determine the mobility of the skin over deep structures. Where skin ligaments are longer and sparse, the skin is more mobile, such as on the back of the hand (Fig. 1.8A, B). Where ligaments are short and abundant, the skin is firmly attached to the underlying deep fascia, such as in the palms and soles (Fig. 1.8C). In dissection, removal of skin where the skin ligaments are short and abundant requires use of a sharp scalpel. The skin ligaments are long but particularly well developed in the breasts, where they form weight-bearing suspensory ligaments (see Chapter 4, Thorax). FIGURE 1.8. Skin ligaments in subcutaneous tissue. A. Estimating thickness of subcutaneous tissue. The thickness of subcutaneous tissue can be estimated as being approximately half that of a pinched fold of skin (i.e., a fold of skin includes a double thickness of subcutaneous tissue). The dorsum of the hand has relatively little subcutaneous tissue. B. Long, relatively sparse skin ligaments. Such ligaments allow the mobility of the skin demonstrated in part A. C. Short, abundant skin ligaments. The skin of the palm (like that of the sole) is firmly attached to the underlying deep fascia by relatively ALGRAWANY short, dense skin ligaments. CLINICAL BOX INTEGUMENTARY SYSTEM Skin Color Signs in Physical Diagnosis Blood flow through the superficial capillary beds of the dermis (Fig. 1.6) affects the color of skin and can provide important clues for diagnosing certain clinical conditions. When the blood is not carrying enough oxygen from the lungs, such as in a person who has stopped breathing or whose circulation is unable to send adequate amount of blood through the lungs, the skin can appear bluish (cyanotic). Cyanosis occurs because the oxygen-carrying hemoglobin of blood appears bright red when carrying oxygen (as it does in arteries and usually does in capillaries) and appears deep, purplish blue when depleted of oxygen, as it does in veins. Cyanosis is especially evident where skin is thin, such as the lips, eyelids, and deep to the transparent nails. Skin injury, exposure to excess heat, infection, inflammation, or allergic reactions may cause the superficial capillary beds to become engorged, making the skin look abnormally red, a sign called erythema. In certain liver disorders, a yellow pigment called bilirubin builds up in the blood, giving a yellow appearance to the whites of the eyes and skin, a condition called jaundice. Skin color changes are most readily observed in people with light-colored skin and may be difficult to discern in people with dark skin, in which case examination of the delicate underside of the tongue may be helpful. Skin Incisions and Scarring The skin is always under tension. In general, lacerations or incisions that parallel the tension lines usually heal well with little scarring because there is minimal disruption of collagen fibers (Fig. 1.7, lower inset). The uninterrupted fibers tend to retain the cut edges in place. However, a laceration or incision across the tension lines disrupts more collagen fibers. The disrupted lines of force cause the wound to gape (Fig. 1.7, upper inset), and it may heal with excessive (keloid) scarring. When other considerations, such as adequate exposure and access or avoidance of nerves, are not of greater importance, surgeons attempting to minimize scarring for cosmetic reasons may use surgical incisions that parallel the tension lines. Stretch Marks in Skin The collagen and elastic fibers in the dermis form a tough, flexible meshwork of tissue. Because the skin can distend considerably, a relatively small incision can be made during surgery compared with the much larger incision required to attempt the same procedure in an embalmed cadaver, which no longer exhibits elasticity. The skin can stretch and grow to accommodate gradual increases in size. However, marked and relatively fast size increases, such as the abdominal enlargement and weight gain accompanying pregnancy, can stretch the skin too much, damaging the collagen fibers in the dermis (Fig. B1.1). During pregnancy, stretch marks (L. striae gravidarum)—bands of thin wrinkled skin, initially red but later becoming purple or white—may appear on the abdomen, buttocks, thighs, and breasts. Stretch marks also form outside of pregnancy (L. striae cutis distensae) in obese individuals and in certain diseases (e.g., hypercortisolism or Cushing syndrome); they occur along with distension and loosening of the deep fascia due to protein breakdown leading to reduced cohesion between the collagen fibers. Stretch marks generally fade after pregnancy and weight loss, but they never disappear completely. FIGURE B1.1. Stretch marks. Skin Injuries and Wounds Lacerations. Accidental cuts and skin tears are superficial or deep. Superficial lacerations penetrate the epidermis and perhaps the superficial layer of the dermis; they bleed but do not interrupt the continuity of the dermis. Deep lacerations penetrate the deep layer of the dermis, extending into the subcutaneous tissue or beyond; they gape and require approximation of the cut edges of the dermis (by suturing, or stitches) to minimize scarring. Burns. Burns are caused by thermal trauma, ultraviolet or ionizing radiation, or chemical agents. Burns are classified, in increasing order of severity, based on the depth of skin injury and the need for surgical intervention. The current classification system does not use numerical designations except for fourth-degree burns (the most severe) (Fig. B1.2): Superficial burn (e.g., sunburn): Damage is limited to the epidermis; symptoms are erythema (hot red skin), pain, and edema (swelling); desquamation (peeling) of the superficial layer usually occurs several days later, but the layer is quickly replaced from the basal layer of the epidermis without significant scarring. Partial-thickness burn: Epidermis and superficial dermis are damaged with blistering (superficial partial thickness) or loss (deep partial thickness); nerve endings are damaged, making this variety the most painful; except for their most superficial parts, the sweat ALGRAWANY glands and hair follicles are not damaged and can provide the source of replacement cells for the basal layer of the epidermis along with cells from the edges of the wound; healing occurs slowly (3 weeks to several months), leaving scarring and some contracture, but it is usually complete. Full-thickness burn: