Molecular Biology Final Edit PDF
Document Details
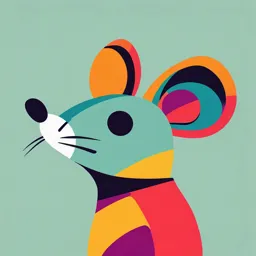
Uploaded by EnjoyableChimera
Beni-Suef University
Tags
Summary
This presentation covers fundamental concepts in molecular biology, particularly focusing on DNA technology, DNA history, and DNA structure. It also details important concepts, including DNA distribution, and related biological concepts such as DNA replication and transcription.
Full Transcript
DNA Technology DNA History Avery – Discovered that DNA is the nucleic acid that stores and transmits the genetic information from one generation to the next. Griffith – Experimented on mice and observed some harmless strains of bacteria (Diplococcus pneumonia) could cha...
DNA Technology DNA History Avery – Discovered that DNA is the nucleic acid that stores and transmits the genetic information from one generation to the next. Griffith – Experimented on mice and observed some harmless strains of bacteria (Diplococcus pneumonia) could change into harmful strains. He called this transformation. As it is found in 2 forms( Rough, Smooth) (Transformation exp.) Griffith More DNA History 2- Hershey-Chase experiment – Concluded that the genetic material in bacteria was DNA not proteins (Bacteriophage T2 virus that replicate only in bacterium via adsorption on their surface ) when the virus infects the bacterial cell, it injects its DNA, and the protein coat remains loosely attached to the bacterium Hershey Chase 3- DNA distribution By comparing amount of DNA in cells As non reproductive cells ( liver, kidney, spleen, …………) has the same amount of DNA While reproductive cell (sperms, eggs) contain half genetic material. DNA Structure (Waston & Crick) Structure of DNA DNA is a long molecule made up of units called nucleotides. Each nucleotide is made up of three parts: a 5- carbon sugar called deoxyribose, a phosphate group, and a nitrogenous base (Nitrogen Containing). The backbone of DNA is formed by sugar and phosphate groups of the nucleotide. The nitrogenous base stick out from the sides and can be joined together in any order, meaning that any sequence of bases is possible. Nitrogenous Bases There are four kinds of nitrogenous bases. They are divided into two classes: purines and pyrmidines Purines – Adenine and Guanine Pyrmidines – Cytosine and Thymine Chargaff’s Rules Chargaff discovered how the nitrogenous bases bond together. He discovered that Adenine always binds with Thymine and that Cytosine always binds with Guanine. Chargaff Prokaryotes & DNA In prokaryotes, DNA molecules are located in the cytoplasm of the cell. Most prokaryotic DNA is a single circular molecule that contains nearly all the cell’s genetic information. Eukaryotes & DNA Many eukaryotes have 1000 times as much DNA as prokaryotes. DNA is located in the nucleus in the form of chromosomes. Chromosomes are DNA wound tightly around proteins called histones. What is DNA? DNA, or deoxyribonucleic acid, is the hereditary material in humans and almost all other organisms. Most DNA is located in the cell nucleus (where it is called nuclear DNA), but a small amount of DNA can also be found in the Mitochondria (where it is called mitochondrial DNA or mtDNA). 19 DNA Stands for Deoxyribonucleic acid Made up of subunits called nucleotides Nucleotide made of: 1. Phosphate group 2. 