Module 6 - Chemistry in the Environment PDF
Document Details
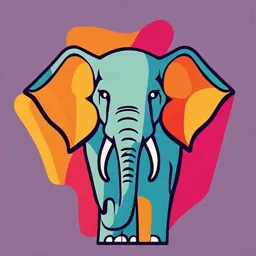
Uploaded by EthicalFractal
Tags
Summary
This document is a chapter on chemistry in the environment, specifically focusing on the atmosphere. It discusses the structure and composition of the atmosphere, chemical processes occurring in the atmosphere, sources of air pollution, and potential control measures.
Full Transcript
20.1 Earth’s Atmosphere 901 W e have studied basic definitions in chemistry, and we have examined the properties of gases, liquids, solids, and solutions. We have discussed chemical bonding and intermolecular forces and seen how chemical kinetics and chemical equilibrium c...
20.1 Earth’s Atmosphere 901 W e have studied basic definitions in chemistry, and we have examined the properties of gases, liquids, solids, and solutions. We have discussed chemical bonding and intermolecular forces and seen how chemical kinetics and chemical equilibrium concepts help us understand the nature of chemical reactions. It is appropriate at this stage to apply our knowledge to the study of one extremely important system: the atmosphere. Although Earth’s atmosphere is fairly simple in composition, its chemistry is very complex and not fully understood. The chemical processes that take place in our atmosphere are induced by solar radiation, but they are intimately connected to natural events and human activities on Earth’s surface. In this chapter, we will discuss the structure and composition of the atmosphere, together with some of the chemical processes that occur there. In addition, we will take a look at the major sources of air pollution and prospects for controlling them. 20.1 Earth’s Atmosphere Earth is unique among the planets of our solar system in having an atmosphere that is chemically active and rich in oxygen. Mars, for example, has a much thinner atmosphere that is about 90 percent carbon dioxide. Jupiter, on the other hand, has no solid surface; it is made up of 90 percent hydrogen, 9 percent helium, and 1 percent other substances. It is generally believed that 3 to 4 billion years ago, Earth’s atmosphere con- sisted mainly of ammonia, methane, and water. There was little, if any, free oxygen present. Ultraviolet (UV) radiation from the sun probably penetrated the atmosphere, rendering the surface of Earth sterile. However, the same UV radiation may have triggered the chemical reactions (perhaps beneath the surface) that eventually led to life on Earth. Primitive organisms used energy from the sun to break down carbon dioxide (produced by volcanic activity) to obtain carbon, which they incorporated in their own cells. The major by-product of this process, called photosynthesis, is oxygen. Another important source of oxygen is the photodecomposition of water vapor by UV light. Over time, the more reactive gases such as ammonia and meth- ane have largely disappeared, and today our atmosphere consists mainly of oxygen and nitrogen gases. Biological processes determine to a great extent the atmospheric concentrations of these gases, one of which is reactive (oxygen) and the other unre- active (nitrogen). Table 20.1 shows the composition of dry air at sea level. The total mass of the atmosphere is about 5.3 3 1018 kg. Water is excluded from this table because its concentration in air can vary drastically from location to location. Figure 20.1 shows the major processes involved in the cycle of nitrogen in nature. Molecular nitrogen, with its triple bond, is a very stable molecule. How- Table 20.1 ever, through biological and industrial nitrogen fixation, the conversion of molec- Composition of Dry Air ular nitrogen into nitrogen compounds, atmospheric nitrogen gas is converted at Sea Level into nitrates and other compounds suitable for assimilation by algae and plants. Another important mechanism for producing nitrates from nitrogen gas is light- Composition ning. The steps are Gas (% by Volume) N2 78.09 electrical N2 (g) 1 O2 (g) ¬¬¡ energy 2NO(g) O2 20.95 2NO(g) 1 O2 (g) ¬¬¡ 2NO2 (g) Ar 0.93 2NO2 (g) 1 H2O(l) ¬¬¡ HNO2 (aq) 1 HNO3 (aq) CO2 0.040 Ne 0.0018 About 30 million tons of HNO3 are produced this way annually. Nitric acid is He 0.000524 converted to nitrate salts in the soil. These nutrients are taken up by plants, which Kr 0.00011 in turn are ingested by animals. Animals use the nutrients from plants to make Xe 0.000006 proteins and other essential biomolecules. Denitrification reverses nitrogen fixation 902 Chapter 20 Chemistry in the Atmosphere Atmospheric nitrogen Atmospheric fixation Fixed juvenile nitrogen Industrial fixation Biological fixation Protein Denitrification Igneous rocks Plant and animal wastes, dead organisms Nitrate reduction Nitrous oxide Ammonium Nitrite Nitrate To groundwater Figure 20.1 The nitrogen cycle. Although the supply of nitrogen in the atmosphere is virtually inexhaustible, it must be combined with hydrogen or oxygen before it can be assimilated by higher plants, which in turn are consumed by animals. Juvenile nitrogen is nitrogen that has not previously participated in the nitrogen cycle. to complete the cycle. For example, certain anaerobic organisms decompose ani- mal wastes as well as dead plants and animals to produce free molecular nitrogen from nitrates. The main processes of the global oxygen cycle are shown in Figure 20.2. This cycle is complicated by the fact that oxygen takes so many different chemical forms. Atmospheric oxygen is removed through respiration and various industrial processes (mostly combustion), which produce carbon dioxide. Photosynthesis is the major mechanism by which molecular oxygen is regenerated from carbon diox- ide and water. Scientists divide the atmosphere into several different layers according to tem- perature variation and composition (Figure 20.3). As far as visible events are concerned, the most active region is the troposphere, the layer of the atmosphere that contains about 80 percent of the total mass of air and practically all of the atmosphere’s water vapor. The troposphere is the thinnest layer of the atmo- sphere (10 km), but it is where all the dramatic events of weather—rain, lightning, hurricanes—occur. Temperature decreases almost linearly with increasing altitude in this region. 20.1 Earth’s Atmosphere 903 O High-energy ultraviolet radiation H H2O H2O O2 O2 O2 ⫹ 2CO 2CO2 OH O3 Ozone screen O O2 CO O2 O2 Volcanism CO2 CO2 CO2 Oxidative weathering Phytoplankton 4FeO ⫹ O2 2Fe2O3 Photic zone H2O ⫹ CO2 H2CO3 HCO3ⴚ ⫹ H⫹ 2HCOⴚ 3 CO2ⴚ 3 CaCO3 Ca2⫹ Sediments Sediments H2O Figure 20.2 The oxygen cycle. The cycle is complicated because oxygen appears in so many chemical forms and combinations, primarily as molecular oxygen, in water, and in organic and inorganic compounds. Above the troposphere is the stratosphere, which consists of nitrogen, oxygen, and ozone. In the stratosphere, the air temperature rises with altitude. This warming effect is the result of exothermic reactions triggered by UV radiation from the sun (to be discussed in Section 20.3). One of the products of this reaction sequence is ozone (O3), which, as we will see shortly, serves to prevent harmful UV rays from reaching Earth’s surface. In the mesosphere, which is above the stratosphere, the concentration of ozone and other gases is low, and the temperature decreases with increasing altitude. The thermosphere, or ionosphere, is the uppermost layer of the atmosphere. The rise in temperature in this region is the result of the bombardment of molecular oxygen and nitrogen and atomic species by energetic particles, such as electrons and protons, from the sun. Typical reactions are N2 ¡ 2N ¢H° 5 941.4 kJ/mol N ¡ N1 1 e2 ¢H° 5 1400 kJ/mol O2 ¡ O 1 2 1 e 2 ¢H° 5 1176 kJ/mol In reverse, these processes liberate the equivalent amount of energy, mostly as heat. Ionized particles are responsible for the reflection of radio waves back toward Earth. 