The entire thickness of the skin is damaged and often the subcutaneous tissue; there is marked edema and the burned area is numb since sensory endings are destroyed; minor degree of healing may occur at the edges, but the open, ulcerated portions require skin grafting: Dead material (eschar) is removed and replaced (grafted) over the burned area with skin harvested (taken) from a nonburned location (autograft) or using skin from human cadavers or pigs or cultured or artificial skin. Fourth-degree burn: Damage extends through the entire thickness of the skin into underlying fascia, muscle, or bone; these injuries are life threatening. FIGURE B1.2. Skin burns. Burns are classified as severe if they cover 20% or more of the total body surface area (excluding superficial burns like sunburn), are complicated by trauma or inhalation injury, or are caused by chemicals or high-voltage electricity. One way to estimate the surface area affected by a burn in an adult is to apply the “rule of nines,” in which the body is divided into areas that are approximately 9% or multiples of 9% of the total body surface (Fig. B1.3). Three factors that increase the risk of death from burn injury are (1) age older than 60 years, (2) partial-thickness and full-thickness burns of over 40% body surface area, and (3) the presence of inhalation injury. FIGURE B1.3. Estimating body surface area. FASCIAS, FASCIAL COMPARTMENTS, BURSAE, AND POTENTIAL SPACES Fascias (L. fasciae) constitute the wrapping, packing, and insulating materials of the deep structures of the body. Underlying the subcutaneous tissue (superficial fascia) almost everywhere is the deep fascia (Fig. 1.9). The deep fascia is a dense, organized connective tissue layer, devoid of fat, that covers most of the body parallel to (deep to) the skin and subcutaneous tissue. Extensions from its internal surface invest deeper structures, such as individual muscles (when it may also be called epimysium—see Fig. 1.21) and neurovascular bundles, as investing fascia. Its thickness varies widely. For example, in most of the face, distinct layers of deep fascia are absent. ALGRAWANY FIGURE 1.9. Excavated section of leg. The deep fascia and fascial formations are demonstrated. In the limbs, groups of muscles with similar functions, usually sharing the same nerve supply, are located in fascial compartments. These compartments are separated by thick sheets of deep fascia, called intermuscular septa, that extend centrally from the surrounding fascial sleeve to attach to bones. These compartments may contain or direct the spread of an infection or a tumor. In a few places, the deep fascia gives attachment (origin) to the underlying muscles (although it is not usually included in lists or tables of origins and insertions); but in most places, the muscles are free to contract and glide deep to it. However, the deep fascia itself never passes freely over bone; where deep fascia contacts bone, it blends firmly with the periosteum (bone covering). The relatively unyielding deep fascia investing muscles, and especially that surrounding the fascial compartments in the limbs, limits the outward expansion of the bellies of contracting skeletal muscles. Blood is thus pushed out as the veins of the muscles and compartments are compressed. Valves within the veins allow the blood to flow only in one direction (toward the heart), preventing the backflow that might occur as the muscles relax. Thus, deep fascia, contracting muscles, and venous valves work together as a musculovenous pump to return blood to the heart, especially in the lower limbs where blood must move against the pull of gravity (see Fig. 1.26). Near certain joints (e.g., wrist and ankle), the deep fascia becomes markedly thickened, forming a retinaculum (plural = retinacula) to hold tendons in place where they cross the joint during flexion and extension, preventing them from taking a shortcut, or bow stringing, across the angle created (see Fig. 1.19). Subserous fascia, with varying amounts of fatty tissue, lies between the internal surfaces of the musculoskeletal walls and the serous membranes lining the body cavities. These are the endothoracic, endoabdominal, and endopelvic fascias; the latter two may be referred to collectively as extraperitoneal fascia. Bursae (singular = bursa; Mediev. L., a purse) are closed sacs or envelopes of serous membrane (a delicate connective tissue membrane capable of secreting fluid to lubricate a smooth internal surface). Bursae are normally collapsed. Unlike three-dimensional realized or actual spaces, these potential spaces have no depth; their walls are apposed with only a thin film of lubricating fluid between them that is secreted by the enclosing membranes. When the wall is interrupted at any point, or when a fluid is secreted or formed within them in excess, they become realized spaces; however, this condition is abnormal or pathological. Usually occurring in locations subject to friction, bursae enable one structure to move more freely over another. Subcutaneous bursae occur in the subcutaneous tissue between the skin and bony prominences, such as at the elbow or knee; subfascial bursae lie beneath deep fascia; and subtendinous bursae facilitate the movement of tendons over bone. Synovial tendon sheaths are a specialized type of elongated bursae that wrap around tendons, usually enclosing them as they traverse osseofibrous tunnels that anchor the tendons in place (Fig. 1.10A). FIGURE 1.10. Synovial tendon sheaths and bursal sacs. A. Synovial tendon sheaths. Longitudinal bursae surround tendons as they pass deep to retinacula or through fibrous digital sheaths. B. Bursal sacs. Several structures, such as the heart, lungs, abdominal viscera, and tendons, are enclosed much like this collapsed balloon encloses the fist. A thin film of ALGRAWANY lubricating fluid between the parietal and visceral layers confers mobility to the structure surrounded by the bursa within a confined compartment. The transitional folds of synovial membrane between the continuous parietal and visceral layers surrounding the connecting stalks (the wrist in this example) and/or neurovascular structures serving the surrounded mass are called mesenteries. In the case of a synovial tendon sheath, the mesentery is called a mesotendon. Bursae occasionally communicate with the synovial cavities of joints. Because they are formed by delicate, transparent