5-carbon sugar 3. Nitrogenous base 20 The Structure of DNA DNA is composed of four nucleotides, each containing: adenine, cytosine, thymine, or guanine. The amounts of A = T, G = C, and purines = pyrimidines [Chargaff’s Rule]. DNA is a double-stranded helix with antiparallel strands [Watson and Crick]. Nucleotides in each strand are linked by 5’- 3’ phosphodiester bonds Bases on opposite strands are linked by hydrogen bonding: A with T, and G with C. 5 DNA O 3 3 O P 5 P 5 O 1 G C 3 2 4 4 2 1 3 5 O P P 5 T A 3 O O 5 P 3 P22 Chargaff’s Rules Question: If there is 30% Adenine, how much Cytosine is present? There would be 20% Cytosine Adenine (30%) = Thymine (30%) Guanine (20%) = Cytosine (20%) Therefore, 60% A-T and 40% C-G 25 An important property of DNA is that it can replicate, or make copies of itself. Each strand of DNA in the double helix can serve as a pattern for duplicating the sequence of bases. This is critical when cells divide because each new cell needs to have an exact copy of the DNA present in the old cell. 26 Replication Facts DNA has to be copied before a cell divides DNA is copied during the S or synthesis phase of interphase New cells will need identical DNA strands 27 Synthesis Phase (S phase) S phase during interphase of the cell cycle Nucleus of eukaryotes DNA replication takes place in the S phase. 28 Models of replication 1. Conservative replication would produce an entirely new DNA strand during replication. 2. Semiconservative replication would produce two DNA molecules, each of which was composed of one-half of the parental DNA along with an entirely new complementary strand. 3. Dispersive replication involved the breaking of the parental strands during replication, and somehow, a reassembly of The Central Dogma DNA encodes the information to make RNA.........and RNA molecules function together to make protein II. What is RNA and how is it different from DNA? Two big differences between DNA and RNA: 1. The sugar in DNA is deoxyribose; in RNA it is ribose 2. The nitrogenous base uracil (U) is used in RNA in place of T (they are very similar bases; in RNA U= A just like T = A.) 3. DNA is double strands while RNA is single DNA Replication During DNA replication, the DNA molecule separates into two strands, then produces two new complimentary strands following the rules of base pairing (Chargaff Rules). Each strand of double helix of DNA serves as a template, or model, for the new strand. 5’ 3’ Identical 5’ base 3’ sequences 3’ 5’ 3’ 5’ Figure 11.1 Watson/Crick proposed mechanism of DNA replication Replication Of DNA DNA Replication The copying of DNA is remarkable in its speed and accuracy. Involves unwinding the double helix and synthesizing two new strands. More than a dozen enzymes and other proteins participate in DNA replication The replication of a DNA molecule begins at special sites called origins of replication, where the two strands are separated Initiation of Replication The origin of replication in E. coli is termed oriC – origin of Chromosomal replication Important DNA sequences in oriC – AT-rich region – DnaA boxes – ATP builds up when the cell is in a rich medium, triggering DNA replication once the cell has reached a specific size. ATP competes with ADP to bind to DnaA, and the DnaA-ATP complex is able to initiate replication. Figure 11.4 Overview of bacterial DNA replication Figure 11.5 DNA sequences at the Bacterial origin of Replication Origins of Replication A eukaryotic chromosome may have hundreds or even thousands of replication origins Origin of replication Parental (template) strand 0.25 µm 1 Replication begins at specific sites where the two parental strands separate and form replication bubbles. Bubble Replication fork 2 The bubbles expand laterally, as DNA replication proceeds in both directions. 3 Eventually, the replication Daughter (new) strand bubbles fuse, and synthesis of the daughter strands is complete. Two daughter DNA molecules (a) In eukaryotes, DNA replication begins at many sites along the giant (b) In this micrograph, three replication DNA molecule of each chromosome. bubbles are visible along the DNA of a cultured Chinese hamster cell (TEM). DNA Replication Begins at Origins of Replication Two strands open forming Replication Forks (Y-shaped region) New strands grow at the forks 3’ Parental DNA Molecule 5’ Replication Fork 3’ copyright cmassengale 40 5’ DNA Replication Before new DNA strands can form, there must be RNA primers present to start the addition