904 Chapter 20 Chemistry in the Atmosphere 500 km 1000˚C 400 Space station 300 950˚C Thermosphere 200 900˚C Aurora 700˚C borealis 100 165˚C Shooting star –80˚C 80 Mesosphere 50 0˚C Stratosphere –50˚C Ozone layer Concorde Troposphere 10 1˚C Mt. Pinatubo 0 Figure 20.3 Regions of Earth’s atmosphere. Notice the variation of temperature with altitude. Most of the phenomena shown here are discussed in the chapter. 20.2 Phenomena in the Outer Layers of the Atmosphere 905 20.2 Phenomena in the Outer Layers of the Atmosphere In this section, we will discuss two dazzling phenomena that occur in the outer regions of the atmosphere. One is a natural event. The other is a curious by-product of human space travel. Aurora Borealis and Aurora Australis Violent eruptions on the surface of the sun, called solar flares, result in the ejection of myriad electrons and protons into space, where they disrupt radio transmission and provide us with spectacular celestial light shows known as auroras (Figure 20.4). These electrons and protons collide with the molecules and atoms in Earth’s upper atmosphere, causing them to become ionized and electronically excited. Eventually, the excited molecules and ions return to the ground state with the emission of light. For example, an excited oxygen atom emits photons at wavelengths of 558 nm (green) and between 630 nm and 636 nm (red): O* ¡ O 1 hv where the asterisk denotes an electronically excited species and hv the emitted photon (see Section 7.2). Similarly, the blue and violet colors often observed in auroras result from the transition in the ionized nitrogen molecule: N1 1 2 * ¡ N2 1 hv The wavelengths for this transition fall between 391 and 470 nm. The incoming streams of solar protons and electrons are oriented by Earth’s magnetic field so that most auroral displays occur in doughnut-shaped zones about 2000 km in diameter centered on the North and South Poles. Aurora bore- alis is the name given to this phenomenon in the Northern Hemisphere. In the Southern Hemisphere, it is called aurora australis. Sometimes, the number of solar particles is so immense that auroras are also visible from other locations on Earth. Figure 20.4 Aurora borealis, commonly referred to as the northern lights. 906 Chapter 20 Chemistry in the Atmosphere Example 20.1 The bond enthalpy of O2 is 498.7 kJ/mol. Calculate the maximum wavelength (nm) of a photon that can cause the dissociation of an O2 molecule. Strategy We want to calculate the wavelength of a photon that will break an O“O bond. Therefore, we need the amount of energy in one bond. The bond enthalpy of O2 is given in units of kJ/mol. The units needed for the energy of one bond are J/molecule. Once we know the energy in one bond, we can calculate the minimum frequency and maximum wavelength needed to dissociate one O2 molecule. The conversion steps are kJ/mol ¡ J/molecule ¡ frequency of photon ¡ wavelength of photon Solution First we calculate the energy required to break one O“O bond: 498.7 3 103 J 1 mol J 3 23 5 8.281 3 10219 1 mol 6.022 3 10 molecules molecule The energy of the photon is given by E 5 hv [Equation (7.2)]. Therefore, E 8.281 3 10219 J v5 5 h 6.63 3 10234 J ? s 5 1.25 3 1015 s21 Finally, we calculate the wavelength of the photon, given by l 5 c/v [see Equation (7.1)], as follows: 3.00 3 108 mys λ5 1.25 3 1015 s21 5 2.40 3 1027 m 5 240 nm Comment In principle, any photon with a wavelength of 240 nm or shorter can Similar problem: 20.11. dissociate an O2 molecule. Practice Exercise Calculate the wavelength (in nm) of a photon needed to dissociate an O3 molecule: O3 ¡ O 1 O2 ¢H° 5 107.2 kJ/mol The Mystery Glow of Space Shuttles A human-made light show that baffled scientists for several years is produced by space shuttles orbiting Earth. In 1983, astronauts first noticed an eerie orange glow on the outside surface of their spacecraft at an altitude about 300 km above Earth (Figure 20.5). The light, which usually extends about 10 cm away from the protec- tive silica heat tiles and other surface materials, is most pronounced on the parts of the shuttle facing its direction of travel. This fact led scientists to postulate that collision between oxygen atoms in the atmosphere and the fast-moving shuttle somehow produced the orange light. Spectroscopic measurements of the glow, as well as laboratory tests, strongly suggested that nitric oxide (NO) and nitrogen Figure 20.5 The glowing tail dioxide (NO2) also played a part. It is believed that oxygen atoms interact with section of the space shuttle viewed from inside the vehicle. nitric oxide adsorbed on (that is, bound to) the shuttle’s surface to form electroni- cally excited nitrogen dioxide: O 1 NO ¡ NO2* As the NO2* leaves the shell of the spacecraft, it emits photons at a wavelength of 680 nm (orange). NO2* ¡ NO2 1 hv 20.3 Depletion of Ozone in the Stratosphere 907 Support for this explanation came inadvertently in 1991, when astronauts aboard Discovery released various gases, including carbon dioxide, neon, xenon, and nitric oxide, from the cargo bay in the course of an unrelated experiment. Expelled one at a time, these gases scattered onto the surface of the shuttle’s tail. The nitric oxide caused the normal shuttle glow to intensify markedly, but the other gases had no effect on it. What is the source of the nitric oxide on the outside of the spacecraft? Scientists believe that some of it may come from the exhaust gases emitted by the shuttle’s rockets and that some of it is present in the surrounding atmosphere. The shuttle glow does not harm the vehicle, but it does interfere with spectroscopic measurements on distant objects made from the spacecraft. 