serous membranes and are collapsed, bursae are not easily noticed or dissected in the laboratory. It is possible to display bursae by injecting and distending them with colored fluid. Collapsed bursal sacs surround many important organs (e.g., the heart, lungs, and abdominal viscera) and structures (e.g., portions of tendons). This configuration is much like wrapping a large but empty balloon around a structure, such as a fist (Fig. 1.10B). The object is surrounded by the two layers of the empty balloon but is not inside the balloon; the balloon itself remains empty. For an even more exact comparison, the balloon should first be filled with water and then emptied, leaving the empty balloon wet inside. In exactly this way, the heart is surrounded by— but is not inside—the pericardial sac. Each lung is surrounded by—but is not inside—a pleural sac, and the abdominal viscera are surrounded by—but are not inside—the peritoneal sac. In such cases, the inner layer of the balloon or serous sac (the one adjacent to the fist, viscus, or viscera) is called the visceral layer; the outer layer of the balloon (or the one in contact with the body wall) is called the parietal layer. Such a surrounding double layer of membranes, moistened on their apposed surfaces, confers freedom of movement on the surrounded structure when it is contained within a confined space, such as the heart within its surrounding fibrous sac (pericardium) or flexor tendons within the fibrous tunnels that hold the tendons against the bones of the fingers. CLINICAL BOX FASCIAS Fascial Planes and Surgery In living people, fascial planes (interfascial and intrafascial) are potential spaces between adjacent fascias or fascia-lined structures or within loose areolar fascias, such as the subserous fascias. Surgeons take advantage of these interfascial planes, separating structures to create spaces that allow movement and access to deeply placed structures. In some procedures, surgeons use extrapleural or extraperitoneal fascial planes, which allow them to operate outside the membranes lining the body cavities, minimizing the potential for contamination, the spread of infection, and consequent formation of adhesions (adherences) within the cavities. Unfortunately, these planes are often fused and difficult to establish or appreciate in embalmed cadavers. The Bottom Line: Integument, Fascia, and Anatomical Spaces Integumentary system: The integumentary system (the skin) consists of the epidermis, dermis, and specialized structures (hair follicles, sebaceous glands, and sweat glands). The skin: plays important roles in protection, containment, heat regulation, and sensation; synthesizes and stores vitamin D; and features tension lines, relating to the predominant direction of collagen fibers in the skin, that have implications for surgery and wound healing. Subcutaneous tissue, located beneath the dermis, contains most of the body’s fat stores. Fascias and bursae: Deep fascia is an organized connective tissue layer that completely envelops the body beneath the subcutaneous tissue underlying the skin. Extensions and modifications of the deep fascia: divide muscles into groups (intermuscular septa), invest individual muscles and neurovascular bundles (investing fascia), lie between musculoskeletal walls and the serous membranes lining body cavities (subserous fascia), and hold tendons in place during joint movements (retinacula). Bursae are closed sacs formed of serous membrane that occur in locations subject to friction; they enable one structure to move freely over another. SKELETAL SYSTEM The skeletal system may be divided into two functional parts (Fig. 1.11): The axial skeleton consists of the bones of the head (cranium or skull), neck (hyoid bone and cervical vertebrae), and trunk (ribs, sternum, vertebrae, and sacrum). The appendicular skeleton consists of the bones of the limbs, including those forming the pectoral (shoulder) and pelvic girdles. ALGRAWANY FIGURE 1.11. Skeletal system. Cartilage and Bones The skeleton is composed of cartilages and bones. Cartilage is a resilient, semirigid form of connective tissue that forms parts of the skeleton where more flexibility is required—for example, where the costal cartilages attach the ribs to the sternum. Also, the articulating surfaces (bearing surfaces) of bones participating in a synovial joint are capped with articular cartilage that provides smooth, low-friction, gliding surfaces for free movement (see Fig. 1.16A). Blood vessels do not enter cartilage (i.e., it is avascular); consequently, its cells obtain oxygen and nutrients by diffusion. The proportion of bone and cartilage in the skeleton changes as the body grows; the younger a person is, the more cartilage he or she has. The bones of a newborn are soft and flexible because they are mostly composed of cartilage. Bone, a living tissue, is a highly specialized, hard form of connective tissue that makes up most of the skeleton. Bones of the adult skeleton provide: Support for the body and its vital cavities; it is the chief supporting tissue of the body Protection for vital structures (e.g., the heart) The mechanical basis for movement (leverage) Storage for salts (e.g., calcium) A continuous supply of new blood cells (produced by the marrow in the medullary cavity of many bones) A fibrous connective tissue covering surrounds each skeletal element like a sleeve, except where articular cartilage occurs; that surrounding bones is periosteum (see Fig. 1.15), whereas that around cartilage is perichondrium. The periosteum and perichondrium nourish the external aspects of the skeletal tissue. They are capable of laying down more cartilage or bone (particularly during fracture healing) and provide the interface for attachment of tendons and ligaments. The two types of bone are compact bone and spongy (trabecular) bone. They are distinguished by the relative amount of solid matter and by the number and size of the spaces they contain (Fig. 1.12). All bones have a superficial thin layer of compact bone around a central mass of spongy bone, except where the latter is replaced by a medullary (marrow) cavity. Within the medullary cavity of adult bones, and between the spicules (trabeculae) of spongy bone, yellow (fatty) or red (blood cell and platelet forming) bone marrow—or a combination of both— is found. FIGURE 1.12. Transverse sections of femur. The shaft of a living bone