Module 2 Reading Skeletal System PDF
Document Details
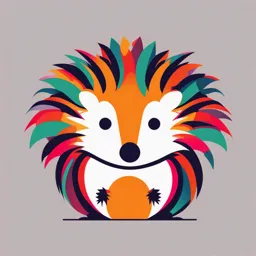
Uploaded by InvinciblePedalSteelGuitar
null
Dr. Thomas Moore and Dr. Roberta Rutledge
Tags
Summary
This document is an educational module about the skeletal system, detailing functions, structures, and features of bones, joints, and connective tissues. It includes a case study on brittle bone disease and questions for the reader to answer.
Full Transcript
6 C H A P T E R Skeletal System: bones and Joints LEARn TO PREdICT dr. Thomas moore and dr. Roberta Rutledge had worked together for almost two decades, and Roberta knew something was bothering Thomas. She noticed him wincing in pain whenever he bent down to retrieve something from a bottom shelf, a...
6 C H A P T E R Skeletal System: bones and Joints LEARn TO PREdICT dr. Thomas moore and dr. Roberta Rutledge had worked together for almost two decades, and Roberta knew something was bothering Thomas. She noticed him wincing in pain whenever he bent down to retrieve something from a bottom shelf, and he was shorttempered rather than his usual happy self. Roberta knew Thomas would never admit to being injured if it meant he couldn’t care for his patients, even if only for a few days. Finally, Roberta convinced him to let her x-ray his lower back. Right away, when Roberta showed Thomas the x-ray, he pointed to the cause of the pain he’d been suffering. using knowledge of the vertebral column, predict the source of Thomas’s pain, based on his x-ray shown in this photo. Also, using your knowledge of vertebral anatomy, predict the region of the injury and explain why this region of the vertebral column is more prone to this type of injury than other regions. doctors examine an x-ray of the lumbar region of the spinal column to diagnose Thomas's ailment. 6.1 FunCTIOnS OF THE SkELETAL SySTEm Learning Outcome Module 5 Skeletal System After reading this section, you should be able to A. Explain the functions of the skeletal system. Sitting, standing, walking, picking up a pencil, and taking a breath all involve the skeletal system. Without the skeletal system, there would be no rigid framework to support the soft tissues of the body and no system of joints and levers to allow the body to move. The skeletal system consists of bones, such as those shown in figure 6.1, as well as their associated connective tissues, which include cartilage, tendons, and ligaments. The term skeleton is derived from a Greek word meaning dried. But the skeleton is far from being dry and nonliving. Rather, the skeletal system consists of dynamic, living tissues that are able to grow, detect pain stimuli, adapt to stress, and undergo repair after injury. A joint, or an articulation, is a place where two bones come together. Many joints are movable, although some of them allow only limited movement; others allow no apparent movement. The structure of a given joint is directly correlated to its degree of movement. Although the skeleton is usually thought of as the framework of the body, the skeletal system has many other functions in addition to support. The major functions of the skeletal system include 1. Support. Rigid, strong bone is well suited for bearing weight and is the major supporting tissue of the body. Cartilage provides firm yet flexible support within certain structures, such as the nose, external ear, thoracic cage, and trachea. Ligaments are strong bands of fibrous connective tissue that attach to bones and hold them together. 2. Protection. Bone is hard and protects the organs it surrounds. For example, the skull encloses and protects the brain, and the vertebrae surround the spinal cord. The rib cage protects the heart, lungs, and other organs of the thorax. 110 Universal Free E-Book Store Skeletal System: Bones and Joints Skull Sternum Ribs Humerus Vertebral column Pelvis Radius Ulna Femur Tibia Fibula Figure 6.1 Major Bones of the Skeletal System molecules, as well as water and minerals. But the types and quantities of these substances differ in each type of connective tissue. Collagen (kol′ lă-jen; koila, glue + -gen, producing) is a tough, ropelike protein. Proteoglycans (prō′ tē-ō-gl ı̄ ′ kanz; proteo, protein + glycan, polysaccharide) are large molecules consisting of polysaccharides attached to core proteins, similar to the way needles of a pine tree are attached to the tree’s branches. The proteoglycans form large aggregates, much as pine branches combine to form a whole tree. Proteoglycans can attract and retain large amounts of water between their polysaccharide “needles.” The extracellular matrix of tendons and ligaments contains large amounts of collagen fibers, making these structures very tough, like ropes or cables. The extracellular matrix of cartilage (kar′ ti-lij) contains collagen and proteoglycans. Collagen makes cartilage tough, whereas the water-filled proteoglycans make it smooth and resilient. As a result, cartilage is relatively rigid, but it springs back to its original shape after being bent or slightly compressed. It is an excellent shock absorber. The extracellular matrix of bone contains collagen and minerals, including calcium and phosphate. The ropelike collagen fibers, like the reinforcing steel bars in concrete, lend flexible strength to the bone. The mineral component, like the concrete itself, gives the bone compression (weight-bearing) strength. Most of the mineral in bone is in the form of calcium phosphate crystals called hydroxyapatite (hı̄ -drok′ sē-ap-ă-t ı̄ t). Skeletal Clavicle 111 Predict 2 What would a bone be like if all of the minerals were removed? What would it be like if all of the collagen were removed? 3. Movement. Skeletal muscles attach to bones by tendons, which are strong bands of connective tissue. Contraction of the skeletal muscles moves the bones, producing body movements. Joints, where two or more bones come together, allow movement between bones. Smooth cartilage covers the ends of bones within some joints, allowing the bones to move freely. Ligaments allow some movement between bones but prevent excessive movement. 