Organic Intermediates PDF
Document Details
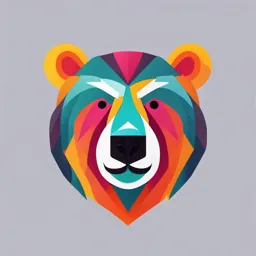
Uploaded by DeliciousMulberryTree
VIT Vellore
Tags
Summary
This document provides an overview of organic intermediates, including carbocations, carbanions, free radicals, carbenes, and nitrenes. It details important factors influencing the stability of carbocations, such as inductive effects, hyperconjugation, resonance, and steric effects.
Full Transcript
MOD No. 3 VIT-Vellore-14 1 Reaction Intermediates Most of the organic reaction occur through the involvement of certain chemical species. These are generally short – lived (10-6 second to a few seconds ) and highly reactive and hence cannot be isolated. These short –liv...
MOD No. 3 VIT-Vellore-14 1 Reaction Intermediates Most of the organic reaction occur through the involvement of certain chemical species. These are generally short – lived (10-6 second to a few seconds ) and highly reactive and hence cannot be isolated. These short –lived highly reactive chemical species through which the organic reactions occur are called reactive intermediates. Some examples of reaction intermediates are, 2 Carbocations, Carbanions, Free Radicals, Carbenes and Nitrenes There are four types of organic species in which a carbon atom has a valence of only 2 or 3. They are usually very short-lived- mostly existing only as intermediates that are quickly converted to more stable molecules. However, some are more stable than others and fairly stable examples have been prepared of three of the four types. The four types of species are carbocations, free radicals, carbanions, and carbenes. Of the four, only carbanions have a complete octet around the carbon. There are many other organic ions and radicals with charges and unpaired electrons on atoms other than carbon-but we will discuss only nitrenes (E), the nitrogen analogs of carbenes. 3 Carbocation A group of organic species having a positively charged carbon atom bearing only six bonded electrons are called carbocations. For example, Structure: The central carbon atom of a carbocation (or carbonium ion) is sp2 hybridized. The three sp2 orbitals are utilized in making bonds to three substituents. In order to minimize repulsion between the bonding electron pairs (I.e. to afford maximum separation of these electron pairs) a carbocation possesses a planar configuration with bond angles of 120o. The empty p orbital is perpendicular to the plane. Carbocations very highly reactive species, because of having a strong tendency to complete the octet of the electron-deficient carbon. 4 Classification: Carbocations are classified as primary (1o), secondary(2o), and tertiary (3o) on the basis of the number of the number of carbon atoms (one, two, or three) directly attached to the positively charged carbon. For example, The factors responsible for carbocation stability are – (i) Inductive effect, (ii) Hyperconjugative effect, (iii) Resonance effect, (iv) Steric effect and (v) constituting an aromatic system. (i) Inductive effect A charge-dispersing factor stabilizes an ion. The electron-releasing inductive effect (+I) exerted by an alkyl group attached to the positive carbon of a carbocation neutralizes the charge partially. As a consequence, the charge becomes dispersed over the alkyl groups and the system becomes stabilized. For example, the methyl groups in isopropyl cation stabilize the system through their +I effects. The stabilities of carbocations increase with increasing the number of alkyl groups attached to the positive carbon. 5 (ii) Hyperconjugative effect An alkyl group may also reduce the positive charge of a carbocation by hyperconjugative electron- release. The charge becomes dispersed over the a-hydrogens and consequently, the system becomes stabilized. Hyperconjugation in ethyl cation, for example, occurs as follows: As the number of α-hydrogens, i.e., the number of hyperconjugative forms increases, the stability of carbocations increases. Hence, the order of stabilities of methyl substituted carbocations is : (iii) Resonance effect Resonance is a major factor influencing the stability of carbocations. When the positive carbon of a carbocation is a to a double bond, effective charge delocalization with consequent stabilization occurs. Allyl and benzyl cations, for examples, are found to be highly stabilized by resonance. 