is a tube of compact bone that surrounds a medullary cavity. The architecture and proportion of compact and spongy bone vary according to function. Compact bone provides strength for weight bearing. In long bones designed for rigidity and attachment of muscles and ligaments, the amount of compact bone is greatest near the middle of the shaft where the bones are liable to buckle. In addition, long bones have elevations (e.g., ridges, crests, and tubercles) that serve as buttresses (supports) where large muscles attach. Living bones have some elasticity (flexibility) and great rigidity (hardness). ALGRAWANY Classification of Bones Bones are classified according to their shape. Long bones are tubular (e.g., the humerus in the arm). Short bones are cuboidal and are found only in the tarsus (ankle) and carpus (wrist). Flat bones usually serve protective functions (e.g., the flat bones of the cranium protect the brain). Irregular bones have various shapes other than long, short, or flat (e.g., bones of the face). Sesamoid bones (e.g., the patella or knee cap) develop in certain tendons and are found where tendons cross the ends of long bones in the limbs; they protect the tendons from excessive wear and often change the angle of the tendons as they pass to their attachments. Bone Markings and Formations Bone markings appear wherever tendons, ligaments, and fascias are attached or where arteries lie adjacent to or enter bones. Other formations occur in relation to the passage of a tendon (often to direct the tendon or improve its leverage) or to control the type of movement occurring at a joint. Some of the various markings and features of bones are (Fig. 1.13): Body: the principal mass of a bone; with long bones, the shaft of the bone; with vertebrae, the anterior, weight-bearing portions between interventricular discs Capitulum: small, round, articular head (e.g., capitulum of the humerus) Condyle: rounded, knuckle-like articular area, often occurring in pairs (e.g., the lateral and medial femoral condyles) Crest: ridge of bone (e.g., the iliac crest) Epicondyle: eminence superior or adjacent to a condyle (e.g., lateral epicondyle of the humerus) Facet: smooth flat area, usually covered with cartilage, where a bone articulates with another bone (e.g., superior costal facet on the body of a vertebra for articulation with a rib) Foramen: passage through a bone (e.g., obturator foramen) Fossa: hollow or depressed area (e.g., infraspinous fossa of the scapula) Groove: elongated depression or furrow (e.g., radial groove of the humerus) Head (L. caput): large, round articular end (e.g., head of the humerus) Line: linear elevation, sometimes called a ridge (e.g., soleal line of the tibia). Malleolus: rounded process (e.g., lateral malleolus of the fibula) Neck: relatively narrow portion adjacent to the head Notch: indentation at the edge of a bone (e.g., greater sciatic notch) Process: an extension or projection serving a particular purpose, having a characteristic shape, or extending in a particular direction (e.g., articular process, spinous process, or transverse process of a vertebra) Protuberance: a bulge or projection of bone (e.g., external occipital protuberance) Shaft: the diaphysis, or body, of a long bone Spine: thorn-like process (e.g., the spine of the scapula) Trochanter: large blunt elevation (e.g., greater trochanter of the femur) Trochlea: spool-like articular process or process that acts as a pulley (e.g., trochlea of the humerus) Tubercle: small raised eminence (e.g., greater tubercle of the humerus) Tuberosity: large rounded elevation (e.g., ischial tuberosity) FIGURE 1.13. Bone markings and formations. Markings appear on bones wherever tendons, ligaments, and fascia attach. Other formations relate to joints, the passage of tendons, and the provision of increased leverage. Bone Development Most bones take many years to grow and mature. The humerus (arm bone), for example, begins to ossify at the end of the embryonic period (8 weeks); however, ossification is not complete until age 20. All bones derive from mesenchyme (embryonic connective tissue) by two different processes: intramembranous ossification (directly from mesenchyme) and endochondral ossification (from cartilage derived from mesenchyme). The histology (microscopic structure) of a bone is the same by either process (Pawlina, 2020). The two processes of bone development proceed as follows: ALGRAWANY In intramembranous ossification (membranous bone formation), mesenchymal models of bones form during the embryonic period, and direct ossification of the mesenchyme begins in the fetal period. In endochondral ossification (cartilaginous bone formation), cartilage models of the bones form from mesenchyme during the fetal period, and bone subsequently replaces most of the cartilage. A brief description of endochondral ossification helps explain how long bones grow (Fig. 1.14). The mesenchymal cells condense and differentiate into chondroblasts, dividing cells in growing cartilage tissue, thereby forming a cartilaginous bone model. In the midregion of the model, the cartilage calcifies (becomes impregnated with calcium salts), and periosteal capillaries (capillaries from the fibrous sheath surrounding the model) grow into the calcified cartilage of the bone model and supply its interior. These blood vessels, together with associated osteogenic (bone-forming) cells, form a periosteal bud (Fig. 1.14A). The capillaries initiate the primary ossification center, so named because the bone tissue it forms replaces most of the cartilage in the main body of the bone model. The shaft of a bone ossified from the primary ossification center is the diaphysis, which grows as the bone develops. FIGURE 1.14. Development and growth of a long bone. A. Ossification centers. The formation of primary and secondary ossification centers is shown. B. Growth of long bones. Growth in length occurs on both sides of the cartilaginous epiphysial plates (arrowheads). The bone formed from the primary center in the diaphysis does not fuse with that formed from the secondary centers in the epiphyses until the bone reaches its adult size. When growth ceases, the depleted epiphysial plate is replaced by a synostosis (bone-to-bone fusion), observed as an epiphysial line in radiographs and sectioned bone. Most secondary ossification centers appear in other parts of the developing bone after birth; the