4. Storage. Some minerals in the blood—principally, calcium and phosphorus—are stored in bone. Should blood levels of these minerals decrease, the minerals are released from bone into the blood. Adipose tissue is also stored within bone cavities. If needed, the lipids are released into the blood and used by other tissues as a source of energy. 5. Blood cell production. Many bones contain cavities filled with red bone marrow, which produces blood cells and platelets (see chapter 11). 6.2 ExTRACELLuLAR mATRIx Learning Outcome After reading this section, you should be able to A. describe the components of the extracellular matrix, and explain the function of each. The bone, cartilage, tendons, and ligaments of the skeletal system are all connective tissues. Their characteristics are largely determined by the composition of their extracellular matrix. The matrix always contains collagen, ground substance, and other organic A CASE In POInT Brittle Bone Disease may Trix is a 10-year-old girl who has a history of numerous broken bones. At first, physicians suspected she was a victim of child abuse, but eventually they determined that she has brittle bone disease, or osteogenesis imperfecta, which literally means imperfect bone formation. may is short for her age, and her limbs are short and bowed. Her vertebral column is also abnormally curved. Brittle bone disease is a rare disorder caused by any one of a number of faulty genes that results in either too little collagen formation or poor quality collagen. As a result, the bone matrix has decreased flexibility and is more easily broken than normal bone. 6.3 GEnERAL FEATuRES OF BOnE Learning Outcomes After reading this section, you should be able to A. Explain the structural differences between compact bone and spongy bone. B. Outline the processes of bone ossification, growth, remodeling, and repair. There are four categories of bone, based on their shape: long, short, flat, and irregular. Long bones are longer than they are wide. Most of the bones of the upper and lower limbs are long bones. Short bones are approximately as wide as they are long; examples are the bones of the wrist and ankle. Flat bones have a relatively thin, Universal Free E-Book Store Skeletal 112 Chapter 6 flattened shape. Examples of flat bones are certain skull bones, the ribs, the scapulae (shoulder blades), and the sternum. Irregular bones include the vertebrae and facial bones, which have shapes that do not fit readily into the other three categories. Structure of a Long Bone A long bone serves as a useful model for illustrating the parts of a typical bone (figure 6.2). Each long bone consists of a central shaft, called the diaphysis (d ı̄ -af′ i-sis; growing between), and two Articular cartilage Epiphysis Epiphyseal plates in juveniles Epiphyseal lines in adults Spongy bone Compact bone Medullary cavity (contains red marrow in juveniles and yellow marrow in adults) Diaphysis Diaphysis Periosteum Endosteum Young bone (a) (b) (a) Adult bone (b) Osteons (haversian systems) Endosteum Inner layer Periosteum Outer layer Compact bone Central canals Spongy bone with trabeculae Connecting vessels Medullary cavity (c) Adult bone (c) Figure 6.2 Structure of a Long Bone (a) young long bone (the femur) showing the epiphysis, epiphyseal plates, and diaphysis. (b) Adult long bone with epiphyseal lines. (c) Internal features of a portion of the diaphysis in (a). Universal Free E-Book Store 113 Skeletal System: Bones and Joints Osteon Histology of Bone The periosteum and endosteum contain osteoblasts (os′ tē-ōblasts; bone-forming cells), which function in the formation of bone, as well as in the repair and remodeling of bone. When osteoblasts become surrounded by matrix, they are referred to as osteocytes (os′ tē-ō-sı̄ tz; bone cells). Osteoclasts (os′ tē-ō-klastz; bone-eating cells) are also present and contribute to bone repair and remodeling by removing existing bone. Bone is formed in thin sheets of extracellular matrix called lamellae (lă-mel′ ē; plates), with osteocytes located between the lamellae within spaces called lacunae (lă-koo′ nē; a hollows) (figure 6.3). Cell processes extend from the osteocytes across the extracellular matrix of the lamellae within tiny canals called canaliculi (kan-ă-lik′ ū-lı̄ ; sing. canaliculus, little canal). Bone tissue found throughout the skeleton is divided into two major types, based on the histological structure. Compact bone is mostly solid matrix and cells. Spongy bone, or cancellous (kan′ sĕ-lŭs) bone, consists of a lacy network of bone with many small, marrow-filled spaces. Skeletal ends, each called an epiphysis (e-pif′ i-sis; growing upon). A thin layer of articular (ar-tik′ ū-lăr; joint) cartilage covers the ends of the epiphyses where the bone articulates (joins) with other bones. A long bone that is still growing has an epiphyseal plate, or growth plate, composed of cartilage, between each epiphysis and the diaphysis (figure 6.2a). The epiphyseal plate is where the bone grows in length. When bone growth stops, the cartilage of each epiphyseal plate is replaced by bone and becomes an epiphyseal line (figure 6.2b). Bones contain cavities, such as the large medullary cavity in the diaphysis, as well as smaller cavities in the epiphyses of long bones and in the interior of other bones. These spaces are filled with soft tissue called marrow. Yellow marrow consists mostly of adipose tissue. Red marrow consists of blood-forming cells and is the only site of blood formation in adults (see chapter 11). Children’s bones have proportionately more red marrow than do adult bones because, as a person ages, red marrow is mostly replaced by yellow