6 (iv) Steric effect Steric effect causes an increase in stability of tertiary carbocations having bulky alkyl groups. For example, the substituents in tri-isopropyl cation (having planar arrangement with 120° angles) are far apart from each other and so there is no steric interference among them. However, if this carbocation is added to a nucleophile, i.e., if a change of hybridization of the central carbon atom from sp2 (trigonal) to sp3 (tetrahedral) takes place, the bulky isopropyl groups will be pushed together. This will result in a steric strain (B strain) in the product molecule. Because of this, the carbocation is much reluctant to react with a nucleophile, that is, its stability is enhanced due to steric reason. (v) constituting an aromatic system The vacant p orbital of a carbocation may be involved in constituting a planar (4n +2)π electron system. where n = 0,1,2.... etc., i.e., a carbocation may be stabilized by constituting an aromatic system. Cycloheptatrienyl cation, for example, is unusually stable because it is a planar 6π electron system and aromatic. 7 Question: Arrange the following carbocations in order of their increasing stability with reasoning: Ans: The carbocation I is stabilized by the +I effects of three -CH3 groups and hyperconjugative effect involving nine c-H atoms. The carbocation III is similarly stabilized by +I effect of three ring bonds. However, it is not stabilized by hyperconjugation because formation of a double bond at the bridgehead position is not possible (Bredt's rule). Again, the carbocation suffers from angle strain because the angle between bonds is somewhat less than the sp2 bond angle, i.e., 120". So, the carbocation II, although a 3° one, is less stable than the 3° carbocation I. The carbocation II is the most stable one because it is highly stabilized by resonance and also by both inductive and hyperconjugative effects of two methyl groups. Hence, the order of their increasing stability is: 8 Carbanion The species containing negatively charged carbon atom are known as carbanions. For example, Structure: The central carbon atom of a carbanion is sp3 hybridized. it is surrounded by three bonding pairs and one unshared pair of electrons which occupies an sp3 orbital. Thus, a carbanion is expected to have the tetrahedral shape. However, the shape is not exactly that of a tetrahedron. It is found to have the pyramidal shape. Since the repulsion between the unshared pair and any bonding pair is greater than the repulsion between any two bonding pairs, the angle between two bonding pairs (i.e., two sp3- σ bonds) is slightly less than the normal tetrahedral value of 109.5° and because of this, a carbanion appears to be shaped like a pyramid with the negative carbon at the apex and the three groups at the corners of a triangular base. However, the resonance-stabilized carbanions, such as allylic and benzylic carbanions are sp2 hybridized and they assume trigonal planar structure. 9 The factors responsible for carbanion stability are - The structural features responsible mainly for the increased stability of carbanions are : (i) the amount of s character of the carbanion carbon atom, (ii) inductive electron withdrawal, (iii) conjugation of the non-bonding electron pair with an unsaturated system, and (iv) constituting an aromatic system. (i) The amount of s character of the carbanion carbon atom An s orbital is closer to the nucleus than the p orbital in a given main quantum level and it possesses lower energy. An electron pair in an orbital having large s character is, therefore, more tightly held by the nucleus and hence of lower energy than an electron pair in an orbital having small s character. Hence, a carbanion at an sp hybridized (50% s character) carbon atom is more stable than a carbanion at an sp2 hybridized (33.33% s character) carbon atom, which in turn is more stable than a carbanion at an sp3 hybridized (25% s character) carbon atom. Thus, the order of carbanion stability is: (ii) inductive electron withdrawal Groups having electron-withdrawing inductive effects (H) stabilize a carbanion by dispersing the negative charge. In a nitrogen ylide, for example, the carbanion is stabilized by the -i effect of the adjacent positive nitrogen. 10 (iii) Conjugation of the non-bonding electron pair with an unsaturated system Where there is a double or triple bond a to the carbanion carbon atom, the anion is stabilized by delocalization of its negative charge with the t orbitals of the multiple bond. Thus, allylic and benzylic carbanions and the carbanions attached to the functional groups such as -NO2, -C≡N, >C=O, etc. are stabilized by resonance. (iv) constituting an aromatic system The unshared pair of a carbanion may be involved in constituting a planar (4n + 2)π electron system where n=0, 1, 2... etc., i.e., a carbanion may be stabilized by constituting an aromatic system Cyclopentadienyl anion, for example. is unusually stable because it is a 6π electron system and aromatic. 