parts of a bone ossified from these centers are epiphyses. The chondrocytes in the middle of the epiphysis hypertrophy, and the bone matrix (extracellular substance) between them calcifies. Epiphysial arteries grow into the developing cavities with associated osteogenic cells. The flared part of the diaphysis nearest the epiphysis is the metaphysis. For growth to continue, the bone formed from the primary center in the diaphysis does not fuse with that formed from the secondary centers in the epiphyses until the bone reaches its adult size. Thus, during growth of a long bone, cartilaginous epiphysial plates intervene between the diaphysis and epiphyses (Fig. 1.14B). These growth plates are eventually replaced by bone at each of its two sides, diaphysial and epiphysial. When this occurs, bone growth ceases and the diaphysis fuses with the epiphyses. The seam formed during this fusion process (synostosis) is particularly dense and is recognizable in sectioned bone or radiographs as an epiphysial line (Fig. 1.15). The epiphysial fusion of bones occurs progressively from puberty to maturity. Ossification of short bones is similar to that of the primary ossification center of long bones, and only one short bone, the calcaneus (heel bone), develops a secondary ossification center. FIGURE 1.15. Vasculature and innervation of a long bone. Vasculature and Innervation of Bones Bones are richly supplied with blood vessels. Most apparent are the nutrient arteries (one or more per bone) that arise as independent branches of adjacent arteries outside the periosteum and pass obliquely through the compact bone of the shaft of a long bone via nutrient foramina. The nutrient artery divides in the medullary cavity into longitudinal branches that proceed toward ALGRAWANY each end, supplying the bone marrow, spongy bone, and deeper portions of the compact bone (Fig. 1.15). However, many small branches from the periosteal arteries of the periosteum are responsible for nourishment of most of the compact bone. Consequently, a bone from which the periosteum has been removed dies. Blood reaches the osteocytes (bone cells) in the compact bone by means of haversian systems or osteons (microscopic canal systems) that house small blood vessels. The ends of the bones are supplied by metaphyseal and epiphysial arteries that arise mainly from the arteries that supply the joints. In the limbs, these arteries are typically part of a peri-articular arterial plexus, which surrounds the joint, ensuring blood flow distal to the joint regardless of the position assumed by the joint. Veins accompany arteries through the nutrient foramina. Many large veins also leave through foramina near the articular ends of the bones. Bones containing red bone marrow have numerous large veins. Lymphatic vessels are also abundant in the periosteum. Nerves accompany blood vessels supplying bones. The periosteum is richly supplied with sensory nerves—periosteal nerves—that carry pain fibers. The periosteum is especially sensitive to tearing or tension, which explains the acute pain from bone fractures. Bone itself is relatively sparsely supplied with sensory endings. Within bones, vasomotor nerves cause constriction or dilation of blood vessels, regulating blood flow through the bone marrow. CLINICAL BOX BONES Accessory (Supernumerary) Bones Accessory (supernumerary) bones develop when additional ossification centers appear and form extra bones. Many bones develop from several centers of ossification, and the separate parts normally fuse. Sometimes one of these centers fails to fuse with the main bone, giving the appearance of an extra bone. Careful study shows that the apparent extra bone is a missing part of the main bone. Circumscribed areas of bone are often seen along the sutures of the cranium where the flat bones abut, particularly those related to the parietal bone (see Chapter 8, Head). These small, irregular, worm-like bones are sutural bones (wormian bones). It is important to know that accessory bones are common in the foot to avoid mistaking them for bone fragments in radiographs and other medical images. Heterotopic Bones Bones sometimes form in soft tissues where they are not normally present (e.g., in scars). Horse riders often develop heterotopic bones in their thighs (rider’s bones), probably because of chronic muscle strain resulting in small hemorrhagic (bloody) areas that undergo calcification and eventual ossification. Trauma to Bone and Bone Changes Bones are living organs that cause pain when injured, bleed when fractured, remodel in relationship to stresses placed on them, and change with age. Like other organs, bones have blood vessels, lymphatic vessels, and nerves, and they may become diseased. Unused bones, such as in a paralyzed limb, atrophy (decrease in size). Bone may be absorbed, which occurs in the mandible when teeth are extracted. Bones hypertrophy (enlarge) when they support increased weight for a long period. Trauma to a bone may break it. For the fracture to heal properly, the broken ends must be brought together, approximating their normal position. This is called reduction of a fracture. During bone healing, the surrounding fibroblasts (connective tissue cells) proliferate and secrete collagen, which forms a collar of callus to hold the bones together (Fig. B1.4). Bone remodeling occurs in the fracture area, and the callus calcifies. Eventually, the callus is resorbed and replaced by bone. After several months, little evidence of the fracture remains, especially in young people. Fractures are more common in children than in adults because of the combination of their slender, growing bones and carefree activities. Fortunately, many of these breaks are greenstick fractures (incomplete breaks caused by bending of the bones). Fractures in growing bones heal faster than those in adult bones. FIGURE B1.4. Bone fracture healing. Osteoporosis During the aging process, the organic and inorganic components of bone both decrease, often resulting in osteoporosis, a reduction in the quantity of bone, or atrophy of skeletal tissue (Fig. B1.5). Hence, the bones become brittle, lose their elasticity, and fracture easily. Bone scanning is an imaging method used to assess normal ALGRAWANY and diminished bone mass (see “Medical Imaging Techniques” at the end of this chapter). FIGURE B1.5. Osteoporosis. Sternal Puncture Examination of bone marrow provides