Molecular Biology Lecture 7 PDF
Document Details
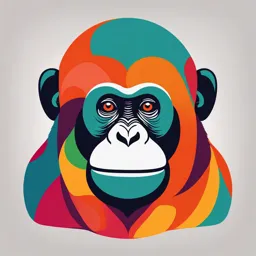
Uploaded by StateOfTheArtViolet
Francis Marion University
Tags
Summary
This document outlines various molecular biology concepts regarding cell signaling. It covers features, definitions, and changes in associated principles, such as binding sites, ligands, receptor activation, and their consequences. Other concepts included are related to molecular interactions in signaling, pathways, and their effects on cell growth.
Full Transcript
1.List and explain the following features of signaling/general principles involved in all signaling pathways and assess the consequences for changes in the associated steps and principles: binding sites, ligands, receptor activation, conformational flexibility, cooperativity, regulation, reversibili...
1.List and explain the following features of signaling/general principles involved in all signaling pathways and assess the consequences for changes in the associated steps and principles: binding sites, ligands, receptor activation, conformational flexibility, cooperativity, regulation, reversibility, propagation/amplification, and termination and reset 1. Binding Sites Definition: Binding sites are regions on receptors where ligands (e.g., hormones, neurotransmitters) bind. These sites are specific in shape and charge, allowing selective ligand interaction. Consequences of Changes: Altered binding site structure (mutations, chemical modifications) can reduce ligand affinity, preventing signal initiation or leading to non-specific binding, which can cause aberrant signaling. 2. Ligands Definition: Ligands are molecules that bind to receptors to trigger a response. They can be hormones, growth factors, neurotransmitters, or ions. Consequences of Changes: Changes in ligand concentration or structure can affect receptor activation. Overproduction may lead to overstimulation, while ligand deficiency results in reduced signaling. 3. Receptor Activation Definition: Upon ligand binding, receptors undergo a conformational change, triggering downstream signaling cascades. Consequences of Changes: Defective activation prevents the initiation of signaling pathways, potentially leading to diseases like diabetes or cancer (e.g., if insulin receptors or growth factor receptors fail to activate). 4. Conformational Flexibility Definition: Receptors exhibit conformational flexibility, changing shape to accommodate ligands and transmit signals. Consequences of Changes: Reduced flexibility can hinder receptor function. Excessive flexibility may lead to inappropriate activation even in the absence of ligands, leading to unregulated signaling. 5. Cooperativity Definition: Cooperativity occurs when the binding of one ligand affects the binding of additional ligands, often seen in multimeric receptors (e.g., hemoglobin). Consequences of Changes: Loss of cooperativity can reduce the efficiency of receptor activation, requiring higher ligand concentrations to achieve the same effect. Enhanced cooperativity can result in hyper-sensitivity to signaling molecules. 6. Regulation Definition: Cells regulate signaling through feedback mechanisms, involving inhibitors or activators that fine-tune the signal. Consequences of Changes: Dysregulation can lead to continuous signal transduction, contributing to pathologies such as cancer (e.g., dysregulated growth factor signaling). Insufficient regulation may lead to an inability to respond properly to external stimuli. 7. Reversibility Definition: Signaling pathways are reversible, meaning the binding of ligands and receptor activation can be undone, allowing pathways to reset. Consequences of Changes: If signaling is irreversible, continuous activation or inhibition can result, leading to disrupted cellular processes (e.g., constant receptor activation leading to cell overgrowth or cell death). 8. Propagation/Amplification Definition: Signal propagation involves transmitting a signal from the receptor to downstream molecules. Amplification allows a small initial signal to generate a large response (e.g., G-protein activation). Consequences of Changes: Impaired propagation reduces the efficiency of signal transmission. Excessive amplification may cause exaggerated responses, resulting in conditions like hyperactivity of immune responses. 9. Termination and Reset Definition: After signal transduction, pathways must terminate, and the system must reset to respond to future signals. This involves ligand dissociation, deactivation of second messengers, or receptor endocytosis. Consequences of Changes: Failure in termination can result in prolonged signaling, as seen in diseases like chronic inflammation or cancer. Inability to reset may impair future signaling responses, leading to cellular dysfunction. 