11 Question: Give the order of stability of the following simple carbanions : Ans: Because of the destabilizing influence of the electron-donating inductive effect of alkyl groups, the order of stabilities of these simple carbanions is as follows: Question: Arrange the following carbanions in each of the following series in order or increasing stability: Ans: The order of increasing stability of these carbanions is: 12 continued The electron-releasing methyl groups of isopropyl anion (I) intensify the negative charge on carbon and make it less stable than methyl anion (III) where there is no possibility of charge intensification. The external orbitals (orbitals directed to the outside bonds) in cyclopropane have larger (33%) s character i.e., they are approximately sp2 orbitals. Because of this, the unshared pair in cyclopropyl anion (IV) is more tightly held with the carbon nucleus than the electrons in methyl anion (III) that occupies an sp3 orbital (25% s character). Consequently, the former anion is more stable than the latter. In vinyl anion (VI), the unshared pair occupies a sp orbital (33.33% s character) and so this anion is somewhat more stable than cyclopropyl anion (IV). The charge in allyl anion (II) is delocalized by resonance with the adjacent double bond and so it is more stable than vinyl anion (VI) in which the charge is localized. Since the unshared pair in cyclopentadienyl anion (V) is involved in forming an aromatic system, charge delocalization and consequent stabilization is far greater for this anion than for allyl anion. 13 Radical Homolytic cleavage of covalent bonds leads to the formation of neutral species possessing an unpaired electron. These are known as free radicals. Free radicals containing odd electrons on carbon atoms are collectively called carbon radicals or simply free radicals. For example, methyl radical (CH), phenyl radical (Ph), etc. They are classified as primary, secondary, and tertiary free radicals according to the number of carbon atoms (one, two or three) directly attached to the carbon atom bearing the unpaired electron. For example, ethyl radical (CH3ĊH2) is a primary, isopropyl radical (Me2ĊH) is a secondary and tertbutyl radical (Me3Ċ) is a tertiary radical. Stability: (i) Hyperconjugation: Free radicals become stabilized by hyperconjugation involving α-H atoms 14 As the number of a-H atoms increases, hyperconjugation becomes more effective and consequently, the radical becomes more stabilized. The relative stability of simple alkyl radicals is found to follow the sequence (most stable) R3Ċ (tertiary) > R2ĊH (secondary)> RĊH2 (primary) > ĊH3 (methyl) (least stable). For example, tert-butyl radical, Me3Ċ (with nine hyperconjugable α-H atom) is more stable than isopropyl radical, Me2ĊH (with six hyperconjugable α-H atom) which in turn is more stable than ethyl radical, MeĊH2 (with only three hyperconjugable α-H atom). The methyl radical, ĊH3 is least stable because the unpaired electron is not at all delocalized. (ii) Resonance: Resonance is a major factor influencing the stability of tree radicals. When the carbon bearing the odd electron is a to a double bond, effective delocalization of the unpaired electron with the π orbital system with consequent stabilization occurs. Allyl and benzyl radicals, for example, are found to be particularly stable because of resonance. (iii) Steric Strain: Another factor that is responsible for the increased stability of tertiary radicals is steric. There occurs considerable relief of steric strain when a sp2 hybridized tertiary radical is formed from an sp3 hybridized precursor and this is because repulsion between the bulky alkyl groups is relieved to a certain extent by an increase in bond angles from 109.5° to about 120°. Thus, the radical is much reluctant to react further, i.e., its stability is enhanced due to steric reason. 15 AROMATICITY 16 Introduction Aromaticity is a chemical property of organic compound , aromatic compound have following charachterisics: ❖ It has high degree of stability ❖ It shows electrophilic substitution reaction rather than electrophilic addition reaction. It means it doesn’t decolourize bromine water solution. ❖ Aromatic compound follows Huckel rule, according to which a cyclic planar conjugated species having (4n+2𝜋) electrons (where n= 0,1,2,3,…..) is aromatic in nature. ❖ There is diamagnetic ring current. ❖ Each carbon must be sp 2- hybridized or sp-hybridized. ❖ It has high degree stability due to filled bonding molecular orbital 17 Cont.,,, 1. High Degree of stability: High Degree of stability is associated with resonance energy. The compound which have more resonance energy is more stable. The compound which have more potential energy is least stable. Aromaticity α Resonance energy (R.E) α Stability α 1 Potential Energy (P.E) 2. Greater the resonance energy higher is the stability of compound. Resonance energyof some of the aromatic system are given below: 18 Cont.,,, ❖ Cyclic, conjugated π bond, planar with uninterrupted cloud of π electrons above and below the plane of the ring. ❖ Each atom in the ring must have an unhybridized p orbital. (The ring atoms are usually sp2 hybridized or occasionally sp hybridized.) ❖ The Chemist Erich Huckel was the first one to recognize that an aromatic compound must have an odd number of pairs of π electrons in cyclic structure (1, 3, 5, 7), which can mathematically be written as 4n+2 (n = 0,1,2,3 etc.). ❖ Delocalization of the pi electrons over the ring must lower the electronic energy and increases the stability. ❖ Aromatic compounds will have all occupied bonding molecular orbitals completely filled and the relative energies of p molecul ar orbitals in planar cyclic conjugated systems can be determined by a simplified approach developed by A. A. frost in 1953 (FRO ST CIRCLE). ❖ Aromatic systems exhibit a diamagnetic ring current, which causes protons on the outside of the ring to be shifted downfield while any inner protons are shifted upfield (eg-18- annulene), in sharp contrast to a paramagnetic ring current, which causes shifts in the opposite directions. Compounds that sustain a diamagnetic ring current are called diatropic; and are prevalent in 2, 6, 10, 14, 18… electron system. ❖ Commonly it shows electrophilic substitution reaction which is a characteristic of saturated compounds and not electrophilic addition reaction which is characteristic of 19 Types of aromatic compounds (A) 2π- electron system. I. It follow (4n+2)π- electron system. II. If electron is delocalized then compound is aromatic. III. If electron not delocalized then compound is non-aromatic. Compound will never be antiaromatic Examples: 20 (A) 4π- electron system. I. Belongs to 4nπ- electron system doesn’t follow Huckels rule. II. If electron is delocalized then compound is Anti-aromatic. III. If electron does not delocalized then compound is never aromatic. IV. If electron does not delocalized then compound is non-aromatic. 21 (A)6π- electron system. I. Belongs to (4n+2)π- electron system. II ) If electron is delocalized then compound is aromatic III) If electron does not delocalized then compound must be non-aromatic 22 8π- electron system I. Belongs to (4n)π- electron system. II. If electron is delocalized then compound must be Anti-aromatic. III. If electron does not delocalized then compound is non-aromatic 23 Heterocyclic compounds Heterocycle: any cyclic compound that contains ring atom(s) other than carbon (N, O, S, P). Cyclic compounds that contain only carbon are called carbocycles. Pyridine: pi-electron structure resembles benzene (6 pi-electrons) The nitrogen lone pair electrons are not part of the aromatic system. Pyrrole is aromatic but when nitrogen atom of pyrrole is protonated, it becomes non-aromatic. 24 25 26 27 28 29 Anti-Aromaticity Cyclic, containing some number of conjugated π bond, planar with uninterrupted cloud of π electrons above and below the plane of the ring. Each atom in the ring must have an unhybridized p orbital. (The ring atoms are usually sp2 hybridized or occasionally sp hybridized.) Delocalization of the pi electrons over the ring increases the electronic energy and decreases the stability. 4. According to Huckel Antiaromatic compound must have an even number of pairs of π electrons in cyclic structure ( 2, 4, 6, 8 ) which can mathematically be written as 4n (n = 1,2,3 etc.). Antiaromatic systems exhibit a paramagnetic ring current, which causes protons on the outside of the ring to be shifted upfield while any inner protons are shifted downfield (eg-12-annulene), in sharp contrast to a diamagnetic ring current, which causes shifts in the opposite directions. Compounds that sustain a paramagnetic ring current are called paratropic; and are prevalent in 4, 8, 12, 16, 20… electron system 30 Tropylium ion 31 Pentalene 32 Cyclopentadienyl anion 33 Stability Order. Aromatic > Homoaromatic > Nonaromatic > Antiaromatic. Energy Order. Antiaromatic > Nonaromatic > Homoaromatic > Aromatic 34 Questions ? 