valuable information for evaluating hematological (blood) diseases. Because it lies just beneath the skin (i.e., is subcutaneous) and is easily accessible, the sternum (breast bone) is a commonly used site for harvesting bone marrow. During a sternal puncture, a wide-bore (large- diameter) needle is inserted through the thin cortical bone into the spongy bone. A sample of red bone marrow is aspirated with a syringe for laboratory examination. Bone marrow transplantation is sometimes performed in the treatment of leukemia. If vascular collapse has occurred in a patient in shock, fluids may be rapidly infused by needle into the bone marrow of the tibia (preferred) or the sternum. Bone Growth and Assessment of Bone Age Knowledge of the sites where ossification centers occur, the times of their appearance, the rates at which they grow, and the times of fusion of the sites (times when synostosis occurs) is important in clinical medicine, forensic science, and anthropology. A general index of growth during infancy, childhood, and adolescence is indicated by bone age, as determined from radiographs, usually of the hands (Fig. B1.6). The age of a young person can be determined by studying the ossification centers in the bones. The main criteria are (1) the appearance of calcified material in ossification centers, such as the diaphysis and/or epiphyses of long bones, and (2) the narrowing and disappearance of the radiolucent (dark) line representing the epiphysial plate (absence of this line indicates that epiphysial fusion has occurred; fusion occurs at specific times for each epiphysis). The fusion of epiphyses with the diaphysis occurs 1 to 2 years earlier in females than in males. Determining bone age can be helpful in predicting adult height in early- or late-maturing adolescents. Assessment of bone age also helps establish the approximate age of human skeletal remains in medicolegal cases. FIGURE B1.6. Anterior radiographs. Right hand of a 2.5-year-old (A) and an 11-year-old (B). Effects of Disease, Diet, and Trauma on Bone Growth Some diseases produce early epiphysial fusion (ossification time) compared with what is normal for the person’s chronological age; other diseases result in delayed fusion. The growing skeleton is sensitive to relatively slight and transient illnesses and to periods of malnutrition. Proliferation of cartilage at the metaphyses slows down during starvation and illness, but the degeneration of cartilage cells in the columns continues, producing a dense line of provisional calcification. These lines later become bone with thickened trabeculae, or lines of arrested growth. Fractures involving the epiphyses may cause stunting of bone growth. Displacement and Separation of Epiphyses Without knowledge of bone growth and the appearance of bones in radiographic and other diagnostic images at various ages, a displaced epiphysial plate could be mistaken for a fracture, and separation of an epiphysis could be interpreted as a displaced piece of a fractured bone. Knowing the patient’s age and the location of epiphyses can prevent these anatomical errors. The edges of the diaphysis and epiphysis are smoothly curved in the region of the epiphysial plate. Bone fractures always leave a sharp, often uneven edge of bone. An injury that causes a fracture in an adult usually causes the displacement of an epiphysis in a child. Avascular Necrosis Loss of arterial supply to an epiphysis or other parts of a bone results in the death of bone tissue—avascular necrosis (G. nekrosis, deadness). After every fracture, small areas of adjacent bone undergo necrosis. In some fractures, avascular necrosis of a large fragment of bone may occur. A number of clinical disorders of epiphyses in children result from avascular necrosis of unknown etiology (cause). These disorders are referred to ALGRAWANY as osteochondroses. Joints Joints (articulations) are unions or junctions between two or more bones or rigid parts of the skeleton. Joints exhibit a variety of forms and functions. Some joints have no movement, such as the epiphysial plates between the epiphysis and diaphysis of a growing long bone; others allow only slight movement, such as teeth within their sockets; and some are freely movable, such as the glenohumeral (shoulder) joint. CLASSIFICATION OF JOINTS Three classes of joints are described, based on the manner or type of material by which the articulating bones are united (Fig. 1.16): 1. The articulating bones of synovial joints are united by a joint (articular) capsule (composed of an outer fibrous layer lined by a serous synovial membrane) spanning and enclosing a joint or articular cavity. The joint cavity of a synovial joint, like the knee, is a potential space that contains a small amount of lubricating synovial fluid, secreted by the synovial membrane. Inside the capsule, articular cartilage covers the articulating surfaces of the bones; all other internal surfaces are covered by synovial membrane. The bones in Figure 1.16A, normally closely apposed, have been pulled apart for demonstration, and the joint capsule has been inflated. Consequently, the normally potential joint cavity is exaggerated. The periosteum investing the participating bones external to the joint blends with the fibrous layer of the joint capsule. 2. The articulating bones of fibrous joints are united by fibrous tissue. The amount of movement occurring at a fibrous joint depends in most cases on the length of the fibers uniting the articulating bones. The sutures of the cranium are examples of fibrous joints (Fig. 1.16B). These bones are held close together, either interlocking along a wavy line or overlapping. A syndesmosis type of fibrous joint unites the bones with a sheet of fibrous tissue, either a ligament or a fibrous membrane. Consequently, this type of joint is partially movable. The interosseous membrane in the forearm is a sheet of fibrous tissue that joins the radius and ulna in a syndesmosis. A dento-alveolar syndesmosis (gomphosis or socket) is a fibrous joint in which a peg-like process fits into a socket, forming an articulation between the root of the tooth and the alveolar process of the jaw. Mobility of this joint (a loose tooth) indicates a pathological state affecting the supporting tissues of the tooth. However, microscopic movements here give us information (via the sense of proprioception) about how hard we are biting or clenching our teeth and whether we have a particle stuck between our teeth. 