2.Define the following: ligand, mitogen/mitogenic, dimerization, autophosphorylation, SRC-homology domain, growth factor, quorum sensing, growth, development, totipotency, pluripotency, stem cell, morphogenesis, specialization, and differentiation Molecular interaction principles: - Often REVERSIBLE - Ligand - Binding site - Conformational flexibility - inducible/induced fit - Cooperativity - Regulation Signaling pathway basics: Initiation, Propagation, Termination and Reset - Rate and progression controlled by enzymes - Post-translational modifications of proteins are critical to the function and activity of signaling pathways 1. Ligand A ligand is a molecule that binds specifically to a receptor on the cell surface or within a cell, initiating a signaling cascade. Ligands can be proteins, peptides, hormones, or ions. Typically highly specific, subject to concentration effects, quantifiable, reversible 2. Mitogen/Mitogenic A mitogen is a substance that stimulates cell division (mitosis) by triggering specific signaling pathways. Mitogenic refers to the ability of a molecule to induce mitosis or promote cell proliferation. - Examples: Growth factors (EGF), Tyrosine Kinases (EGFR), SH2-domain containing proteins (PI3K, GTP binding proteins (Ras), Serine/Threonine kinases (Raf), transcription factors 3. Dimerization Dimerization refers to the process where two receptor molecules (often identical or similar) come together to form a functional pair (dimer) upon ligand binding, which activates downstream signaling. 4. Autophosphorylation Autophosphorylation occurs when a receptor or enzyme adds a phosphate group to itself, usually at tyrosine, serine, or threonine residues. This self-phosphorylation is a key step in receptor activation and intracellular signaling. 5. SRC-Homology Domain (SH Domain) SRC-homology domains (SH2 and SH3) are protein domains found in various signaling proteins. SH2 domains bind to phosphorylated tyrosines on receptors or adaptor proteins, while SH3 domains mediate protein-protein interactions, facilitating signal transduction. - Protein example: P13K 6. Growth Factor A growth factor is a signaling molecule that promotes cell growth, differentiation, and survival. Examples include epidermal growth factor (EGF) and nerve growth factor (NGF), which bind to specific receptors to trigger cellular responses. 7. Quorum Sensing Quorum sensing is a form of cell-to-cell communication in bacteria, where the production and detection of signaling molecules (autoinducers) allow bacteria to coordinate behavior based on population density, such as biofilm formation or virulence factor expression. 8. Growth In cell biology, growth refers to the increase in cell size or the number of cells, typically driven by signaling pathways that regulate the cell cycle, protein synthesis, and nutrient uptake. 9. Development Development refers to the process by which cells, tissues, and organisms grow and specialize over time, involving stages such as cell division, differentiation, and morphogenesis. 10. Totipotency Totipotency is the ability of a single cell (e.g., a zygote) to develop into all the cell types of an organism, including both embryonic and extra-embryonic tissues (e.g., placenta). 11. Pluripotency Pluripotency is the ability of a stem cell to differentiate into any of the three germ layers (ectoderm, mesoderm, and endoderm), which can give rise to all the body’s cell types, but not extra-embryonic tissues. 12. Stem Cell A stem cell is an undifferentiated cell with the ability to self-renew and differentiate into various specialized cell types. Stem cells can be totipotent, pluripotent, or multipotent based on their differentiation potential. 13. Morphogenesis Morphogenesis is the biological process that causes an organism or tissue to develop its shape. It is driven by cellular processes such as cell migration, differentiation, and apoptosis during development. 14. Specialization Specialization is the process by which cells acquire distinct functional characteristics, often resulting from differentiation, allowing them to perform specific roles within a tissue or organ. 