marrow. In adults, red marrow is confined to the bones in the central axis of the body and in the most proximal epiphyses of the limbs. Most of the outer surface of bone is covered by dense connective tissue called the periosteum (per-ē-os′ tē-ŭm; peri, around + osteon, bone), which consists of two layers and contains blood vessels and nerves (figure 6.2c). The surface of the medullary cavity is lined with a thinner connective tissue membrane, the endosteum (en-dos′ tē-ŭm; endo, inside). Compact Bone Compact bone (figure 6.3) forms most of the diaphysis of a long bone and the thinner surfaces of all other bones. As you can see in figure 6.3, compact bone has a predictable pattern of repeating units. These units are called osteons (os′ tē-onz). Each osteon consists of concentric rings of lamellae surrounding a central canal, or Haversian (ha-ver′ shan) canal. As described earlier, osteocytes are located in lacunae between the lamellae of each osteon. Blood vessels that run parallel to the long axis of the bone are located in Concentric rings of lamellae Central canal Osteon Lamellae on surface of bone Lamellae between osteons Periosteum Blood vessel within the periosteum Blood vessels connecting to a central canal Blood vessels within a central (Haversian) canal Canaliculi Osteocytes in lacunae LM 400x (a) Figure 6.3 Canaliculi Lacunae (b) Blood vessel connecting to a central canal between osteons Structure of Bone Tissue (a) Photomicrograph of compact bone. (b) Fine structure of compact bone. Universal Free E-Book Store Skeletal 114 Chapter 6 the central canals. Osteocytes are connected to one another by cell processes in canaliculi. The canaliculi give the osteon the appearance of having tiny cracks within the lamellae. Nutrients leave the blood vessels of the central canals and diffuse to the osteocytes through the canaliculi. Waste products diffuse in the opposite direction. The blood vessels in the central canals, in turn, are connected to blood vessels in the periosteum and endosteum. Spongy Bone Spongy bone, so called because of its appearance, is located mainly in the epiphyses of long bones. It forms the interior of all other bones. Spongy bone consists of delicate interconnecting rods or plates of bone called trabeculae (tră-bek′ū-lē; beams), which resemble the beams or scaffolding of a building (figure 6.4a). Like scaffolding, the trabeculae add strength to a bone without the added weight that would be present if the bone were solid mineralized matrix. The spaces between the trabeculae are filled with marrow. Each trabecula consists of several lamellae with osteocytes between them (figure 6.4b). Usually, no blood vessels penetrate the trabeculae, and the trabeculae have no central canals. Nutrients exit vessels in the marrow and pass by diffusion through canaliculi to the osteocytes of the trabeculae. Bone Ossification Ossification (os′ i-fi-kā′ shŭn; os, bone + facio, to make) is the formation of bone by osteoblasts. After an osteoblast becomes completely surrounded by bone matrix, it becomes a mature bone cell, or osteocyte. In the fetus, bones develop by two processes, each involving the formation of bone matrix on preexisting connective tissue (figure 6.5). Bone formation that occurs within connective tissue membranes is called intramembranous ossification, and bone formation that occurs inside cartilage is called endochondral ossification. Both types of bone formation result in compact and spongy bone. Parietal bone Frontal bone Ossification center Ossification center Superior part of occipital bone Ethmoid bone Inferior part of occipital bone Maxilla Nasal bone Zygomatic bone Temporal bone Mandible Vertebrae Compact bone (a) Spongy bone Styloid process Sphenoid bone Fontanel Intramembranous bones forming Spaces containing bone marrow and blood vessels Trabeculae (a) Cartilage Osteoblast Osteoclast Endochondral bones forming Osteocyte Trabecula (b) Canaliculus Lamellae (b) Figure 6.4 Spongy Bone (a) Beams of bone, the trabeculae, surround spaces in the bone. In life, the spaces are filled with red or yellow bone marrow and with blood vessels. (b) Transverse section of a trabecula. Figure 6.5 Bone Formation in a Fetus (a) Intramembranous ossification occurs in a 12-week-old fetus at ossification centers in the flat bones of the skull (yellow). Endochondral ossification occurs in the bones forming the inferior part of the skull (blue). (b) Radiograph of an 18-week-old fetus, showing intramembranous and endochondral ossification. Intramembranous ossification occurs at ossification centers in the flat bones of the skull. Endochondral ossification has formed bones in the diaphyses of long bones. The epiphyses are still cartilage at this stage of development. Universal Free E-Book Store 115 Skeletal System: Bones and Joints increase in number, enlarge, and die. Then the cartilage matrix becomes calcified (figure 6.6, step 2). As this process is occurring in the center of the cartilage model, blood vessels accumulate in the perichondrium. The presence of blood vessels in the outer surface of future bone causes some of the unspecified connective tissue cells on the surface to become osteoblasts. These osteoblasts then produce a collar of bone around part of the outer surface of the diaphysis, and the perichondrium becomes periosteum in that area. Blood vessels also grow into the center of the diaphyses, bringing in osteoblasts and stimulating ossification. The center part of the diaphysis, where bone first begins to appear, is called the primary ossification center (figure 6.6, step 3). Osteoblasts invade spaces in the center of the bone left by the dying cartilage cells. Some of the calcified cartilage matrix is removed by osteoclasts, and the osteoblasts line up on the remaining calcified matrix and begin to form bone trabeculae. As the bone develops, it Epiphysis Perichondrium Skeletal