35 36 When Is A Molecule Aromatic? For a molecule to be aromatic it must: Be cyclic Have a p-orbital on every atom in ring Be planar Posses 4n+2 p electrons (n = any integer) benzene naphthalene Erich Hückel + -Annulene cyclopropenyl cation 37 In general : heterocyclic is the largest and most varied family of organic compounds, heterocyclic system can be 3, 4, 5, 6, 7 membered rings in addition to a fused rings (two rings joined at two adjacent atoms) 38 Heterocyclic derivatives and classification Heterocyclic derivatives as a group, can be divided into two broad areas: aromatic and non-aromatic. (note : aromatic system must have 4n+2 π-electron) 1) the 1, five-membered rings are shown below, the derivative furan 1 is aromatic, while tetrahydrofuran (2), dihydrofuran-2-one (3) are not aromatic, Why?. 2) The six-membered rings below, pyridine is aromatic (5), while piperidine (6), piperidin-2-one (7) are not aromatic, why? Most heterocycles have the same chemistry as their open-chain counterparts particularly when the ring is unsaturated. 39 Six Membered Heterocycles: Pyridine See full size image Piperidine-3D-balls N N H pyridine piperidine Pyridine replaces the CH of benzene by a N atom (and a pair of electrons) Hybridization = sp2 with similar resonance stabilization energy Lone pair of electrons not involved in aromaticity Pyridinium ion: pKa = 5.5 1H NMR: Piperidine: pKa = 11.29 diethylamine : pKa = 10.28 H 7.5 H H 7.1 Pyridine is a weak base Pyridine is -electron deficient H N H 8.5 Electrophilic aromatic substitution is difficult Nucleophilic aromatic substitution is easy pyridine 40 Synthesis of Dyes using simple organic strategy – one hour Dyes and pigments, natural dyes and synthetic dyes – slides 98, a few contents of slides 99, 100 and 101, how doe dye get its colour and specific chromophore – azo and benzenoid and quininoid structures – slides 102, 104 (30 minutes) Synthesis of methyl orange - 113, 115 (15 minutes) Alizarin : slides 122 and 128 (15 minutes) Synthesis of Drugs using simple organic strategy – one hour Aspirin : slide 137, 138 and 139 - (20 minutes) Paracetamol : slides 140, 141 and 142 (20 minutes) Pantaprazole : slides 155, 156 and 157 ( if we have sufficient time, otherwise we will not include) – (20 minutes) 41 42 43 44 45 46 47 48 49 50 51 52 53 54 55 56 57 58 59 60 61 62 63 64 65 66 Aspirin STRUCTURE PROPERTIES Acetylsalicylic Acid is colourless to white crystalline solid It has a smell similar to that of vinegar, because of the hydrolysis yielding salicylic and acetic acid of Acetylsalicylic Acid It is bitter in taste, and density is 1.4 g/cc The melting point of aspirin is 135 deg C, & decomposes at higher temperature Acetyl Salicylic Acid soluble in water, ethyl ether, ethanol, and chloroform. 67 Synthetic route of Aspirin The synthesis of aspirin is an esterification reaction. Salicylic acid is treated with acetic anhydride, an acid derivative, causing a chemical reaction that turns OH group of salicylic acid into anester group (R-OH → R- OCOCH3). This process yields aspirin and acetic acid. The catalyst used in this reaction is sulphuric acid or phosphoric acid. Mechanism of Aspirin Synthesis 68 Application of Aspirin In the year of 1897, Bayer laboratory gave Acetylsalicylic Acid the name of Aspirin. It is a very popular medicine and is available all over the world in large quantities. Ever since the naming, the commercialization of it began. This medicine is most commonly used as an anti-inflammatory and antipyretic. However, considering its use in recent decades, it has also gained a reputation for treating cardiovascular diseases. The other uses also include rheumatic fever and Kawasaki disease. Similarly, we also use it as an intermediate and raw material in producing other medicines or chemical compounds like 4- hydroxycoumarin. Safety Hazards When kept at room temperature. Aspirin will remain stable. However, try to keep it dry so as to avoid its hydrolysis. If you continue using it for a long period of time, it can result in causing gastritis and ulceration. Similarly, it is also incompatible with tough oxidizing agents and strong acids and bases. 69 PARACETAMOL PROPERTIES Density 1.263 g/cm3 Melting point 169 °C (336 °F) 12.78 g/kg (20 °C) ~14 mg/ml (20 °C) Solubility in water 70 Synthetic route of Paracetamol 71 Application of Paracetamol Paracetamol is a common painkiller (analgesic) used to treat aches and pain. It can also be used to reduce a high temperature (antipyretic). but it has no useful anti-inflammatory properties. It's available combined with other painkillers and anti-sickness medicines. It's also an ingredient in a wide range of cold and flu remedies. Paracetamol's effects are thought to be related to inhibition of prostaglandin synthesis. Paracetamol is readily absorbed from the gastrointestinal tract. Safety Hazards Adverse effects of Paracetamol are rare but hypersensitivity including skin rash may occur. There have been reports of blood dyscrasias including thrombocytopenia, neutropenia, pancytopenia, leukopenia and agranulocytosis but these were not necessarily causality related to Paracetamol Very rare cases of serious skin reactions have been reported. 72