15. Differentiation Differentiation is the process by which a less specialized cell undergoes changes to become more specialized in form and function, typically as part of development or in response to signaling cues. 3.Evaluate cellular signaling and its function in cell proliferation and embryogenesis ◦Identify whether a molecule is defined as mitogenic and explain the role of mitogenic signaling in cell growth and regulation of the cell cycle ◦Summarize the generic MAPK signaling cascade Growth must be triggered - Polypeptide hormones - Cytokines - Growth Factors Growth relies on signaling cascades that alter gene activity and promote cellular division General Consequences of Growth Factor Signaling - Increase intracellular Ca2+ levels - Reorganization of actin stress fibers - Activation and/or translocation to nucleus of transcription factors (turn on immediate early genes) - DNA synthesis and cell division 1. Cellular Signaling in Cell Proliferation and Embryogenesis Cellular signaling plays a critical role in both cell proliferation and embryogenesis by controlling the behavior of cells through various pathways. In cell proliferation, signaling pathways regulate the cell cycle, ensuring cells grow, divide, and repair tissues. In embryogenesis, signaling governs early development, driving processes such as differentiation, tissue formation, and morphogenesis to build the organism. 2. Mitogenic Molecules and Their Role in Cell Growth A mitogenic molecule is defined as one that promotes cell division (mitosis) by activating pathways that lead to cell cycle progression. Examples of mitogenic molecules include growth factors such as epidermal growth factor (EGF) and platelet-derived growth factor (PDGF). These ligands bind to their receptors on the cell surface, triggering mitogenic signaling cascades. Mitogenic Signaling in Cell Growth and Cell Cycle Regulation Initiation: Mitogenic signals, often through receptor tyrosine kinases (RTKs), are activated when growth factors bind to their receptors. This leads to receptor dimerization and autophosphorylation, which triggers downstream signaling pathways. Role in Cell Growth: Mitogenic signaling promotes the expression of genes that are critical for cell cycle progression, particularly those involved in moving the cell from the G1 phase to the S phase, where DNA replication occurs. Regulation: These signals are tightly regulated to prevent uncontrolled cell division, which can lead to cancer. Negative feedback loops and checkpoints within the cell cycle ensure proper timing and response to mitogens. 3. The MAPK (Mitogen-Activated Protein Kinase) Signaling Cascade The MAPK signaling cascade is a key pathway that mediates responses to mitogenic signals, influencing cell proliferation, differentiation, and survival. Here's a summary of the generic MAPK cascade: Steps in the MAPK Cascade: 1. Receptor Activation: A ligand (e.g., a growth factor) binds to a receptor, typically a receptor tyrosine kinase (RTK). This leads to receptor dimerization and autophosphorylation. 2. Activation of Ras: The activated receptor recruits adaptor proteins (like Grb2) that bind to the small GTPase Ras. Ras is then activated by exchanging GDP for GTP, becoming Ras-GTP. 3. Activation of RAF: Activated Ras-GTP binds and activates the RAF kinase, which is the first kinase in the MAPK cascade. 4. Phosphorylation of MEK: RAF phosphorylates and activates MEK (Mitogen-activated protein kinase kinase). 5. Phosphorylation of ERK: MEK phosphorylates and activates ERK (Extracellular signal-regulated kinase), the final kinase in the cascade. 6. Transcriptional Activation: Activated ERK translocates to the nucleus and phosphorylates various transcription factors, leading to the expression of genes that promote cell proliferation, differentiation, or survival. Regulation of the MAPK Pathway: Positive feedback: Some downstream products may enhance pathway activation. Negative feedback: Inhibitors, such as MAP kinase phosphatases (MKPs), deactivate components of the cascade, ensuring the pathway is tightly controlled. Consequences of Dysregulated MAPK Signaling: Overactivation: Continuous or unregulated MAPK activation can lead to excessive cell proliferation and cancer (e.g., mutations in Ras or RAF in cancers like melanoma). Inhibition: Insufficient MAPK activity can impair cell proliferation, affecting tissue regeneration and repair. 