20.3 Depletion of Ozone in the Stratosphere As mentioned earlier, ozone in the stratosphere prevents UV radiation emitted by the sun from reaching Earth’s surface. The formation of ozone in this region begins with the photodissociation of oxygen molecules by solar radiation at wavelengths below 240 nm: Photodissociation is the breaking of chemical bonds by radiant energy. UV O2 ¬¬¡ , 240 nm O1O (20.1) The highly reactive O atoms combine with oxygen molecules to form ozone as follows: O 1 O 2 1 M ¡ O3 1 M (20.2) where M is some inert substance such as N2. The role of M in this exothermic reaction is to absorb some of the excess energy released and prevent the spontaneous decom- position of the O3 molecule. The energy that is not absorbed by M is given off as heat. (As the M molecules themselves become de-excited, they release more heat to the surroundings.) In addition, ozone itself absorbs UV light between 200 and 300 nm: UV O3 ¡ O 1 O 2 (20.3) The process continues when O and O2 recombine to form O3 as shown in Equation (20.2), further warming the stratosphere. If all the stratospheric ozone were compressed into a single layer at STP on Earth, that layer would be only about 3 mm thick! Although the concentration of ozone in the stratosphere is very low, it is sufficient to filter out (that is, absorb) solar radiation Recycling feasible in the 200- to 300-nm range [see Equation (20.3)]. In the stratosphere, it acts as our Recycling not feasible protective shield against UV radiation, which can induce skin cancer, cause genetic mutations, and destroy crops and other forms of vegetation. The formation and destruction of ozone by natural processes is a dynamic equi- Auto air librium that maintains a constant concentration of ozone in the stratosphere. Since the conditioning Foam 21% mid-1970s scientists have been concerned about the harmful effects of certain chlo- insulation 20% rofluorocarbons (CFCs) on the ozone layer. The CFCs, which are generally known Commercial by the trade name Freons, were first synthesized in the 1930s. Some of the common refrigeration Other foam 17% ones are CFCl3 (Freon 11), CF2Cl2 (Freon 12), C2F3Cl3 (Freon 113), and C2F4Cl2 uses 13% Solvent (Freon 114). Because these compounds are readily liquefied, relatively inert, nontoxic, cleaning noncombustible, and volatile, they have been used as coolants in refrigerators and air 4% 11% 14% conditioners, in place of highly toxic liquid sulfur dioxide (SO2) and ammonia (NH3). Aerosols Large quantities of CFCs are also used in the manufacture of disposable foam products such as cups and plates, as aerosol propellants in spray cans, and as solvents to clean Others (sterilization, household refrigeration) newly soldered electronic circuit boards (Figure 20.6). In 1977, the peak year of production, nearly 1.5 3 106 tons of CFCs were produced in the United States. Most Figure 20.6 Uses of CFCs. Since 1978, the use of aerosol of the CFCs produced for commercial and industrial use are eventually discharged propellants has been banned in into the atmosphere. the United States. 908 Chapter 20 Chemistry in the Atmosphere Because of their relative inertness, the CFCs slowly diffuse unchanged up to the stratosphere, where UV radiation of wavelengths between 175 nm and 220 nm causes them to decompose: CFCl3 ¡ CFCl2 1 Cl CF2Cl2 ¡ CF2Cl 1 Cl It can take years for CFCs to reach the The reactive chlorine atoms then undergo the following reactions: stratosphere. Cl is a homogeneous catalyst. Cl 1 O3 ¡ ClO 1 O2 (20.4) ClO 1 O ¡ Cl 1 O2 (20.5) The overall result [sum of Equations (20.4) and (20.5)] is the net removal of an O3 molecule from the stratosphere: O3 1 O ¡ 2O2 (20.6) The oxygen atoms in Equation (20.5) are supplied by the photochemical decomposition of molecular oxygen and ozone described earlier. Note that the Cl atom plays the role of a catalyst in the reaction mechanism scheme represented by Equations (20.4) and (20.5) because it is not used up and therefore can take part in many such reactions. One Cl atom can destroy up to 100,000 O3 molecules before it is removed by some other reaction. The ClO (chlorine monoxide) species is an intermediate because it is produced in the first elementary step [Equation (20.4)] and consumed in the second step [Equation (20.5)]. The preceding mechanism for the destruction of ozone has been supported by the detection of ClO in the stratosphere in recent years (Figure 20.7). As can be seen, the concentration of O3 decreases in regions that have high amounts of ClO. Another group of compounds that can destroy stratospheric ozone are the nitrogen oxides, generally denoted as NOx. (Examples of NOx are NO and NO2.) These com- pounds come from the exhausts of high-altitude supersonic aircraft and from human and natural activities on Earth. Solar radiation decomposes a substantial amount of the other nitrogen oxides to nitric oxide (NO), which participates in the destruction of ozone as follows: O3 ¡ O2 1 O NO 1 O3 ¡ NO2 1 O2 NO2 1 O ¡ NO 1 O2 Overall: 2O3 ¡ 3O2 Figure 20.7 The variations in the concentrations of ClO and O3 2.5 with latitude. O3 Chlorine monoxide (ppb by volume) 1.0 2.0 Ozone (ppm by volume) 1.5 0.5 1.0 ClO 0.5 0 0 63°S 72°S Latitude 20.3 Depletion of Ozone in the Stratosphere 909 Figure 20.8 In recent years, scientists have found that the ozone layer in the stratosphere over the South Pole has become thinner. This map, based on data collected over a number of years, shows the depletion of ozone in red. (Source: NASA/Goddard Space Flight Center) In this case, NO is the catalyst and NO2 is the intermediate. Nitrogen dioxide also reacts with chlorine monoxide to form chlorine nitrate: ClO 1 NO2 ¡ ClONO2 Chlorine nitrate is relatively stable and serves as a “chlorine reservoir,” which plays a role in the depletion of the stratospheric ozone over the North and South Poles. Polar Ozone Holes In the mid-1980s, evidence began to accumulate that an “Antarctic ozone hole” devel- oped in late winter, depleting the stratospheric ozone over Antarctica by as much as 50 percent (Figure 20.8). In the stratosphere, a stream of air known as the “polar vortex” circles Antarctica in winter. Air trapped within this vortex becomes extremely cold during the polar night. This condition leads to the formation of ice particles known as polar stratospheric clouds (PSCs) (Figure 20.9). Acting as a heterogeneous Figure 20.9 Polar stratospheric clouds containing ice particles can catalyze the formation of Cl atoms and lead to the destruction of ozone. 910 Chapter 20 Chemistry in the Atmosphere catalyst, these PSCs provide a surface for reactions converting HCl (emitted from Earth) and chlorine nitrate to more reactive chlorine molecules: HCl 1 ClONO2 ¡ Cl2 1 HNO3 By early spring, the sunlight splits molecular chlorine into chlorine atoms Cl2 1 hn ¡ 2Cl which then attack ozone as shown earlier. The situation is not as severe in the warmer Arctic region, where the vortex does not persist quite as long. Studies have shown that ozone levels in this region have declined between 4 and 8 percent in the past decade. Volcanic eruptions, such as that of Mount Pinatubo in the Philippines in 1991, inject large quantities of dust-sized particles and sulfuric acid aerosols (see p. 547) into the atmosphere. These particles can perform the same catalytic function as the ice crystals at the South Pole. As a result, the Arctic hole is expected to grow larger during the next few years. Recognizing the serious implications of the loss of ozone in the stratosphere, nations throughout the world have acknowledged the need to drastically curtail or totally stop the production of CFCs. In 1978 the United States was one of the few countries to ban the use of CFCs in hair sprays and other aerosols. An international treaty—the Montreal protocol—was signed by most industrialized nations in 1987, setting targets for cutbacks in CFC production and the complete elimination of these