of new nucleotides. Primase is the enzyme that synthesizes the RNA Primer DNA polymerase can then add the new nucleotides copyright cmassengale 41 DNA Replication DNA polymerase can only add nucleotides to the 3’ end of the DNA This causes the NEW strand to be built in a 5’ to 3’ direction 5’ 3’ 5’ RNA DNA Polymerase Primer Nucleotide Direction of Replication 42 Synthesis of the New DNA Strands The Leading Strand is synthesized as a single strand from the point of origin toward the opening replication fork 5’ 3’ 5’ RNA Nucleotides DNA Polymerase Primer copyright cmassengale 43 Synthesis of the New DNA Strands The Lagging Strand is synthesized discontinuously against overall direction of replication This strand is made in MANY short segments It is replicated from the replication fork toward the origin 5’ Leading Strand 3’ 3’ 5’ DNA Polymerase RNA Primer 5’ 3’ 3’ 5’ copyright cmassengale 44 Lagging Strand Lagging Strand Segments Okazaki Fragments - series of short segments on the lagging strand Must be joined together by an enzyme Okazaki Fragment DNA Polymerase RNA Primer 5’ 3’ 3’ 5’ Lagging Strand 45 Joining of Okazaki Fragments The enzyme Ligase joins the Okazaki fragments together to make one strand DNA ligase 5’ Okazaki Fragment 1 Okazaki Fragment 2 3’ 3’ 5’ Lagging Strand 46 Mechanism of DNA Replication Nucleotides are added by complementary base pairing with the template strand During replication, new nucleotides are added to the free 3’ hydroxyl on the growing strand. The rate of elongation is about 500 nucleotides per second in bacteria and 50 per second in human cells Template strand New strand 5¢ end 3¢ end 5¢ end 3¢ end Sugar Phosphate Base DNA polymerase 3¢ end Pyrophosphate 3¢ end Nucleoside triphosphate 5¢ end 5¢ end Replication 3’ 3’ 5’ 5’ 3’ 5’ 3’ 5’ Helicase protein binds to DNA sequences called origins and unwinds DNA strands. Binding proteins prevent single strands from rewinding. Primase protein makes a short segment of RNA complementary to the DNA, a primer. Replication Overall direction 3’ of replication 3’ 5’ 5’ 3’ 5’ 3’ 5’ DNA polymerase III enzyme adds DNA nucleotides to the RNA primer. Replication Overall direction 3’ of replication 3’ 5’ 5’ 3’ 5’ 3’ 5’ DNA polymerase proofreads bases added and replaces incorrect nucleotides. Replication Overall direction 3’ of replication 3’ 5’ 5’ 3’ 5’ 3’ 5’ Leading strand synthesis continues in a 5’ to 3’ direction. Replication Overall direction 3’ of replication 3’ 5’ 5’ Okazaki fragment 3’ 3’ 5’ 5’ 3’ 5’ Leading strand synthesis continues in a 5’ to 3’ direction. Discontinuous synthesis produces 5’ to 3’ DNA segments called Okazaki fragments. Replication Overall direction 3’ of replication 3’ 5’ 5’ Okazaki fragment 3’ 3’ 5’ 5’ 3’ 5’ Leading strand synthesis continues in a 5’ to 3’ direction. Discontinuous synthesis produces 5’ to 3’ DNA segments called Okazaki fragments. Replication 3’ 3’ 5’ 5’ 3’ 5’ 3’ 5’ 3’ 5’ 3’ 5’ Leading strand synthesis continues in a 5’ to 3’ direction. Discontinuous synthesis produces 5’ to 3’ DNA segments called Okazaki fragments. Replication 3’ 3’ 5’ 5’ 3’ 5’ 3’5’ 3’5’ 3’ 5’ Leading strand synthesis continues in a 5’ to 3’ direction. Discontinuous synthesis produces 5’ to 3’ DNA segments called Okazaki fragments. Replication 3’ 3’ 5’ 5’ 3’ 5’ 3’5’ 3’5’ 3’ 5’ Exonuclease activity of DNA polymerase I removes RNA primers. Replicatio n 3’ 3’ 5’ 3’ 5’ 3’5’ 3’ 5’ Polymerase activity of DNA polymerase I fills the gaps. Ligase forms bonds between sugar-phosphate backbone. Proofreading DNA must be faithfully replicated…but mistakes occur – DNA polymerase (DNA pol) inserts the wrong nucleotide base in 1/10,000 bases DNA pol has a proofreading capability and can correct errors – Mismatch repair: ‘wrong’ inserted base can be removed – Excision repair: DNA may be damaged by chemicals, radiation, etc that may lead to mutations. Mechanism to cut out and replace with correct