4.Identify the corresponding signaling mediators for a given receptor or pathway and assess the consequence of altering that mediator on the overall activity of the pathway with an emphasis on the following pathways: MAPK, EGFR, Integrin, Fas/Apoptosis Signaling, NOTCH, and Wnt signaling ◦Outline the steps of tyrosine kinase receptor activation and explain the process and importance of both dimerization and autophosphorylation ◦Explain the role of SRC homology domains in mitogenic signaling ◦You do NOT need to memorize the entire signaling cascade but rather should be able to work through a signaling process and identify the consequences of alterations by following the pathway downstream EVEN IF GIVEN A PATHWAY YOU HAVE NEVER SEEN BEFORE ◦Link a given pathway to the expected consequence and determine the anticipated change if the signaling pathway is altered 1. Identify Signaling Mediators and Consequences of Alterations For each pathway, the key mediators are critical components that regulate the cascade. Alterations in these mediators can have profound effects on pathway activity, including hyperactivation or inhibition, both of which could lead to disease. MAPK Pathway Key Mediators: Ras, RAF, MEK, ERK Consequence of Altering Mediators: ○ If Ras is overactive (e.g., mutation leads to constant GTP binding), it leads to continuous ERK activation and uncontrolled cell proliferation (common in cancers). ○ If MEK is inhibited, the pathway cannot proceed to ERK, impairing cell proliferation and survival. EGFR (Epidermal Growth Factor Receptor) Key Mediators: EGFR, Grb2, Ras, PI3K, PLCγ, STAT proteins Consequence of Altering Mediators: ○ Overexpression of EGFR (as seen in some cancers) leads to excessive cell proliferation and survival signals. ○ Loss of function in Grb2 or STATs would block downstream signaling, leading to poor cell proliferation and reduced growth. Integrin Signaling Key Mediators: FAK (Focal Adhesion Kinase), Src, PI3K, Rac, Rho Consequence of Altering Mediators: ○ Loss of FAK would prevent integrin-mediated adhesion signals, impairing cell migration and motility. ○ Overactivation of Rho could lead to excessive stress fiber formation and hinder proper cell movement. Fas/Apoptosis Signaling Key Mediators: Fas receptor, FADD, caspases (initiator and executioner), BID Consequence of Altering Mediators: ○ Loss of caspase 8 prevents apoptosis, leading to survival of cells that should undergo programmed death (e.g., in immune or cancer cells). ○ Overactivation of Fas signaling leads to excessive apoptosis, contributing to degenerative conditions. NOTCH Signaling Key Mediators: NOTCH receptor, NICD (NOTCH intracellular domain), CSL complex Consequence of Altering Mediators: ○ Overactivation of NOTCH can lead to aberrant cell fate decisions, contributing to developmental defects or cancers. ○ Inhibition of NICD would prevent the expression of downstream genes essential for cell differentiation. Wnt Signaling Key Mediators: Wnt ligands, Frizzled receptor, β-catenin, GSK-3β, APC Consequence of Altering Mediators: ○ Loss of APC or GSK-3β allows unregulated β-catenin activity, leading to uncontrolled cell proliferation (e.g., colon cancer). ○ Deficient Wnt signaling can result in impaired tissue development and stem cell maintenance. 2. Steps of Tyrosine Kinase Receptor Activation Tyrosine kinase receptors (RTKs) like EGFR follow a general activation process: Ligand Binding: A signaling molecule (ligand) binds to the extracellular domain of the receptor. Dimerization: Ligand binding induces the receptor to dimerize (form a pair), which is crucial for activation. Autophosphorylation: Upon dimerization, the receptors phosphorylate each other on specific tyrosine residues in their intracellular domains. Downstream Signaling: The phosphorylated tyrosines serve as docking sites for signaling proteins, which propagate the signal inside the cell. Importance: ○ Dimerization: Ensures that receptor activation only occurs upon ligand binding, thus controlling signal specificity. ○ Autophosphorylation: Critical for recruiting signaling proteins to the activated receptor and initiating the cascade. Consequences of Alterations: ○ Mutations that prevent dimerization (e.g., EGFR) lead to loss of function, while mutations that cause dimerization without ligand binding can lead to constant activation (oncogenic). 3. Role of SRC Homology Domains (SH2 and SH3) in Mitogenic Signaling SRC-homology domains are important in linking receptor activation to downstream signaling. SH2 Domain: Binds to specific phosphorylated tyrosines on receptors or adaptor proteins. This enables signal transduction by bringing key signaling proteins to the active receptor. SH3 Domain: Mediates interactions with other proteins through proline-rich sequences, facilitating the assembly of signaling complexes. Role in Mitogenic Signaling: ○ In pathways like MAPK, the SH2 domain on proteins like Grb2 binds to phosphorylated RTKs, allowing Ras activation. SH3 domains help recruit other molecules that propagate the signal. Without functional SH2/SH3 domains, signaling would be inefficient, impairing mitogenic signals required for cell growth. 