substances by the year 2000. While some progress has been made in this respect, many nations have not been able to abide by the treaty because of the importance of CFCs to their economies. Recycling could play a significant supplementary role in preventing CFCs already in appliances from escaping into the atmosphere. As Figure 20.6 shows, more than half of the CFCs in use are recoverable. An intense effort is under way to find CFC substitutes that are effective refriger- ants but not harmful to the ozone layer. One of the promising candidates is hydro- chlorofluorocarbon 134a, or HCFC-134a (CH2FCF3). The presence of the hydrogen atoms makes the compound more susceptible to oxidation in the lower atmosphere, The OH radical is formed by a series of so that it never reaches the stratosphere. Specifically, it is attacked by the hydroxyl complex reactions in the troposphere that are driven by sunlight. radical in the troposphere: CH2FCF3 1 OH ¡ CHFCF3 1 H2O The CHFCF3 fragments react with oxygen, eventually decomposing to CO2, water, and hydrogen fluoride that are removed by rainwater. Although it is not clear whether the CFCs already released to the atmosphere will eventually result in catastrophic damage to life on Earth, it is conceivable that the depletion of ozone can be slowed by reducing the availability of Cl atoms. Indeed, some chemists have suggested sending a fleet of planes to spray 50,000 tons of ethane (C2H6) or propane (C3H8) high over the South Pole in an attempt to heal the hole in the ozone layer. Being a reactive species, the chlorine atom would react with the hydrocarbons as follows: Cl 1 C2H6 ¡ HCl 1 C2H5 Cl 1 C3H8 ¡ HCl 1 C3H7 The products of these reactions would not affect the ozone concentration. A less realistic plan is to rejuvenate the ozone layer by producing large quantities of ozone and releas- ing it into the stratosphere from airplanes. Technically this solution is feasible, but it would be enormously costly and it would require the collaboration of many nations. 20.4 Volcanoes 911 Having discussed the chemistry in the outer regions of Earth’s atmosphere, we will focus in Sections 20.4 through 20.8 on events closer to us, that is, in the troposphere. 20.4 Volcanoes Volcanic eruptions, Earth’s most spectacular natural displays of energy, are instru- mental in forming large parts of Earth’s crust. The upper mantle, immediately under the crust, is nearly molten. A slight increase in heat, such as that generated by the movement of one crustal plate under another, melts the rock. The molten rock, called magma, rises to the surface and generates some types of volcanic eruptions (Figure 20.10). An active volcano emits gases, liquids, and solids. The gases spewed into the atmosphere include primarily N2, CO2, HCl, HF, H2S, and water vapor. It is estimated that volcanoes are the source of about two-thirds of the sulfur in the air. On the slopes of Mount St. Helens, which last erupted in 1980, deposits of elemental sulfur are visible near the eruption site. At high temperatures, the hydrogen sulfide gas given off by a volcano is oxidized by air: 2H2S(g) 1 3O2 (g) ¡ 2SO2 (g) 1 2H2O(g) Some of the SO2 is reduced by more H2S from the volcano to elemental sulfur and water: Sulfur deposits at a volcanic site. 2H2S(g) 1 SO2 (g) ¡ 3S(s) 1 2H2O(g) The rest of the SO2 is released into the atmosphere, where it reacts with water to form acid rain (see Section 20.6). The tremendous force of a volcanic eruption carries a sizable amount of gas into the stratosphere. There SO2 is oxidized to SO3, which is eventually converted to sul furic acid aerosols in a series of complex mechanisms. In addition to destroying ozone in the stratosphere (see p. 910), these aerosols can also affect climate. Because the stratosphere is above the atmospheric weather patterns, the aerosol clouds often persist for more than a year. They absorb solar radiation and thereby cause a drop in tem- perature at Earth’s surface. However, this cooling effect is local rather than global, because it depends on the site and frequency of volcanic eruptions. Figure 20.10 A volcanic eruption on the island of Hawaii. 912 Chapter 20 Chemistry in the Atmosphere 20.5 The Greenhouse Effect A dramatic illustration of the greenhouse Although carbon dioxide is only a trace gas in Earth’s atmosphere, with a concentra- effect is found on Venus where the atmosphere is 97 percent CO2 and the tion of about 0.033 percent by volume (see Table 20.1), it plays a critical role in atmospheric pressure is 9 3 106 Pa controlling our climate. The so-called greenhouse effect describes the trapping of heat (equivalent to 89 atm). The surface temperature of Venus is about 730 K! near Earth’s surface by gases in the atmosphere, particularly carbon dioxide. The glass roof of a greenhouse transmits visible sunlight and absorbs some of the outgo- ing infrared (IR) radiation, thereby trapping the heat. Carbon dioxide acts somewhat like a glass roof, except that the temperature rise in the greenhouse is due mainly to the restricted air circulation inside. Calculations show that if the atmosphere did not contain carbon dioxide, Earth would be 30°C cooler! Figure 20.11 shows the carbon cycle in our global ecosystem. The transfer of carbon dioxide to and from the atmosphere is an essential part of the carbon cycle. Carbon dioxide is produced when any form of carbon or a carbon-containing com- pound is burned in an excess of oxygen. Many carbonates give off CO2 when heated, and all give off CO2 when treated with acid: CaCO3 (s) ¡ CaO(s) 1 CO2 (g) CaCO3 (s) 1 2HCl(aq) ¡ CaCl2 (aq) 1 H2O(l) 1 CO2 (g) Carbon dioxide in atmosphere Plant respiration Assimilation by plants Soil Animal respiration respiration Litter Dead organisms Root respiration Decomposition Figure 20.11 The carbon cycle. 20.5 The Greenhouse Effect 913 Figure 20.12 The incoming radiation from the sun and the outgoing radiation from Earth’s Incoming solar radiation surface. Energy Outgoing terrestrial radiation 5000 15,000 25,000 Wavelength (nm) Carbon dioxide is also a by-product of the fermentation of sugar: yeast C6H12O6 (aq) ¡ 2C2H5OH(aq) 1 2CO2 (g) glucose ethanol Carbohydrates and other complex carbon-containing molecules are consumed by ani- mals, which respire and release CO2 as an end product of metabolism: C6H12O6 (aq) 1 6O2 (g) ¡ 6CO2 (g) 1 6H2O(l) As mentioned earlier, another major source of CO2 is volcanic activity. Carbon dioxide is removed from the atmosphere by photosynthetic plants and certain microorganisms: 6CO2 (g) 1 6H2O(l) ¡ C6H12O6 (aq) 1 6O2 (g) This reaction requires radiant energy (visible light). After plants and animals die, the carbon in their tissues is oxidized to CO2 and returns to the atmosphere. In addition, there is a dynamic equilibrium between atmospheric CO2 and carbonates in the oceans and lakes. The solar radiant energy received by Earth is distributed over a band of wave- lengths between 100 and 5000 nm, but much of it is concentrated in the 400- to Stable form 700-nm range, which is the visible region of the spectrum (Figure 20.12). By contrast, the thermal radiation emitted by Earth’s surface is characterized by wavelengths longer than 4000 nm (IR region) because of the much lower average surface temperature Stretched compared to that of the sun. The outgoing IR radiation can be absorbed by water and carbon dioxide, but not by nitrogen and oxygen. All molecules vibrate, even at the lowest temperatures. The energy associated with molecular vibration is quantized, much like the electronic energies of atoms and Compressed molecules. To vibrate more energetically, a molecule must absorb a photon of a specific wavelength in the IR region. First, however, its dipole moment must change Figure 20.13 Vibrational motion of a diatomic molecule. Chemical during the course of a vibration. [Recall that the dipole moment of a molecule is the bonds can be stretched and product of the charge and the distance between charges (see p. 423).] Figure 20.13 compressed like a spring. 