Intramembranous (in′ tră-mem′ brā-nŭs) ossification occurs when osteoblasts begin to produce bone in connective tissue membranes. This occurs primarily in the bones of the skull. Osteoblasts line up on the surface of connective tissue fibers and begin depositing bone matrix to form trabeculae. The process begins in areas called ossification centers (figure 6.5a), and the trabeculae radiate out from the centers. Usually, two or more ossification centers exist in each flat skull bone, and the skull bones result from fusion of these centers as they enlarge. The trabeculae are constantly remodeled after their initial formation, and they may enlarge or be replaced by compact bone. The bones at the base of the skull and most of the remaining skeletal system develop through the process of endochondral ossification from cartilage models. The cartilage models have the general shape of the mature bone (figure 6.6, step 1). During endochondral ossification, cartilage cells, called chondrocytes, Uncalcified cartilage Perichondrium Calcified cartilage Diaphysis Cartilage Periosteum Bone collar Blood vessel to periosteum Epiphysis 1 A cartilage model, with the general shape of the mature bone, is produced by chondrocytes. A perichondrium surrounds most of the cartilage model. 2 The chondrocytes enlarge, and cartilage is calcified. A 2. bone collar is produced, and the perichondrium of the diaphysis becomes the periosteum. Cartilage Perichondrium Calcified cartilage Primary ossification center Periosteum Bone collar Blood vessel Trabecula Medullary cavity Secondary ossification center Spongy bone Space in bone Cartilage Blood vessel Calcified cartilage Spongy bone Periosteum Bone collar Blood vessel Medullary cavity 3. 3 A primary ossification center forms as blood vessels and osteoblasts invade the calcified cartilage. The osteoblasts lay down bone matrix, forming trabeculae. 4 Secondary ossification centers form in the epiphyses 4. of long bones. PROCESS Figure 6.6 Endochondral Ossification of a Long Bone Universal Free E-Book Store Skeletal 116 Chapter 6 is constantly changing. A medullary cavity forms in the center of the diaphysis as osteoclasts remove bone and calcified cartilage, which are replaced by bone marrow. Later, secondary ossification centers form in the epiphyses (figure 6.6, step 4). enlarge and die. The cartilage matrix becomes calcified. Much of the cartilage that forms around the enlarged cells is removed by osteoclasts, and the dying chondrocytes are replaced by osteoblasts. The osteoblasts start forming bone by depositing bone lamellae on the surface of the calcified cartilage. This process produces bone on the diaphyseal side of the epiphyseal plate. Bone Growth Bone growth occurs by the deposition of new bone lamellae onto existing bone or other connective tissue. As osteoblasts deposit new bone matrix on the surface of bones between the periosteum and the existing bone matrix, the bone increases in width, or diameter. This process is called appositional growth. Growth in the length of a bone, which is the major source of increased height in an individual, occurs in the epiphyseal plate. This type of bone growth occurs through endochondral ossification (figure 6.7). Chondrocytes increase in number on the epiphyseal side of the epiphyseal plate. They line up in columns parallel to the long axis of the bone, causing the bone to elongate. Then the chondrocytes Predict 3 Describe the appearance of an adult if cartilage growth did not occur in the long bones during childhood. Bone Remodeling Bone remodeling involves the removal of existing bone by osteoclasts and the deposition of new bone by osteoblasts. Bone remodeling occurs in all bone. Remodeling is responsible for changes in bone shape, the adjustment of bone to stress, bone repair, and calcium ion regulation in the body fluids. Remodeling is also involved in Femur Patella Epiphysis Epiphyseal plate Diaphysis (a) Length of bone increases. 1 Epiphyseal plate Chondrocytes divide and enlarge. Thickness of epiphyseal plate remains unchanged. 2 3 Calcified cartilage is replaced by bone. Bone of diaphysis (b) 4 Bone is added to diaphysis. Epiphyseal side 1 New cartilage is produced on the epiphyseal side of the plate as the chondrocytes divide and form stacks of cells. 1 2 Chondrocytes mature and enlarge. 2 3 Matrix is calcified, and chondrocytes die. 3 4 The cartilage on the diaphyseal side of the plate is replaced by bone. 4 LM 400x (c) Diaphyseal side PROCESS Figure 6.7 Endochondral Bone Growth (a) Location of the epiphyseal plate in a long bone. (b) As the chondrocytes of the epiphyseal plate divide and align in columns, the cartilage expands toward the epiphysis, and the bone elongates. At the same time, the older cartilage is calcified and then replaced by bone, which is remodeled, resulting in expansion of the medullary cavity of the diaphysis. The net result is an epiphyseal plate that remains uniform in thickness through time but is constantly moving toward the epiphysis, resulting in elongation of the bone. (c) Photomicrograph of an epiphyseal plate, demonstrating chondrocyte division and enlargement and the areas of calcification and ossification. Universal Free E-Book Store Skeletal System: Bones and Joints Bone Repair Sometimes a bone is broken and needs to be repaired. When this occurs, blood vessels in the bone are also damaged. The vessels bleed, and a clot (hematoma) forms in the damaged area (figure 6.8, step 1). Two to three days after the injury, blood vessels and cells from surrounding tissues begin to invade the clot. Some of these cells produce a fibrous network of connective tissue between the broken bones, which holds the bone fragments together and fills the gap between them. Other cells produce islets of cartilage in the fibrous network. The network of fibers and islets of cartilage between the two bone fragments