bases Proofreading by the 3’ 5’ exonuclease activity of DNA polymerases during DNA replication. DNA repair Replicating the Ends of DNA Molecules The ends of eukaryotic chromosomal DNA get shorter with each round of replication 5 End of parental Leading strand DNA strands Lagging strand 3 Last fragment Previous fragment RNA primer 5 Lagging strand 3 Primer removed but Removal of primers and cannot be replaced replacement with DNA with DNA because where a 3 end is available no 3 end available for DNA polymerase 5 3 Second round of replication 5 New leading strand 3 New lagging strand 5 3 Further rounds of replication Shorter and shorter daughter molecules Telomeres Eukaryotic chromosomal DNA molecules have at their ends nucleotide sequences, called telomeres, that postpone the erosion of genes near the ends of DNA molecules. Telomerases If the chromosomes of germ cells became shorter in every cell cycle essential genes would eventually be missing from the gametes they produce An enzyme called telomerase catalyzes the lengthening of telomeres in germ cells Other Proteins That Assist DNA Replication Helicase, topoisomerase, single-strand binding protein are all proteins that assist DNA replication 66 Transcription RNA molecules are produced by copying part of the nucleotide sequence of DNA into complementary sequence in RNA, a process called transcription. During transcription, RNA polymerase binds to DNA and separates the DNA strands. RNA polymerase then uses one strand of DNA as a template from which nucleotides are assembled into a strand of mRNA. RNA: DNA’s overworked cousin RNA is very similar in structure to DNA. The only differences are: 1. The sugar in RNA is slightly different (ribose instead of deoxyribose) 2. RNA has uracil (U) instead of thymine (T) 3. RNA usually hangs out in a single, These small changes in structure make RNA function very differently from its DNA cousin. 68 You already know that DNA is the “boss” of the cell. It directs all of the cell’s processes. How can it do that when it’s stuck inside the nucleus? 69 Answer: specialized messages! 1.The DNA sends its coded message from inside the nucleus to the cytoplasm through a process called transcription. 2. This message contains instructions for making a specific protein through a process called translation. 3. The protein puts the DNA’s instructions into action, either inside or outside the cell! Transcription has several steps: 1. The strands of DNA are separated by the helicase enzyme. 2. An enzyme called RNA polymerase starts adding complementary RNA nucleotides to one of the separated DNA strands. The nucleotides are joined together by the ligase enzyme. 3. When RNA polymerase reaches a “stop sign” in the DNA strand, it stops transcribing and releases the newly formed RNA strand. The RNA leaves the nucleus for the cytoplasm, where it can be used to make proteins. 4. The separated DNA strands come back together 71 RNA (Ribonucleic acid) Structure: Similar to that of DNA except: 1- it is single stranded polunucleotide chain. 2- Sugar is ribose 3- Uracil is instead of thymine There are 3 types of RNA: 1- Ribosomal RNA (rRNA) 2- Messenger RNA (mRNA) 3- Transfer RNA (tRNA) RNA are copies from DNA sequences formed by a process called “ transcription”. After transcription some modifications occur to obtain the three types of RNA. 72 Notes: 1- The synthesized RNA will have the sequence of the sense strand except for U instead of T. 2- In prokaryotic (bacteria) all types of RNA are synthesized by only one species of RNA polymerase. 3- In Eukaryotic ( mammalians), there are 3 classes of RNA polymerase a- RNA polymerase I: synthesizes the precursor of rRNA named : pre rRNA b- RNA polymerase II: synthesizes pre mRNA c- RNA polymerase III: synthesizes pre tRNA All these enzymes synthesize what is called primary transcript or immature RNAs (pre form) which by some modifications occur after transcription, will give the mature rRNA, mRNA and tRNA. 73 Characters of transcription 1- Highly specific and selective (not all DNA region) 2- Selectivity is due to signals embedded in the nucleotide sequence of DNA that instruct RNA polymerase to start and stop transcription. 