914 Chapter 20 Chemistry in the Atmosphere (a) (b) Figure 20.15 Two of the four ways a carbon dioxide molecule can vibrate. The vibration in (a) does not result in a change in dipole moment, but the vibration in (b) renders the molecule IR active. shows how a diatomic molecule can vibrate. If the molecule is homonuclear like N2 and O2, there can be no change in the dipole moment; the molecule has a zero dipole moment no matter how far apart or close together the two atoms are. We call such molecules IR-inactive because they cannot absorb IR radiation. On the other hand, all heteronuclear diatomic molecules are IR-active; that is, they all can absorb IR radiation because their dipole moments constantly change as the bond lengths change. A polyatomic molecule can vibrate in more than one way. Water, for example, can vibrate in three different ways, as shown in Figure 20.14. Because water is a polar molecule, it is easy to see that any of these vibrations results in a change in dipole moment because there is a change in bond length. Therefore, a H2O molecule is IR-active. Carbon dioxide has a linear geometry and is nonpolar. Figure 20.15 shows two of the four ways a CO2 molecule can vibrate. One of Figure 20.14 The three different them [Figure 20.15(a)] symmetrically displaces atoms from the center of gravity modes of vibration of a water and will not create a dipole moment, but the other vibration [Figure 20.15(b)] is molecule. Each mode of vibration IR-active because the dipole moment changes from zero to a maximum value in can be imagined by moving the atoms along the arrows and then one direction and then reaches the same maximum value when it changes to the reversing their directions. other extreme position. Upon receiving a photon in the IR region, a molecule of H2O or CO2 is promoted to a higher vibrational energy level: H2O 1 hn ¡ H2O* CO2 1 hn ¡ CO2* (the asterisk denotes a vibrationally excited molecule). These energetically excited molecules soon lose their excess energy either by collision with other molecules or by spontaneous emission of radiation. Part of this radiation is emitted to outer space Electricity and part returns to Earth’s surface. production 35% Although the total amount of water vapor in our atmosphere has not altered Cars and noticeably over the years, the concentration of CO2 has been rising steadily since trucks the turn of the twentieth century as a result of the burning of fossil fuels (petro- 30% leum, natural gas, and coal). Figure 20.16 shows the percentages of CO2 emitted Industry 24% due to human activities in the United States in 1998, and Figure 20.17 shows the variation of carbon dioxide concentration over a period of years, as measured in 11% Hawaii. In the Northern Hemisphere, the seasonal oscillations are caused by removal of carbon dioxide by photosynthesis during the growing season and its Residential heating buildup during the fall and winter months. Clearly, the trend is toward an increase in CO2. The current rate of increase is about 1 ppm (1 part CO2 per million parts Figure 20.16 Sources of carbon dioxide emission in the air) by volume per year, which is equivalent to 9 3 109 tons of CO2! Scientists United States. Note that not all have estimated that by the year 2014 the CO2 concentration will exceed preindus- of the emitted CO2 enters the trial levels by about 40 percent. atmosphere. Some of it is taken up by carbon dioxide “sinks,” In addition to CO2 and H2O, other greenhouse gases, such as the CFCs, CH4, such as the ocean. NOx, and N2O also contribute appreciably to the warming of the atmosphere. 20.5 The Greenhouse Effect 915 400 CO2 concentration (ppm by volume) 380 360 340 320 1960 1970 1980 1990 2000 2010 Figure 20.17 Yearly variation of carbon dioxide concentration at Mauna Loa, Hawaii. The general trend clearly points to an increase of carbon dioxide in the atmosphere. In May 2013, the mean concentration recorded was 400 ppm. Figure 20.18 shows the gradual increase in temperature over the years and Figure 20.19 shows the relative contributions of the greenhouse gases to global warming. It is predicted by some meteorologists that should the buildup of greenhouse gases The difference in global temperatures between today and the last ice age is continue at its current rate, Earth’s average temperature will increase by about 1° to only 4–5°C. 3°C in this century. Although a temperature increase of a few degrees may seem insignificant, it is actually large enough to disrupt the delicate thermal balance on Earth and could cause glaciers and icecaps to melt. Consequently, the sea level would rise and coastal areas would be flooded. To combat the greenhouse effect, we must lower carbon dioxide emission. This As more nations industrialize, the production of CO2 will increase can be done by improving energy efficiency in automobiles and in household heating appreciably. and lighting, and by developing nonfossil fuel energy sources, such as photovoltaic 0.6 Figure 20.18 The change in Annual average global temperature from 1850 to Five year average 2008. (Source: NASA Goddard Institute for 0.4 Space Studies) Temperature deviation (⬚C) 0.2 0 ⫺0.2 ⫺0.4 ⫺0.6 1860 1880 1900 1920 1940 1960 1980 2000 916 Chapter 20 Chemistry in the Atmosphere cells. Nuclear energy is a viable alternative, but its use is highly controversial due to the difficulty of disposing of radioactive waste and the fact that nuclear power stations CO2 are more prone to accidents than conventional power stations (see Chapter 19). The 55% proposed phasing out of CFCs, the most potent greenhouse gases, will help to slow down the warming trend. The recovery of methane gas generated at landfills and the N2O reduction of natural gas leakages are other steps we could take to control CO2 emis- 6% sion. Finally, the preservation of the Amazon jungle, tropical forests in Southeast Asia, CH4 CFCs 15% and other large forests is vital to maintaining the steady-state concentration of CO2 24% in the atmosphere. Converting forests to farmland for crops and grassland for cattle may do irreparable damage to the delicate ecosystem and permanently alter the cli- mate pattern on Earth. Figure 20.19 Contribution to global warming by various greenhouse gases. The concentrations of CFCs and Example 20.2 methane are much lower than that of carbon