is called a callus (figure 6.8, step 2). Osteoblasts enter the callus and begin forming spongy bone (figure 6.8, step 3). Spongy bone formation in the callus is usually complete 4–6 weeks after the injury. Immobilization of the bone is critical up to this time because movement can refracture the delicate new matrix. Subsequently, the spongy bone is slowly remodeled to form compact and spongy bone, and the repair is complete (figure 6.8, step 4). Although immobilization at a fracture point is critical during the early stages of bone healing, complete immobilization is not good for the bone, the muscles, or the joints. Not long ago, it was common practice to immobilize a bone completely for as long as 10 weeks. But we now know that, if a bone is immobilized for as little as 2 weeks, the muscles associated with that bone may lose as much as half their strength. Furthermore, if a bone is completely immobilized, it is not subjected to the normal mechanical stresses that help it form. Bone matrix is reabsorbed, and the strength of the bone decreases. In experimental animals, complete immobilization of the back for 1 month resulted in up to a threefold decrease in vertebral compression strength. Modern therapy attempts to balance bone immobilization with enough exercise to keep muscle and bone from decreasing in size and strength and to maintain joint mobility. These goals are accomplished by limiting the amount of time a cast is left on the patient and by using “walking casts,” which allow some stress on the bone and some movement. Total healing of the fracture may require several months. If a bone heals properly, the healed region can be even stronger than the adjacent bone. Skeletal bone growth when newly formed spongy bone in the epiphyseal plate forms compact bone. A long bone increases in length and diameter as new bone is deposited on the outer surface and growth occurs at the epiphyseal plate. At the same time, bone is removed from the inner, medullary surface of the bone. As the bone diameter increases, the thickness of the compact bone relative to the medullary cavity tends to remain fairly constant. If the size of the medullary cavity did not also increase as bone size increased, the compact bone of the diaphysis would become thick and very heavy. Because bone is the major storage site for calcium in the body, bone remodeling is important to maintain blood calcium levels within normal limits. Calcium is removed from bones when blood calcium levels decrease, and it is deposited when dietary calcium is adequate. This removal and deposition is under hormonal control (see “Bone and Calcium Homeostasis” in the next section). If too much bone is deposited, the bones become thick or develop abnormal spurs or lumps that can interfere with normal function. Too little bone formation or too much bone removal, as occurs in osteoporosis, weakens the bones and makes them susceptible to fracture (see Systems Pathology, “Osteoporosis”). 117 6.4 Bone and Calcium Homeostasis Learning Outcomes After reading this section, you should be able to A. Explain the role of bone in calcium homeostasis. B. Describe how parathyroid hormone and calcitonin influence bone health and calcium homeostasis. Bone is the major storage site for calcium in the body, and movement of calcium into and out of bone helps determine blood calcium levels, which is critical for normal muscle and nervous system function. Calcium (Ca2+) moves into bone as osteoblasts build new bone and out of bone as osteoclasts break down bone (figure 6.9). When osteoblast and osteoclast activity is balanced, the movements of calcium into and out of a bone are equal. Compact bone Medullary cavity Periosteum Woven bone External callus: Woven bone Cartilage Hematoma Broken humerus Dead bone Internal callus: Dead bone Fibers and cartilage Compact bone at break site Woven bone Hematoma formation Callus around broken humerus (at arrow) (a) 1 Blood released from damaged blood vessels forms a hematoma. (b) Callus formation 2 The internal callus forms between the ends of the bones, and the external callus forms a collar around the break. Callus ossification 3 Woven, spongy bone replaces the internal and external calluses. Bone remodeling 4 Compact bone replaces woven bone, and part of the internal callus is removed, restoring the medullary cavity. PROCESS Figure 6.8 Bone Repair Universal Free E-Book Store 118 Chapter 6 Skeletal CLINICAL IMPACT Bone fractures can be classified as open (or compound), if the bone protrudes through the skin, and closed (or simple), if the skin is not perforated. Figure 6A illustrates some of the different types of fractures. If the fracture totally separates the two bone fragments, it is called complete; if it doesn’t, it is called incomplete. An incomplete fracture that occurs on the convex side of the curve of a bone is called a greenstick fracture. A comminuted (kom′i-nū-ted; broken into small pieces) fracture is one in which the bone breaks into more than two fragments. An impacted fracture occurs when one of the fragments of one part of the bone is driven into the spongy bone of another fragment. Bone Fractures Fractures can also be classified according to the direction of the fracture line as linear (parallel to the long axis); Comminuted Linear transverse (at right angles to the long axis); or oblique or spiral (at an angle other than a right angle to the long axis). Impacted Spiral Incomplete Complete Oblique Transverse Figure 6A Types of bone fractures. Decreased blood Ca2+ 1 5 Increased blood Ca2+ Posterior aspect of thyroid gland Kidney Parathyroid glands 1 Decreased blood Ca2+ stimulates PTH secretion from parathyroid glands. Thyroid gland 2 PTH stimulates osteoclasts to break down bone and release Ca2+ into the blood. 3 In the kidneys, PTH increases Ca2+ reabsorption from the urine. PTH also stimulates active vitamin D formation. 