3- The biochemical differentiation of tissues of an organism is due to the selectivity of the transcription 74 Transcription: DNA -> RNA Translation at ribosome Genes Protein mRNA Transcription RNA comes in three flavors Messenger RNA (mRNA): carries the genetic message from DNA in the nucleus to the cytoplasm (5% of total RNA) Transfer RNA (tRNA): uses the RNA message to construct proteins in the cytoplasm (80%) Ribosomal RNA (rRNA): ribosomes are made out of this kind of RNA. (15%). Proteins are made inside of ribosomes 1-Messenger RNA (mRNA): comprised only 5% of total cellular RNA. Function: Carry genetic information from DNA in the nucleus to ribosomes (in cystol) where it is used as template for protein biosynthesis. 2- Ribosomal RNA (rRNA): 15% of total RNA in the cells are rRNA. rRNA are found in combination with several proteins ( about 82 proteins) as component of the ribosome Which is the site of protein synthesis. In Eucaryotic ( mammals). There are 4 size types of rRNA (5S, 5.8S, 18Ss and 28S) representing 2/3 particle mass of the ribosome. 77 3- Transfer RNA (tRNA): tRNA represents 80% of total RNA in the cell. Structure: 1- amino acid attachment site or amino acid acceptor: which terminates with the triplet CCA. 2- Anticodon loop or anticodon triplet 3- D loop and T loop: contain unusual bases e.g. dihydrouracil, ribothymidine or methyl guanine Functions of tRNA: 1- transport amino acids to ribosome for protein synthesis. Each tRNA carry only one amino acid. The specific amino acid is attached enzymatically to 3' end of tRNA. 2- recognize the specified codon on mRNA to ensure the insertion of the correct amino acid in the growing polypeptide chain. This function is due to anticodon triplet which binds to codon on mRNA by base pairing. NB: Three nucleotide bases on mRNA form a codon which is then translated into specific amino acid. 78 Structure of tRNA Funtions of tRNA 79 A Ribosome (rRNA) tRNA mRNA 80 Hey look, it’s more pictures of transcription! 81 Reaction mechanism of RNA polymerase 1. RNA Pols polymerize in the 5’ to 3’ direction (rNTP added only to the 3’ end) 2. 3’ OH of chain reacts with the a PO4 of incoming rNTP, liberating pyrophosphate 3. Added ribonucleotide follows Watson-Crick pairing rules, determined by template strand 4. RNA polymerases don’t need a primer, but do need ds DNA 5. RNA polymerase lacks exonuclease activities, then can not proof-read and is much more error prone than DNA polymerase. Transcription RNA is a complementary copy from DNA template except uracil is instead of thymine. The process of RNA synthesis from DNA template is called: transcription and occurs in nucleus. The enzyme responsible for transcription is RNA polymerase Steps in RNA synthesis: (Watch Movie) 1) Initiation: Here the transcription is initiated by the binding of RNA polymerase to a specific region of DNA double helix. This site is called promoter site or promoter region. This region is recognized by sigma factor (subunit) of RNA polymerase. When RNA polymerase recognizes this region, it binds to it leading to a local unwinding (separation) of the promoter region into 2 single strands: 83 Where is transcription initiated? Promoters are sequences in the DNA just upstream of transcripts (coding sequences) that define the sites of initiation. Promoter5’ RNA 3’ The role of the promoter is to attract RNA polymerase to the correct start site so transcription can be initiated Promoter5’ RNA 3’ Regions of similarity are found around 10 and 35 bases before the start site of transcription: DNAse protection shows that RNA polymerase can bind to these same regions. Mutations of these sites can lead to the elimination or reduction of transcriptional initiation at a promoter. a- DNA strand that is transcripted into mRNA and called template strand or antisense strand. b- The other strand is coding strand or sense strand that contains gene to be translated ( This strand not transcripted, not used) Direction of transcription: RNA polymerase will read the information sequence on DNA template from 3′ → 5′ direction, so RNA is synthesized antiparallel to DNA template i.e. from 5′ → 3′ direction. 