dioxide. However, because they can absorb IR Which of the following gases qualify as a greenhouse gas: CO, NO, NO2, Cl2, H2, Ne? radiation much more effectively than CO2, they make an Strategy To behave as a greenhouse gas, either the molecule must possess a dipole appreciable contribution to moment or some of its vibrational motions must generate a temporary dipole moment. the overall warming effect. These conditions immediately rule out homonuclear diatomic molecules and atomic species. Solution Only CO, NO, and NO2, which are all polar molecules, qualify as greenhouse gases. Both Cl2 and H2 are homonuclear diatomic molecules, and Ne is Similar problem: 20.36. atomic. These three species are all IR-inactive. Practice Exercise Which of the following is a more effective greenhouse gas: CO or H2O? 20.6 Acid Rain Scientists have known about acid rain Every year acid rain causes hundreds of millions of dollars’ worth of damage to stone since the late nineteenth century, but it has been a public issue for only about buildings and statues throughout the world. The term “stone leprosy” is used by some 30 years. environmental chemists to describe the corrosion of stone by acid rain (Figure 20.20). Acid rain is also toxic to vegetation and aquatic life. Many well-documented cases show dramatically how acid rain has destroyed agricultural and forest lands and killed aquatic organisms (see Figure 15.10). Figure 20.20 The effect of acid rain on the marble statue of George Washington in Washington Square, New York City. The photos were taken 50 years apart (1944–1994). 20.6 Acid Rain 917 Figure 20.21 Mean precipitation pH in the United States in 2009. Most SO2 comes from the midwestern states. Prevailing winds carry the acid droplets formed over the Northeast. Nitrogen oxides also contribute to the acid rain formation. Precipitation in the northeastern United States has an average pH of about 4.3 (Figure 20.21). Because atmospheric CO2 in equilibrium with rainwater would not be expected to result in a pH less than 5.5, sulfur dioxide (SO2) and, to a lesser extent, nitrogen oxides from auto emissions are believed to be responsible for the high acidity of rainwater. Acidic oxides, such as SO2, react with water to give the corresponding acids. There are several sources of atmospheric SO2. Nature itself contributes much SO2 in the form of volcanic eruptions. Also, many metals exist combined with sulfur in nature. Extracting the metals often entails smelting, or roast- ing, the ores—that is, heating the metal sulfide in air to form the metal oxide and SO2. For example, 2ZnS(s) 1 3O2 (g) ¡ 2ZnO(s) 1 2SO2 (g) The metal oxide can be reduced more easily than the sulfide (by a more reactive metal or in some cases by carbon) to the free metal. Although smelting is a major source of SO2, the burning of fossil fuels in industry, in power plants, and in homes accounts for most of the SO2 emitted to the atmosphere. The sulfur content of coal ranges from 0.5 to 5 percent by mass, depending on the source of the coal. The sulfur content of other fossil fuels is similarly variable. Oil from the Middle East, for instance, is low in sulfur, while that from Venezuela has a high sulfur content. To a lesser extent, the nitrogen- containing compounds in oil and coal are converted to nitrogen oxides, which can also acidify rainwater. All in all, some 50 million to 60 million tons of SO2 are released into the atmo- sphere each year! In the troposphere, SO2 is almost all oxidized to H2SO4 in the form of aerosol, which ends up in wet precipitation or acid rain. The mechanism for the conversion of SO2 to H2SO4 is quite complex and not fully understood. The reaction is believed to be initiated by the hydroxyl radical (OH): OH 1 SO2 ¡ HOSO2 The HOSO2 radical is further oxidized to SO3: HOSO2 1 O2 ¡ HO2 1 SO3 918 Chapter 20 Chemistry in the Atmosphere Figure 20.22 Common procedure for removing SO2 from Mostly CO2 and air burning fossil fuel. Powdered limestone decomposes into CaO, which reacts with SO2 to form CaSO3. The remaining SO2 is Smokestack reacted with an aqueous suspension of CaO to form CaSO3. S O2 SO2 Purification chamber CaCO3 CaO CO2 CaO SO2 CaSO3 Aqueous suspension of CaO CaCO3 SO2, CO2 Furnace Air Air Coal CaSO3 The sulfur trioxide formed would then rapidly react with water to form sulfuric acid: SO3 1 H2O ¡ H2SO4 SO2 can also be oxidized to SO3 and then converted to H2SO4 on particles by hetero- geneous catalysis. Eventually, the acid rain can corrode limestone and marble (CaCO3). A typical reaction is CaCO3 (s) 1 H2SO4 (aq) ¡ CaSO4 (s) 1 H2O(l) 1 CO2 (g) Sulfur dioxide can also attack calcium carbonate directly: 2CaCO3 (s) 1 2SO2 (g) 1 O2 (g) ¡ 2CaSO4 (s) 1 2CO2 (g) There are two ways to minimize the effects of SO2 pollution. The most direct approach is to remove sulfur from fossil fuels before combustion, but this is techno- logically difficult to accomplish. A cheaper but less efficient way is to remove SO2 as it is formed. For example, in one process powdered limestone is injected into the power plant boiler or furnace along with coal (Figure 20.22). At high temperatures the following decomposition occurs: CaCO3 (s) ¡ CaO(s) 1 CO2 (g) limestone quicklime The quicklime reacts with SO2 to form calcium sulfite and some calcium sulfate: CaO(s) 1 SO2 (g) ¡ CaSO3 (s) 2CaO(s) 1 2SO2 (g) 1 O2 (g) ¡ 2CaSO4 (s) To remove any remaining SO2, an aqueous suspension of quicklime is injected into a purification chamber prior to the gases’ escape through the smokestack. Quicklime is also added to lakes and soils in a process called liming to reduce their acidity (Figure 20.23). Installing a sulfuric acid plant near a metal ore refining site is also an effective way to cut SO2 emission because the SO2 produced by roasting metal sulfides can be captured for use in the synthesis of sulfuric acid. This is a very sensible way to turn what is a pollutant in one process into a starting material for another process! 20.7 Photochemical Smog 919 Figure 20.23 Spreading calcium oxide (CaO) over acidified soil. This process is called liming. 20.7 Photochemical Smog The word “smog” was coined to describe the combination of smoke and fog that shrouded London during the 1950s. The primary cause of this noxious cloud was sulfur dioxide. Today, however, we are more familiar with photochemical smog, which is formed by the reactions of automobile exhaust in the presence of sunlight. Automobile exhaust consists mainly of NO, CO, and various unburned hydrocar- bons. These gases are called primary pollutants because they set in motion a series of photochemical reactions that produce secondary pollutants. It is the secondary pollutants—chiefly NO2 and O3—that are responsible for the buildup of smog. The heavy use of automobiles is Nitric oxide is the product of the reaction between atmospheric nitrogen and the cause of photochemical smog oxygen at high temperatures inside an automobile engine: formation. N2 (g) 1 O2 (g) ¡ 2NO(g) Once released into the atmosphere, nitric oxide is oxidized to nitrogen dioxide: 2NO(g) 1 O2 (g) ¡ 2NO2 (g) Sunlight causes the photochemical decomposition of NO2 (at a wavelength shorter than 400 nm) into NO and O: NO2 (g) 1 hn ¡ NO(g) 1 O(g) Atomic oxygen is a highly reactive species that can initiate a number of important reactions, one of which is the formation of ozone: O(g) 1 O2 (g) 1 M ¡ O3 (g) 1 M where M is some inert substance such as N2. Ozone attacks the C“C linkage in Ozone plays a dual role in the atmosphere: It is Dr. Jekyll in the stratosphere and rubber: Mr. Hyde in the troposphere. R R R R R R G D G DO G D H2O G D CPC O3 88n C C 88n CPO OPC H2O2 D G DG G D G R R R OOOD R R R 920 Chapter 20 Chemistry in the Atmosphere Figure 20.24 Typical variations with time in concentration of air pollutants on a smoggy day. Relative concentrations Hydrocarbons NO2 O3 NO 4 6 8 10 12 2 4 6 A.M. Noon P.M. where R represents groups of C and H atoms. In smog-ridden areas, this reaction can cause automobile tires to crack. Similar reactions are also damaging to lung tissues and other biological substances. Ozone can be formed also by a series of very complex reactions involving unburned hydrocarbons, nitrogen oxides, and oxygen. One of the products of these reactions is peroxyacetyl nitrate, PAN: CH3OCOOOOONO2 B O PAN is a powerful lachrymator, or tear producer, and causes breathing difficulties. Figure 20.24 shows typical variations with time of primary and secondary pol- lutants. Initially, the concentration of NO2 is quite low. As soon as solar radiation penetrates the atmosphere, more NO2 is formed from NO and O2. Note that the PAN concentration of ozone remains fairly constant at a low level in the early morning hours. As the concentration of unburned hydrocarbons and aldehydes increases in the air, the concentrations of NO2 and O3 also rise rapidly. The actual amounts, of course, depend on the location, traffic, and weather conditions, but their presence is always accompanied by haze (Figure 20.25). The oxidation of hydrocarbons produces various organic intermediates, such as alcohols and carboxylic acids, which are all less volatile than the hydrocarbons themselves. These substances eventually condense into small droplets of liquid. The dispersion of these droplets in air, called aerosol, scatters sunlight and reduces visibility. This interaction also makes the air look hazy. As the mechanism of photochemical smog formation has become better under- stood, major efforts have been made to reduce the buildup of primary pollutants. Most automobiles now are equipped with catalytic converters designed to oxidize CO and unburned hydrocarbons to CO2 and H2O and to reduce NO and NO2 to N2 and O2 (see Section 13.6). More efficient automobile engines and better public transportation systems would also help to decrease air pollution in urban areas. A recent techno- logical innovation to combat photochemical smog is to coat automobile radiators and air conditioner compressors with a platinum catalyst. So equipped, a running car can purify the air that flows under the hood by converting ozone and carbon monoxide The haze over the Smoky Mountains to oxygen and carbon dioxide: is caused by aerosols produced by the oxidation of hydrocarbons Pt emitted by pine trees. O3 (g) 1 CO(g) ¡ O2 (g) 1 CO2 (g) 20.8 Indoor Pollution 921 Figure 20.25 A smoggy day in Beijing. In a city like Los Angeles, where the number of miles driven in one day equals nearly 300 million, this approach would significantly improve the air quality and reduce the “high-ozone level” warnings frequently issued to its residents. In fact, a drive on the freeway would help to clean up the air! 20.8 Indoor Pollution Difficult as it is to avoid air pollution outdoors, it is no easier to avoid indoor pollution. The air quality in homes and in the workplace is affected by human activities, by con- struction materials, and by other factors in our immediate environment. The common indoor pollutants are radon, carbon monoxide and carbon dioxide, and formaldehyde. The Risk from Radon In a highly publicized case in the mid-1980s, an employee reporting for work at the Limerick Nuclear Power Plant in Pennsylvania set off the plant’s radiation monitor. Astonishingly, the source of his contamination turned out not to be the plant, but radon in his home! A lot has been said and written about the potential dangers of radon as an air pol- lutant. Just what is radon? Where does it come from? And how does it affect our health? Radon is a member of Group 8A (the noble gases). It is an intermediate product of the radioactive decay of uranium-238. All isotopes of radon are radioactive, but The uranium decay series is discussed in Chapter 19. radon-222 is the most hazardous because it has the longest half-life—3.8 days. Radon, 922 Chapter 20 Chemistry in the Atmosphere > 8 pCi/L 4-8 pCi/L 2-4 pCi/L < 2 pCi/L Figure 20.26 Map of radon emission in the United States measured in picocuries per liter of air. which accounts for slightly over half the background radioactivity on Earth, is gener- ated mostly from the phosphate minerals of uranium (Figure 20.26). Since the 1970s, high levels of radon have been detected in homes built on reclaimed land above uranium mill tailing deposits. The colorless, odorless, and tasteless radon After cigarette smoking, radon is the gas enters a building through tiny cracks in the basement floor (Figure 20.27). It is leading cause of lung cancer in the United States. It is responsible for slightly soluble in water, so it can be spread in different media. Radon-222 is an perhaps 20,000 deaths per year. α-emitter. When it decays, it produces radioactive polonium-214 and polonium-218, which can build up to high levels in an enclosed space. These solid radioactive particles can adhere to airborne dust and smoke, which are inhaled into the lungs and deposited in the respiratory tract. Over a long period of time, the α particles emitted by polonium and its decay products, which are also radioactive, can cause lung cancer. What can be done to combat radon pollution indoors? The first step is to measure the radon level in the basement with a reliable test kit. Short-term and long-term kits are available (Figure 20.28). The short-term tests use activated charcoal (that is, heat- treated charcoal) to collect the decay products of radon over a period of several days. The container is sent to a laboratory where a technician measures the radioactivity (γ rays) from radon-decay products lead-214 and bismuth-214. Knowing the length of exposure, the lab technician back-calculates to determine radon concentration. The long- term test kits use a piece of special polymer film on which an α particle will leave a “track.” After several months’ exposure, the film is etched with a sodium hydroxide solution and the number of tracks counted. Knowing the length of exposure enables the technician to calculate the radon concentration. If the radon level is unacceptably high, Basement Radon h Uranium n Radium Figure 20.27 Radon usually enters houses through the foundation or basement walls. Figure 20.28 Home radon detectors: Long-term track etch (left) and short-term charcoal canister (right). 