3 PTH Calcitonin 2 6 Stimulates osteoclasts Vitamin D Inhibits osteoclasts Bone 4 Osteoclasts promote Ca2+ uptake from bone. 4 Vitamin D promotes Ca2+ absorption from the small intestine into the blood. 5 Increased blood Ca2+ stimulates calcitonin secretion from the thyroid gland. 6 Calcitonin inhibits osteoclasts, which allows for enhanced osteoblast uptake of Ca2+ from the blood to deposit into bone. Ca2+ Osteoblasts promote Ca2+ deposition in bone. Small intestine Ca2+ Blood PROCESS Figure 6.9 Calcium Homeostasis Universal Free E-Book Store 119 Skeletal System: Bones and Joints 1. PTH indirectly stimulates osteoclasts to break down bone, which releases stored calcium into the blood. 2. PTH stimulates the kidney to take up calcium from the urine and return it to the blood. 3. PTH stimulates the formation of active vitamin D, which, in turn, promotes increased calcium absorption from the small intestine. PTH and vitamin D, therefore, cause blood calcium levels to increase, maintaining homeostatic levels. Decreasing blood calcium levels stimulate PTH secretion. Table 6.1 Number Axial Skeleton Parietal Temporal Frontal Occipital Sphenoid Ethmoid Face Paired Maxilla Zygomatic Palatine Nasal Lacrimal Inferior nasal concha Unpaired Mandible Vomer 6.5 General Considerations of Bone Anatomy Learning Outcome After reading this section, you should be able to A. List and define the major features of a typical bone. It is traditional to list 206 bones in the average adult skeleton (table 6.1), although the actual number varies from person to person and decreases with age as some bones fuse. Anatomists use several common terms to describe the features of bones (table 6.2). For example, a hole in a bone is called a foramen (fō-r ā′ men; pl. foramina, fō-r ā′ min-ă; foro, to pierce). A foramen usually exists in a bone because some structure, such as a nerve or blood vessel, passes through the bone at that point. If Named Bones in the Adult Human Skeleton Bones Skull Braincase Paired Unpaired Calcitonin works to decrease blood calcium levels by inhibiting osteoclast activity. Even in the absence of osteoclast activity, osteoblast activity continues, removing calcium from the blood and depositing it into the bone. Thus, calcitonin maintains homeostatic blood calcium levels by decreasing calcium levels that are too high. In summary, PTH, vitamin D, and calcitonin work together to keep blood calcium levels within the homeostatic range and are described more fully in chapter 10. Skeletal When blood calcium levels are too low, osteoclast activity increases, osteoclasts release calcium from bone into the blood, and blood calcium levels increase. Conversely, if blood calcium levels are too high, osteoclast activity decreases, osteoblasts remove calcium from the blood to produce new bone, and blood calcium levels decrease. Calcium homeostasis is maintained by three hormones: parathyroid hormone (PTH) from the parathyroid glands, vitamin D from the skin or diet, and calcitonin (kal-si-tō′ nin) from the thyroid gland. PTH and vitamin D are secreted when blood calcium levels are too low and calcitonin is secreted when blood calcium levels are too high. PTH works through three simultaneous mechanisms to increase blood calcium levels. total skull 2 2 1 1 1 1 2 2 2 2 2 2 1 1 22 Auditory Ossicles Malleus Incus Stapes 2 2 2 6 total Hyoid 1 Bones Number Thoracic Cage Ribs Sternum (3 parts, sometimes considered 3 bones) 24 1 total thoracic cage 25 total axial skeleton 80 Appendicular Skeleton Pectoral Girdle Scapula Clavicle 2 2 Upper Limb Humerus Ulna Radius Carpal bones Metacarpal bones Phalanges 2 2 2 16 10 28 64 total girdle and upper limb Pelvic Girdle Coxal bone 2 Lower Limb Femur Tibia Fibula Patella Tarsal bones Metatarsal bones Phalanges 2 2 2 2 14 10 28 Vertebral Column Cervical vertebrae Thoracic vertebrae Lumbar vertebrae Sacrum Coccyx 7 12 5 1 1 total girdle and lower limb 62 total appendicular skeleton 126 26 total bones 206 total vertebral column Universal Free E-Book Store 120 Chapter 6 Skeletal Table 6.2 Anatomical Terms for Features of Bones Term Description Major Features Body, shaft Main portion Head Enlarged (often rounded) end Neck Constricted area between head and body Condyle Smooth, rounded articular surface Facet Small, flattened articular surface Crest Prominent ridge Process Prominent projection Tubercle, or tuberosity Knob or enlargement Trochanter Large tuberosity found only on proximal femur Epicondyle Enlargement near or above a condyle Openings or Depressions Foramen Hole Canal, meatus Tunnel Fissure Cleft Sinus Cavity Fossa Depression the hole is elongated into a tunnel-like passage through the bone, it is called a canal or a meatus (mē-ā′ tus; a passage). A depression in a bone is called a fossa (fos′ ă). A lump on a bone is called a tubercle (too′ ber-kl; a knob) or a tuberosity (too′ ber-os′ i-tē), and a projection from a bone is called a process. Most tubercles and processes are sites of muscle attachment on the bone. Increased muscle pull, as occurs when a person lifts weights to build up muscle mass, can increase the size of some tubercles. The smooth, rounded end of a bone, where it forms a joint with another bone, is called a condyle (kon′ d ı̄ l; knuckle). The bones of the skeleton are divided into axial and appendicular portions (figure 6.10). 