4. Assessing Consequences of Pathway Alterations To handle signaling processes, even if you're unfamiliar with a particular pathway, follow these general steps: Identify the Key Components: Look at the ligands, receptors, mediators, and transcription factors involved. Understand the Direction of Signaling: Whether the signal leads to growth, apoptosis, differentiation, etc. Assess the Role of Mediators: What happens if a mediator is missing, overactive, or inactive? This could lead to a blockage in the pathway, over-activation, or faulty cell behavior. 5. Linking Pathways to Expected Consequences MAPK: Hyperactivation leads to cancer (e.g., Ras mutations), while inhibition could lead to insufficient cell growth. EGFR: Overactivation (e.g., in lung cancer) leads to excessive cell proliferation, while inhibition leads to poor growth responses. Fas/Apoptosis: Defective Fas signaling prevents apoptosis, potentially resulting in immune dysfunction or cancer. Wnt: Overactive β-catenin (due to Wnt pathway mutation) can lead to unchecked cell growth (colon cancer). 6.Explain the role of signaling in cellular communication, morphogenesis, motility/movement, tissue development, and tissue organization Pluripotency = Maintain state capable of generation of all 3 embryonic germ layers - Ectoderm, mesoderm, and endoderm Categorization of a wide set of subtypes - Naïve vs. primed Differentiation triggers significant changes - i.e. Global CDK activity decreases with specialization of a cell Transcription Factors - SOX2, NANOG, OCT4, MYC - Can be used to induce a pluripotent state (reprogramming) 1. Signaling induces differentiation and development of specific cell populations 2. Signaling and cell-to-cell communication ensures coupled growth of tissues 3. Signaling also controls the overall behaviors to ensure movement and function is as a tissue rather than independent cells Extracellular Matrix Signaling - Communication occurs between cells and the ECM - Multiple means of signaling between ECM and cells exists (receptors, proteolysis, transduction) - Critical component of both morphogenesis and tissue repair 7.Assess the consequences for changes in signaling pathways or signaling components on cell viability and growth (Assess the consequences for these changes in terms of generating or mitigating human disease in both a general sense and using specific examples) ◦Link specific genetic changes or molecules with the expected phenotype or disease manifestation (and vice versa) ◦Compare and contrast the consequences for changes in signaling during embryogenesis/development and within an adult 3 main options: Increase, Decrease, Stay the Same Signals that promote growth - Consequence for Upregulation = increased growth - Consequence for Downregulation = decreased or absent growth Signals that control death - Consequence for Upregulation = VARIABLE - If suppresses death = less death signaling - If activates or promotes death = more death signaling - - Consequence for Downregulation = VARIABLE - If suppresses death = more death signaling - If activates or promotes death = less death signaling Assessing the Consequences of Changes in Signaling Pathways on Cell Viability and Growth Alterations in signaling pathways can profoundly impact both cell viability (the ability of a cell to survive) and cell growth (including proliferation, differentiation, and apoptosis). The consequences of these changes can either contribute to human diseases or, when corrected, mitigate them. Below is a general assessment and specific examples illustrating the consequences of these alterations. 1. General Consequences of Signaling Pathway Alterations on Disease Hyperactivation of Pathways: Signaling pathways that are overstimulated can lead to uncontrolled cell growth, which is characteristic of cancer. For instance, hyperactivation of growth-promoting pathways like MAPK or PI3K-Akt can drive unregulated proliferation, survival, and inhibition of apoptosis. Inhibition of Pathways: Disruption of normal signaling (e.g., loss of function) can lead to cell death or reduced growth. This may result in degenerative diseases, developmental disorders, or impaired tissue repair. 