3. The articulating structures of cartilaginous joints are united by hyaline cartilage or fibrocartilage. In primary cartilaginous joints, or synchondroses, the bones are united by hyaline cartilage, which permits slight bending during early life. Primary cartilaginous joints are usually temporary unions, such as those present during the development of a long bone (Figs. 1.14 and 1.16C), where the bony epiphysis and the shaft are joined by an epiphysial plate. Primary cartilaginous joints permit growth in the length of a bone. When full growth is achieved, the epiphysial plate converts to bone and the epiphyses fuse with the diaphysis. Secondary cartilaginous joints, or symphyses, are strong, slightly movable joints united by fibrocartilage. The fibrocartilaginous intervertebral discs (Fig. 1.16C) between the vertebrae consist of binding connective tissue that joins the vertebrae together. Cumulatively, these joints provide strength and shock absorption as well as considerable flexibility to the vertebral column (spine). FIGURE 1.16. Classes of joints with examples. A. Synovial (freely moveable) joints. A schematic model and the more complex shoulder joint are shown. B. Fibrous joints. Three types of this class are shown. C. Cartilaginous joints. Primary and secondary cartilaginous joints are shown. Synovial joints, the most common type of joint, provide free movement between the bones they join; they are joints of locomotion, typical of nearly all limb joints. Synovial joints are usually reinforced by accessory ligaments that are either separate (extrinsic) or are a thickening of a portion of the joint capsule (intrinsic). Some synovial joints have other distinguishing features, such as a fibrocartilaginous articular disc or meniscus, which are present when the ALGRAWANY articulating surfaces of the bones are incongruous (Fig. 1.16A). The six major types of synovial joints are classified according to the shape of the articulating surfaces and/or the type of movement they permit (Fig. 1.17): 1. Plane joints permit gliding or sliding movements in the plane of the articular surfaces. The opposed surfaces of the bones are flat or almost flat, with movement limited by their tight joint capsules. Plane joints are numerous and are nearly always small. An example is the acromioclavicular joint between the acromion of the scapula and the clavicle. 2. Hinge joints permit flexion and extension only, movements that occur in one plane (sagittal) around a single axis that runs transversely; thus, hinge joints are uniaxial joints. The joint capsule of these joints is thin and lax anteriorly and posteriorly where movement occurs; however, the bones are joined by strong, laterally placed collateral ligaments. The elbow joint is a hinge joint. 3. Saddle joints permit abduction and adduction as well as flexion and extension, movements occurring around two axes at right angles to each other; thus, saddle joints are biaxial joints that allow movement in two planes, sagittal and frontal. The performance of these movements in a circular sequence (circumduction) is also possible. The opposing articular surfaces are shaped like a saddle (i.e., they are reciprocally concave and convex). The carpometacarpal joint at the base of the 1st digit (thumb) is a saddle joint. 4. Condyloid joints permit flexion and extension as well as abduction and adduction; thus, condyloid joints are also biaxial. However, movement in one plane (sagittal) is usually greater (freer) than in the other. Circumduction, more restricted than that of saddle joints, is also possible. The metacarpophalangeal joints (knuckle joints) are condyloid joints. 5. Ball and socket joints allow movement in multiple axes and planes: flexion and extension, abduction and adduction, medial and lateral rotation, and circumduction; thus, ball and socket joints are multiaxial joints. In these highly mobile joints, the spheroidal surface of one bone moves within the socket of another. The hip joint is a ball and socket joint in which the spherical head of the femur rotates within the socket formed by the acetabulum of the hip bone. 6. Pivot joints permit rotation around a central axis; thus, they are uniaxial. In these joints, a rounded process of bone rotates within a sleeve or ring. The median atlanto-axial joint is a pivot joint in which the atlas (C1 vertebra) rotates around a finger-like process, the dens of the axis (C2 vertebra), during rotation of the head. FIGURE 1.17. Six types of synovial joints. Synovial joints are classified according to the shape of their articulating surfaces and/or the type of movement they permit. JOINT VASCULATURE AND INNERVATION Joints receive blood from articular arteries that arise from the vessels around the joint. The arteries often anastomose (communicate) to form networks (peri-articular arterial anastomoses) to ensure a blood supply to and across the joint in the various positions assumed by the joint. Articular veins are communicating veins that accompany arteries (L. venae comitantes) and, like the arteries, are located in the joint capsule, mostly in the synovial membrane. Joints have a rich nerve supply provided by articular nerves with sensory nerve endings in the joint capsule. In the distal parts of the limbs (hands and feet), the articular nerves are branches of the cutaneous nerves supplying the overlying skin. However, most articular nerves are branches of nerves that supply the muscles that cross and therefore move the joint. The Hilton “law”1 (more a “rule of thumb”) indicates that the nerves supplying a joint also supply the muscles moving the joint and, added later, the skin covering their distal attachments (Ellis & Mahadevan, 2019). Articular nerves transmit sensory impulses from the joint that contribute to the sense of proprioception, which provides an awareness of movement and position of the parts of the ALGRAWANY body. The synovial membrane is relatively insensitive. Pain fibers are numerous in the fibrous layer of the joint capsule and the accessory ligaments, causing considerable pain when the joint is injured. The sensory nerve endings respond to the twisting and stretching that occurs during sports activities. CLINICAL BOX JOINTS Joints of Newborn Cranium The bones of the calvaria (skullcap) of a newborn infant’s cranium do not make full contact with each other (Fig. B1.7). At