6.6 Axial Skeleton Learning Outcomes After reading this section, you should be able to A. Name the bones of the skull and describe their main features as seen from the lateral, frontal, internal, and inferior views. B. List the bones that form the majority of the nasal septum. C. Describe the locations and functions of the paranasal sinuses. D. List the bones of the braincase and the face. E. Describe the shape of the vertebral column, and list its divisions. F. Discuss the common features of the vertebrae and contrast vertebrae from each region of the vertebral column. G. List the bones and cartilage of the rib cage, including the three types of ribs. The axial skeleton is composed of the skull, the vertebral column, and the thoracic cage. Skull The 22 bones of the skull are divided into those of the braincase and those of the face (see table 6.1). The braincase, which encloses the cranial cavity, consists of 8 bones that immediately surround and protect the brain; 14 facial bones form the structure of the face. Thirteen of the facial bones are rather solidly connected to form the bulk of the face. The mandible, however, forms a freely movable joint with the rest of the skull. There are also three auditory ossicles (os′ i-klz) in each middle ear (six total). Many students studying anatomy never see the individual bones of the skull. Even if they do, it makes more sense from a functional, or clinical, perspective to study most of the bones as they appear together in the intact skull because many of the anatomical features of the skull cannot be fully appreciated by examining the separate bones. For example, several ridges on the skull cross more than one bone, and several foramina are located between bones rather than within a single bone. For these reasons, it is more relevant to think of the skull, excluding the mandible, as a single unit. The major features of the intact skull are therefore described from four views. Lateral View The parietal bones (pă-r ı̄ ′ĕ-tăl; wall) and temporal (tem′pō-răl) bones form a large portion of the side of the head (figure 6.11). (The word temporal refers to time, and the temporal bone is so named because the hairs of the temples turn white, indicating the passage of time.) These two bones join each other on the side of the head at the squamous (skwā′mŭs) suture. A suture is a joint uniting bones of the skull. Anteriorly, the parietal bone is joined to the frontal (forehead) bone by the coronal (kōr′ō-năl; corona, crown) suture, and posteriorly it is joined to the occipital (ok-sip′i-tăl; back of the head) bone by the lambdoid (lam′doyd) suture. A prominent feature of the temporal bone is a large opening, the external auditory canal, a canal that enables sound waves to reach the eardrum. The mastoid (mas′toyd) process of the temporal bone can be seen and felt as a prominent lump just posterior to the ear. Important neck muscles involved in rotation of the head attach to the mastoid process. Part of the sphenoid (sfē′ noyd) bone can be seen immediately anterior to the temporal bone. Although it appears to be two small, paired bones on the sides of the skull, the sphenoid bone is actually a single bone that extends completely across the skull. It resembles a butterfly, with its body in the center of the skull and its wings extending to the sides of the skull. Anterior to the sphenoid bone is the zygomatic (zı̄ -gō-mat′ ik) bone, or cheekbone, which can be easily felt. The zygomatic arch, which consists of joined processes of the temporal and zygomatic bones, forms a bridge across the side of the face and provides a major attachment site for a muscle moving the mandible. The maxilla (mak-sil′ă; jawbone) forms the upper jaw, and the mandible (man′di-bl; jaw) forms the lower jaw. The maxilla articulates by sutures to the temporal bone. The maxilla contains the superior set of teeth, and the mandible contains the inferior set of teeth. Frontal View The major structures seen from the frontal view are the frontal bone, the zygomatic bones, the maxillae, and the mandible (figure 6.12a). The teeth are very prominent in this view. Many bones of the face can be easily felt through the skin (figure 6.12b). Universal Free E-Book Store 121 Skeletal System: Bones and Joints Axial Skeleton Appendicular Skeleton Axial Skeleton Skull Skull Mandible Skeletal Mandible Hyoid bone Clavicle Scapula Sternum Humerus Ribs Ribs Vertebral column Vertebral column Ulna Radius Sacrum Sacrum Carpal bones Metacarpal bones Phalanges Coccyx Coxal bone Femur Patella Tibia Fibula Tarsal bones Metatarsal bones Phalanges Anterior view Figure 6.10 Posterior view Complete Skeleton Bones of the axial skeleton are listed in the far left- and right-hand columns; bones of the appendicular skeleton are listed in the center. (The skeleton is not shown in the anatomical position.) Universal Free E-Book Store 122 Chapter 6 Coronal suture Skeletal Frontal bone Parietal bone Squamous suture Temporal bone Sphenoid bone Nasal bone Occipital bone Lacrimal bone Nasolacrimal canal Lambdoid suture Ethmoid bone Mandibular condyle Zygomatic bone External auditory canal Maxilla Mastoid process Styloid process Zygomatic arch Mental foramen Coronoid process Mandible Lateral view Figure 6.11 Skull as Seen from the Right Side (The names of bones are in bold.) From this view, the most prominent openings into the skull are the orbits (ōr′bitz; eye sockets) and the nasal cavity. The orbits are cone-shaped fossae, so named because the eyes rotate within them. The bones of the orbits provide both protection for the eyes and attachment points for the muscles that move the eyes. The orbit is a good example of why it is valuable to study the skull as an intact structure. No fewer than seven bones come together to form the orbit, and for the most part, the contribution of each bone to the orbit cannot be appreciated when the bones are examined individually. Each orbit has several openings through which structures communicate with other cavities (figure 6.12a). The largest of these are the superior and inferior orbital fissures. They provide openings through which nerves and blood vessels communicate with the orbit or pass to the face. The optic nerve, for the sense of vision, passes from the eye through the optic foramen and enters the cranial cavity. The nasolacrimal (nā-zō-lak′ ri-măl; nasus, nose + lacrima, tear) canal (see figure 6.11) passes from the orbit into the