2. Specific Examples of Genetic Changes or Altered Signaling Molecules and Their Disease Manifestation a. Ras Mutation (MAPK Pathway) Genetic Change: Mutations in the Ras gene often lead to the constitutive activation of the Ras protein (it is permanently in the GTP-bound state). Disease Manifestation: Constitutively active Ras leads to continuous activation of the downstream MAPK pathway, promoting excessive cell division and survival. Phenotype/Disease: Cancers such as pancreatic, lung, and colorectal cancers often involve Ras mutations. Mitigation: Targeting components of the MAPK pathway with inhibitors (e.g., RAF inhibitors) can mitigate cancer progression. b. BRCA1/BRCA2 Mutations (DNA Damage Signaling) Genetic Change: Loss of function mutations in BRCA1/BRCA2, genes involved in DNA repair signaling. Disease Manifestation: Deficient DNA repair leads to the accumulation of genetic damage and genomic instability. Phenotype/Disease: Breast and ovarian cancer are linked to mutations in BRCA1/BRCA2, where cells become prone to accumulating mutations that promote cancer. Mitigation: PARP inhibitors are used to target cancer cells with BRCA mutations, enhancing DNA damage in cancerous cells and inducing cell death. c. APC Mutation (Wnt Pathway) Genetic Change: Mutations in the APC gene result in the failure to degrade β-catenin, leading to the persistent activation of Wnt signaling. Disease Manifestation: Uncontrolled β-catenin promotes excessive cell proliferation, especially in the intestinal epithelium. Phenotype/Disease: Mutations in APC are a hallmark of familial adenomatous polyposis (FAP), which increases the risk of colorectal cancer. Mitigation: Therapeutic interventions target downstream elements of the Wnt pathway or reduce the tumor burden via surgery or chemoprevention. d. EGFR Mutation (EGFR Pathway) Genetic Change: Mutations in EGFR can lead to its constitutive activation without ligand binding. Disease Manifestation: This results in continuous cell growth and survival signals. Phenotype/Disease: Non-small cell lung cancer (NSCLC) is often associated with EGFR mutations. Mitigation: Targeting EGFR with tyrosine kinase inhibitors (TKIs), such as erlotinib or gefitinib, helps to control cancer progression. e. NOTCH Mutation (Notch Signaling) Genetic Change: Gain-of-function mutations in the NOTCH receptor cause persistent activation of the pathway. Disease Manifestation: Overactive Notch signaling can drive abnormal cell fate decisions. Phenotype/Disease: NOTCH pathway mutations are linked to T-cell acute lymphoblastic leukemia (T-ALL). Mitigation: NOTCH inhibitors are being developed to mitigate uncontrolled cell growth in leukemia. f. Loss of Fas or Caspases (Fas/Apoptosis Pathway) Genetic Change: Loss of function mutations in Fas or downstream caspases involved in apoptosis. Disease Manifestation: Inability to undergo programmed cell death leads to the survival of damaged or unwanted cells. Phenotype/Disease: Defective apoptosis is linked to conditions like autoimmune lymphoproliferative syndrome (ALPS), where defective apoptosis leads to immune cell accumulation and autoimmunity. Mitigation: Targeting the Fas pathway in overactive immune conditions may restore the balance of immune cell apoptosis. 3. Comparing and Contrasting Signaling Changes in Embryogenesis/Development and Adults The consequences of signaling changes vary depending on whether they occur during embryogenesis (development) or in adult cells. a. Embryogenesis/Development Key Processes: Signaling pathways regulate cell fate decisions, differentiation, and tissue morphogenesis. Consequences of Alterations: ○ Loss of NOTCH signaling: During development, impaired NOTCH can lead to defective cell differentiation, causing congenital abnormalities. ○ Overactive Wnt signaling: Can lead to developmental malformations or improper tissue specification. ○ Importance: Any disruption in embryonic signaling often leads to profound developmental defects or embryonic lethality due to the reliance on precise, tightly controlled signaling for correct tissue patterning and organogenesis. b. Adult Cells Key Processes: Signaling pathways control tissue maintenance, repair, cell proliferation, and programmed cell death. Consequences of Alterations: ○ Loss of EGFR signaling: In adults, loss of EGFR signaling can impair wound healing and tissue regeneration. ○ Overactive Ras/MAPK signaling: Leads to uncontrolled cell growth, contributing to cancer. ○ Importance: In adult tissues, aberrant signaling often manifests as degenerative diseases or cancer due to disruptions in cellular homeostasis and repair mechanisms. Summary Changes in signaling pathways have profound effects on both cell viability and growth. Hyperactivation of pathways can lead to cancer, while inhibition can cause cell death or tissue degeneration. Embryogenesis is highly sensitive to alterations, with developmental defects often being irreversible or fatal, while in adult cells, altered signaling commonly results in diseases like cancer or degenerative conditions. By linking specific genetic mutations (e.g., Ras, APC, BRCA1) to diseases like cancer, we understand how dysregulated signaling contributes to pathology and explore therapeutic interventions to target these alterations.