2) RNA elongation: Once RNA polymerase recognizes promoter region, it begins to synthesize a transcript (copy) of DNA template. 3) Termination: Process of elongation of RNA continues until reach what is called : termination region (C- rich region) which may be recognized by rho factor (additional protein) resulting in release of the enzyme, and the synthesized RNA 87 The elongation stage s70 dissociates from the core RNA polymerase after initiation occurs. This yields: In the absence of s70, RNA polymerase binds ssDNA tightly and is highly processive. Rho-dependent termination Some mRNAs synthesized by RNA polymerase in vitro fail to terminate at the normal in vivo position. – This suggested that additional proteins might be required for termination at these sites. – The missing factor was identified and named rho. Rho in action Rho is a hexamer helicase. Rho binds transcripts at stretches of ~100 nt free of 2nd structure and rich in cytosines. Can unwind RNA-DNA hybrids. Now the mRNA can take the genetic message out the cytosol, where it can be used to make proteins! 91 92 93 Post transcription process 94 RNA (Ribonucleic acid) Structure: Similar to that of DNA except: 1- it is single stranded polunucleotide chain. 2- Sugar is ribose 3- Uracil is instead of thymine There are 3 types of RNA: 1- Ribosomal RNA (rRNA) 2- Messenger RNA (mRNA) 3- Transfer RNA (tRNA) RNA are copies from DNA sequences formed by a process called “ transcription”. After transcription some modifications occur to obtain the three types of RNA. 95 Notes: 1- The synthesized RNA will have the sequence of the sense strand except for U instead of T. 2- In prokaryotic (bacteria) all types of RNA are synthesized by only one species of RNA polymerase. 3- In Eukaryotic ( mammaians), there are 3 classes of RNA polymerase a- RNA polymerase I: synthesizes the precursor of rRNA named : pre rRNA b- RNA polymerase II: synthesizes pre mRNA c- RNA polymerase III: synthesizes pre tRNA All these enzymes synthesize what is called primary transcript or immature RNAs (pre form) which by some modifications occur after transcription, will give the mature rRNA, mRNA and tRNA. 96 Examples on post-transcriptional modifications of RNA: 1-Transcritptinal modifications of mRNA: After transcription, the formed immature mRNA will undergo the following modifications to be mature and functioning: 1) 5′-capping: 5′- end in the first nucleotide is blocked by 7-methyl guanosine triphosphate (7 methyl-GTP). Role of cap: a- help to stabilize mRNA b- Permit initiation of translation (specifies, where translation should begin). 2) Poly A tail: chain of about 20-200 AMP is attached to 3′- end is added after transcription Role of poly A: Help to stabilize mRNA and facilitate its exit from the nucleus. 97 STABILITY Post-Transcriptional Modifications ©1998 by Alberts, Bray, Johnson, Lewis, Raff, Roberts, Walter. Published by Garland Publishing, a member of the Taylor & Francis Group. 2- In rRNA: pre rRNA is a long RNA that is cleaved into the normal sized rRNA species ( 5.8s, 18s and 28s). 5s species is synthesized separately. See hand out. 3- In tRNA: a- addition of CCA sequence to 3′ end of pre tRNA b- modification of bases to give unusual base 101 Structure of tRNA Funtions of tRNA 102 3. Translation - making proteins Nuclear DNA membrane Transcription Pre-mRNA Eukaryotic RNA Processing Cell mRNA Ribosome Translation Protein PROTEIN SYNTHESIS How your cell makes very important proteins The production (synthesis) of proteins. 