these sites, the sutures form wide areas of fibrous tissue called fontanelles. The anterior fontanelle is the most prominent; laypeople call it the “soft spot.” The fontanelles in a newborn are often felt as ridges because of the overlapping of the cranial bones by molding of the calvaria as it passes through the birth canal. Normally, the anterior fontanelle is flat. A bulging fontanelle may indicate increased intracranial pressure; however, the fontanelle normally bulges during crying. Pulsations of the fontanelle reflect the pulse of cerebral arteries. A depressed fontanelle may be observed when the neonate is dehydrated (Swartz, 2021). FIGURE B1.7. Neonatal (newborn) cranium. Degenerative Joint Disease Synovial joints are well designed to withstand wear, but heavy use over several years can cause degenerative changes. Some destruction is inevitable during such activities as jogging, which wears away the articular cartilages and sometimes erodes the underlying articulating surfaces of the bones. The normal aging of articular cartilage begins early in adult life and progresses slowly thereafter, occurring on the ends of the articulating bones, particularly those of the hip, knee, vertebral column, and hands (Salter, 1998). These irreversible degenerative changes in joints result in the articular cartilage becoming a less effective shock absorber and lubricated surface. As a result, the articulation becomes increasingly vulnerable to the repeated friction that occurs during joint movements. In some people, these changes do not produce significant symptoms; in others, they cause considerable pain. Degenerative joint disease or osteoarthritis is often accompanied by stiffness, discomfort, and pain. Osteoarthritis is common in older people and usually affects joints that support the weight of their bodies (e.g., the hips and knees). Most substances in the bloodstream, normal or pathological, easily enter the joint cavity. Similarly, traumatic infection of a joint may be followed by arthritis, inflammation of a joint, and septicemia, blood poisoning. Arthroscopy The cavity of a synovial joint can be examined by inserting a cannula and an arthroscope (a small telescope) into it. This surgical procedure—arthroscopy— enables orthopedic surgeons to examine joints for abnormalities, such as torn menisci (partial articular discs of the knee joint). Some surgical procedures can also be performed during arthroscopy (e.g., by inserting instruments through small puncture incisions). Because the opening in the joint capsule for inserting the arthroscope is small, healing is more rapid after this procedure than after traditional joint surgery. The Bottom Line: Skeletal System Cartilage and bones: The skeletal system can be divided into the axial (bones of the head, neck, and trunk) and appendicular skeletons (bones of the limbs). The skeleton itself is composed of several types of tissue: cartilage, a semirigid connective tissue; bone, a hard form of connective tissue that provides support, protection, movement, storage (of certain electrolytes), and synthesis of blood cells; and periosteum, which surrounds bones, and perichondrium, which surrounds cartilage, provide nourishment for these tissues and are the sites of new cartilage and bone formation. Two types of bone, spongy and compact, are distinguished by the amount of solid matter and the size and number of spaces they contain. Bones can be classified as long, short, flat, irregular, or sesamoid. Standard terms for specific bone markings and features are used when describing the structure of individual bones. Most bones take many years to grow. Bones grow through the processes of intramembranous ossification, in which mesenchymal bone models are formed during the embryonic and prenatal periods, and endochondral ossification, in which cartilage models are formed during the fetal period, ALGRAWANY with bone subsequently replacing most of the cartilage after birth. Joints: A joint is a union between two or more bones or rigid parts of the skeleton. Three general types of joints are recognized: fibrous, cartilaginous, and synovial. Freely moveable synovial joints are the most common type; can be classified into plane, hinge, saddle, condyloid, ball and socket, and pivot; receive their blood supply from articular arteries that often form networks; are drained by articular veins originating in the synovial membrane; and are richly innervated by articular nerves that transmit the sensation of proprioception, an awareness of movement and position of parts of the body. MUSCLE TISSUE AND MUSCULAR SYSTEM The muscular system consists of all the muscles of the body. Voluntary skeletal muscles constitute the great majority of the named muscles. All skeletal muscles are composed of one specific type of muscle tissue. However, other types of muscle tissue constitute a few named muscles (e.g., the ciliary and detrusor muscles and the arrector muscles of hairs) and form important components of the organs of other systems, including the cardiovascular, alimentary, genitourinary, integumentary, and visual systems. Types of Muscle (Muscle Tissue) Muscle cells, often called muscle fibers because they are long and narrow when relaxed, are specialized contractile cells. They are organized into tissues that move body parts or temporarily alter the shape (reduce the circumference of all or part) of internal organs. Associated connective tissue conveys nerve fibers and capillaries to the muscle cells as it binds them into bundles or fascicles. Three types of muscle are described based on distinct characteristics relating to whether it is normally willfully controlled (voluntary vs. involuntary) whether it appears striped or unstriped when viewed under a microscope (striated vs. smooth or unstriated) whether it is located in the body wall (soma) and limbs or makes up the hollow organs (viscera, e.g., the heart) of the body cavities or blood vessels (somatic vs. visceral) There are three muscle types (Table 1.1): 1. Skeletal striated muscle is voluntary somatic muscle that makes up the gross skeletal muscles that compose the muscular system, moving or stabilizing bones and other structures (e.g., the eyeballs). 2. Cardiac striated muscle is involuntary visceral muscle that forms most of the walls of the heart and adjacent parts of the great vessels, such as the aorta, and pumps blood. 3. Smooth muscle (unstr