20.8 Indoor Pollution 923 then the house must be regularly ventilated. This precaution is particularly important in recently built houses, which are well insulated. A more effective way to prevent radon pollution is to reroute the gas before it gets into the house, for example, by installing a ventilation duct to draw air from beneath the basement floor to the outside. Currently there is considerable controversy regarding the health effects of radon. The first detailed studies of the effects of radon on human health were carried out in the 1950s when it was recognized that uranium miners suffered from an abnormally high incidence of lung cancer. Some scientists have challenged the validity of these studies because the miners were also smokers. It seems quite likely that there is a syn- ergistic effect between radon and smoking on the development of lung cancer. Radon- decay products will adhere not only to tobacco tar deposits in the lungs, but also to the solid particles in cigarette smoke, which can be inhaled by smokers and nonsmokers. More systematic studies are needed to evaluate the environmental impact of radon. In the meantime, the Environmental Protection Agency (EPA) has recommended remedial action where the radioactivity level due to radon exceeds 4 pico-curies (pCi) per liter of air. (A curie corresponds to 3.70 3 1010 disintegrations of radioactive nuclei per second; a picocurie is a trillionth of a curie, or 3.70 3 1022 disintegrations per second.) Example 20.3 The half-life of Rn-222 is 3.8 days. Starting with 1.0 g of Rn-222, how much will be left after 10 half-lives? Recall that radioactive decays obey first-order kinetics. Strategy All radioactive decays obey first-order kinetics. Therefore, its half-life is independent of the initial concentration. Solution After one half-life, the amount of Rn left is 0.5 3 1.0 g, or 0.5 g. After two half-lives, only 0.25 g of Rn remains. Generalizing the fraction of the isotope left after n half lives as (1/2)n, where n 5 10, we write 1 10 quantity of Rn-222 left 5 1.0 g 3 a b 2 5 9.8 3 1024 g An alternative solution is to calculate the first-order rate constant from the half-life. Next, use Equation (13.3) to calculate the concentration of radon after 10 half-lives. Try it. Similar problem: 20.73. Practice Exercise The concentration of Rn-222 in the basement of a house is 1.8 3 1026 mol/L. Assume the air remains static and calculate the concentration of the radon after 2.4 days. Carbon Dioxide and Carbon Monoxide Both carbon dioxide (CO2) and carbon monoxide (CO) are products of combustion. In the presence of an abundant supply of oxygen, CO2 is formed; in a limited supply of oxygen, both CO and CO2 are formed. The indoor sources of these gases are gas cooking ranges, woodstoves, space heaters, tobacco smoke, human respiration, and exhaust fumes from cars (in garages). Carbon dioxide is not a toxic gas, but it does have an asphyxiating effect (see Chemistry in Action on p. 531). In airtight buildings, the concentration of CO2 can reach as high as 2000 ppm by volume (compared with 300 ppm outdoors). Workers exposed to high concentrations of CO2 in skyscrapers and other sealed environments become fatigued more easily and have difficulty con- centrating. Adequate ventilation is the solution to CO2 pollution. Like CO2, CO is a colorless and odorless gas, but it differs from CO2 in that it is highly poisonous. The toxicity of CO lies in its unusual ability to bind very strongly to hemoglobin, the oxygen carrier in blood. Both O2 and CO bind to the Fe(II) ion 924 Chapter 20 Chemistry in the Atmosphere in hemoglobin, but the affinity of hemoglobin for CO is about 200 times greater than that for O2 (see Chapter 25). Hemoglobin molecules with tightly bound CO (called carboxyhemoglobin) cannot carry the oxygen needed for metabolic processes. A small amount of CO intake can cause drowsiness and headache; death may result when about half the hemoglobin molecules are complexed with CO. The best first-aid response to CO poisoning is to remove the victim immediately to an area with a plentiful oxygen supply or to give mouth-to-mouth resuscitation. Formaldehyde Formaldehyde (CH2O) is a rather disagreeable-smelling liquid used as a preservative for laboratory specimens. Industrially, formaldehyde resins are used as bonding agents in building and furniture construction materials such as plywood and particle board. In addition, urea-formaldehyde insulation foams are used to fill wall cavities. The resins and foams slowly break down to release free formaldehyde, especially under acid and humid conditions. Low concentrations of formaldehyde in the air can cause drowsiness, nausea, headaches, and other respiratory ailments. Laboratory tests show that breathing high concentrations of formaldehyde can induce cancers in animals, CH2O and it is now also classified as a human carcinogen. The safe standard of formaldehyde in indoor air has been set at 0.1 ppm by volume. Because formaldehyde is a reducing agent, devices have been constructed to remove it by means of a redox reaction. Indoor air is circulated through an air purifier contain- ing an oxidant such as Al2O3/KMnO4, which converts formaldehyde to the less harmful and less volatile formic acid (HCOOH). Proper ventilation is the best way to remove formaldehyde. However, care should be taken not to remove the air from a room too quickly without replenishment, because a reduced pressure would cause the formalde- hyde resins to decompose faster, resulting in the release of more formaldehyde. Summary of Facts & Concepts 1. Earth’s atmosphere is made up mainly of nitrogen and 5. Carbon dioxide’s ability to absorb infrared radiation en- oxygen, plus a number of other trace gases. The chem- ables it to trap some of the outgoing heat from Earth, ical processes that go on in the atmosphere are influ- warming its surface. Other gases such as the CFCs and enced by solar radiation, volcanic eruption, and human methane also contribute to global warming. activities. 6. Sulfur dioxide, and to a lesser extent nitrogen oxides, 2. In the outer regions of the atmosphere the bombard- generated mainly from the burning of fossil fuels and ment of molecules and atoms by solar particles gives from the roasting of metal sulfides, causes acid rain. rise to auroras. The glow on space shuttles is caused 7. Photochemical smog is formed by the photochemical by excitation of molecules adsorbed on the shuttles’ reaction of automobile exhaust in the presence of sun- surface. light. It is a complex reaction involving nitrogen oxides, 3. Ozone in the stratosphere absorbs harmful UV radiation ozone, and hydrocarbons. in the 200- to 300-nm range and protects life under- 8. Indoor air pollution is caused by radon, a radioactive neath. For many years, chlorofluorocarbons have been gas formed during uranium decay; carbon monoxide destroying the ozone layer. and carbon dioxide, products of combustion; and form- 4. Volcanic eruptions can lead to air pollution, deplete aldehyde, a volatile organic substance released from ozone in the stratosphere, and affect climate. resins used in construction materials. Key Words Greenhouse effect, p. 912 Mesosphere, p. 903 Photochemical smog, p. 919 Thermosphere, p. 903 Ionosphere, p. 903 Nitrogen fixation, p. 901 Stratosphere, p. 903 Troposphere, p. 902