3 phases: 1. Transcription 2. RNA processing 3. Translation DNA RNA Protein A. Messenger RNA (mRNA) start codon mRNA A U G G G C U C C A U C G G C G C A U A A codon 1 codon 2 codon 3 codon 4 codon 5 codon 6 codon 7 protein methionine glycine serine isoleucine glycine alanine stop codon Primary structure of a protein aa1 aa2 aa3 aa4 aa5 aa6 peptide bonds RNA and the Genetic Code Base triplets in an mRNA are words in a protein-building message Two other classes of RNA (rRNA and tRNA) translate those words into a polypeptide chain– all three are needed to complete process of making a protein Genetic Information From DNA to mRNA to amino acid sequence Genetic Code Genetic code – Consists of 64 codons (triplets) – Some amino acids are coded by more than one codon Some codons signal the start or end of a gene – AUG (methionine) is a start codon – UAA, UAG, and UGA are stop codons ACGATACCCTGACGAGCGTTAGCTATCG UGCUAUGGGACUG 14.4 Translation: RNA to Protein Translation converts genetic information carried by an mRNA into a new polypeptide chain The order of the codons in the mRNA determines the order of the amino acids in the polypeptide chain Ribosomes 2 subunits, separate in cytoplasm until they join to begin translation – Large – Small Contain 3 binding sites –E –P –A tRNA Function Amino acids must be in the correct order for the protein to function correctly tRNA lines up amino acids using mRNA code i.e: carry specific amino acid and recognize the codon for that amino acid so called (Adaptor molecules) tRNA Transfer RNA Bound to one amino acid on one end Anticodon on the other end complements mRNA codon III. Translation has 3 Steps, Each Requiring Different Supporting Proteins Initiation – Requires Initiation Factors Elongation – Requires Elongation Factors Termination – Requires Termination Factor Ribosomes Large subunit P A Site Site mRNA A U G C U A C U U C G Small subunit 3. Translation Three parts: 1. initiation: start codon (AUG) 2. elongation: 3. termination: stop codon (UAG) Let’s make a PROTEIN!!!!. Initiation: Assembly of components (large ribosmal subunit, small ribosomal subunit, mRNA and the tRNA carrying a methionine come together in the cytoplasm, the ribosome becomes active and "translation", is initiated). The AUG codon binds at the protein binding site (P) of the ribosome and AUG is always the first codon of an mRNA. Ribosomes Large subunit P A Site Site mRNA A U G C U A C U U C G Small subunit Initiation aa2 aa1 2-tRNA 1-tRNA G A U anticodon U A C A U G C U A C U U C G A hydrogen bonds codon mRNA The next complementary tRNA will bind at the attachment binding site (A) of the ribosome. The adjacent amino acids are then joined by a peptide bond via a peptidase enzyme. Thus the polypeptide chain begins to grow Elongation peptide bond aa3 aa1 aa2 3-tRNA G A A 1-tRNA 2-tRNA anticodon U A C G A U A U G C U A C U U C G A hydrogen bonds codon mRNA Elongation The ribosome then moves 1 codon down the mRNA in a 5' to 3' direction. This is achieved by a translocase enzyme. As the process of ribosome translocation continues, the "old" tRNA is released to bind another amino acid and go in search of a new codon. The binding of a new aminoacid is mediated by an enzyme called amino-acyl-tRNA synthase aa1 peptide bond aa3 aa2 1-tRNA U A C 3-tRNA (leaves) G A A 2-tRNA G A U A U G C U A C U U C G A mRNA Ribosomes move over one codon peptide bonds aa1 aa4 aa2 aa3 4-tRNA G C U 2-tRNA 3-tRNA G A U G A A A U G C U A C U U C G A A C U mRNA peptide bonds aa1 aa4 aa2 aa3 2-tRNA 4-tRNA G A U G C U (leaves) 3-tRNA G A A A U G C U A C U U C G A A C U mRNA Ribosomes move over one codon peptide bonds aa5 aa1 aa2 aa4 aa3 5-tRNA U G A 3-tRNA 4-tRNA G A A G C U G C U A C U U C G A A C U mRNA peptide bonds aa5 aa1 aa2 aa3 aa4 5-tRNA U G A 3-tRNA G A A 4-tRNA G C U G C U A C U U C G A A C U mRNA Ribosomes move over one codon Termination The process continues along the mRNA until a stop codon is reached. While there is no tRNA for a stop codon, there is an enzyme called release factor which cleaves the polypeptide chain resulting in a new protein. aa5 aa4 aa199 Termination aa3 primary aa200 structure of a protein aa2 aa1 terminator 200-tRNA or stop codon A C U C A U G U U U A G mRNA End Product The end products of protein synthesis is a primary structure of a protein. A sequence of amino acid bonded together by peptide bonds. aa5 aa4 aa3 aa2 aa199 aa1 aa200 Question The anticodon UAC belongs to a tRNA that recognizes and binds to a particular amino acid. What would be the DNA base code for this amino acid? Answer tRNA - UAC (anticodon) mRNA - AUG (codon) DNA - TAC Termination 1. Binding of Release Factor to Stop Codon UGA, UAA, UAG. 2. Disassembly Mutations What Are Mutations? Changes in the nucleotide sequence of DNA May occur in somatic cells (aren’t passed to offspring) May occur in gametes (eggs & sperm) and be passed to offspring Are Mutations Helpful or Harmful? Some type of skin cancers and leukemia result from somatic mutations Some mutations may improve an organism’s survival (beneficial) Types of Mutations Gene Mutations Change in the nucleotide sequence of a gene May only involve a single nucleotide May be due to long stretches of DNA Types of Gene Mutations Include: ◦ Point Mutations ◦ Gross mutation`` Point mutation Point Mutation (Single base substitutions) Includes the deletion, insertion, or substitution of ONE nucleotide in a gene (If one purine [A or G] or pyrimidine [C or T] is replaced by the other, the substitution is called a transition. If a purine is replaced by a pyrimidine or vice-versa, the substitution is called a transversion.) Classification: I) Point mutation (Base substitution): a- Transition pyrimidine pyrimidine purine purine b- Transversion pyrimidine Purine Sickle Cell Anemia Sickle-cell anemia is caused by a point mutation in the β-globin chain of haemoglobin, causing the hydrophilic amino acid glutamic acid to be replaced with the hydrophobic amino acid valine at the sixth position. Point mutation types 1- Missense mutations With a missense mutation, the new nucleotide alters the codon so as to produce an altered amino acid in the protein product. EXAMPLE: sickle-cell disease. The replacement of A by T at the 17th nucleotide of the gene for the beta chain of hemoglobin changes the codon GAG (for glutamic acid) to GTG (which encodes valine). Thus the 6th amino acid in the chain becomes valine instead of glutamic acid. Point Mutation Sickle Cell disease is the result of one nucleotide substitution Occurs in the hemoglobin gene 2-Nonsense mutations With a nonsense mutation, the new nucleotide changes a codon that specified an amino acid to one of the STOP codons Therefore, translation of the messenger RNA transcribed from this mutant gene will stop prematurely. the protein product will be unable to function. 3-Silent mutations Most amino acids are encoded by several different codons. For example, if the third base in the TCT codon for serine is changed to any one of the other three bases, serine will still be encoded. Such mutations are said to be silent because they cause no change in their product and cannot be detected without sequencing the gene (or its mRNA). Frameshift Mutation Inserting or deleting one or more nucleotides Changes the “reading frame” like changing a sentence Proteins built incorrectly Insertions and Deletions (Indels) Extra base pairs may be added (insertions) or removed (deletions) from the DNA of a gene. The number can range from one to thousands. Collectively, these mutations are called indels. Amino Acid Sequence Changed Cause alteration to DNA involving long stretches of sequences 1- deletion 2- insertion 3-rearangement 4- repetition