nasal cavity. It contains a duct that carries tears from the eyes to the nasal cavity. A small lacrimal (lak′ ri-măl) bone can be seen in the orbit just above the opening of this canal. Predict 4 Why does your nose run when you cry? The nasal cavity is divided into right and left halves by a nasal septum (sep′ tŭm; wall) (figure 6.12a). The bony part of the nasal septum consists primarily of the vomer (vō′ mer) inferiorly and the perpendicular plate of the ethmoid (eth′ moyd; sieveshaped) bone superiorly. The anterior part of the nasal septum is formed by cartilage. The external part of the nose is formed mostly of cartilage. The bridge of the nose is formed by the nasal bones. Each of the lateral walls of the nasal cavity has three bony shelves, called the nasal conchae (kon′kē; resembling a conch shell). The inferior nasal concha is a separate bone, and the middle and superior conchae are projections from the ethmoid bone. The conchae increase the surface area in the nasal cavity. The increased surface area of the overlying epithelium facilitates moistening and warming of the air inhaled through the nose (see chapter 15). Several of the bones associated with the nasal cavity have large cavities within them, called the paranasal (par-ă-nā′săl; para, alongside) sinuses (figure 6.13), which open into the nasal cavity. The sinuses decrease the weight of the skull and act as resonating chambers during voice production. Compare a normal voice with the voice of a person who has a cold and whose sinuses are “stopped up.” The sinuses are named for the bones where they are located and include the frontal, maxillary, ethmoidal, and sphenoidal sinuses. Universal Free E-Book Store Skeletal System: Bones and Joints 123 Frontal bone Skeletal Supraorbital foramen Coronal suture Optic foramen Parietal bone Superior orbital fissure Sphenoid bone Temporal bone Nasal bone Orbit Lacrimal bone Zygomatic bone Inferior orbital fissure Nasal septum Perpendicular plate of ethmoid bone Infraorbital foramen Vomer Middle nasal concha Inferior nasal concha Maxilla Nasal cavity Mandible Mental foramen Frontal view (a) Frontal bone Zygomatic bone Nasal bone Maxilla Mandible Figure 6.12 Skull and Face (The names of bones are in bold.) (a) Frontal view of the skull. (b) Bony landmarks of the face. The skull has additional sinuses, called the mastoid air cells, which are located inside the mastoid processes of the temporal bone. These air cells open into the middle ear instead of into the nasal cavity. An auditory tube connects the middle ear to the nasopharynx (upper part of throat). Interior of the Cranial Cavity When the floor of the cranial cavity is viewed from above with the roof cut away (figure 6.14), it can be divided roughly into three cranial fossae (anterior, middle, and posterior), which are formed as the developing skull conforms to the shape of the brain. The (b) bones forming the floor of the cranial cavity, from anterior to posterior, are the frontal, ethmoid, sphenoid, temporal, and occipital bones. Several foramina can be seen in the floor of the middle fossa. These allow nerves and blood vessels to pass through the skull. For example, the foramen rotundum and foramen ovale transmit important nerves to the face. A major artery to the meninges (the membranes around the brain) passes through the foramen spinosum. The internal carotid artery passes through the carotid canal, and the internal jugular vein passes through the jugular foramen (see chapter 13). The large foramen magnum, through which the spinal cord joins the brain, is located in the posterior Universal Free E-Book Store 124 Chapter 6 Frontal sinus Skeletal Ethmoidal sinus Sphenoidal sinus Maxillary sinus (a) Lateral view (b) Anterior view Figure 6.13 Paranasal Sinuses fossa. The central region of the sphenoid bone is modified into a structure resembling a saddle, the sella turcica (sel′ ă tŭr′ sı̆-kă; Turkish saddle), which contains the pituitary gland. Base of Skull Viewed from Below Many of the same foramina that are visible in the interior of the skull can also be seen in the base of the skull, when viewed from below, with the mandible removed (figure 6.15). Other specialized structures, such as processes for muscle attachments, can also be seen. The foramen magnum is located in the occipital bone near the center of the skull base. Occipital condyles (ok-sip′ i-tăl kon′ d ı̄ lz), the smooth points of articulation between the skull and the vertebral column, are located beside the foramen magnum. Two long, pointed styloid (st ı̄ ′ loyd; stylus or pen-shaped) processes project from the inferior surface of the temporal bone. The muscles involved in moving the tongue, the hyoid bone, and the pharynx (throat) originate from this process. The mandibular fossa, where the mandible articulates with the temporal bone, is anterior to the mastoid process. The hard palate (pal′ ăt) forms the floor of the nasal cavity and the roof of the mouth. The anterior two-thirds of the hard palate is formed by the maxillae, the posterior one-third by the palatine (pal′ ă-tı̄ n) bones. The connective tissue and muscles that make up the soft palate extend posteriorly from the hard, or bony, palate. The hard and soft palates separate the nasal cavity and nasopharynx from the mouth, enabling us to chew and breathe at the same time. Hyoid Bone The hyoid bone (figure 6.16) is an unpaired, U-shaped bone. It is not part of the skull (see table 6.1) and has no direct bony attachment to the skull. Muscles and ligaments attach it to the skull. The hyoid bone provides an attachment for some tongue muscles, and it is an attachment point for important neck muscles that elevate the larynx (voicebox) during speech or swallowing. Frontal sinuses Frontal bone Anterior cranial fossa Ethmoid bone: Crista galli Cribriform plate Sphenoid bone Foramen rotundum Foramen ovale Optic foramen Foramen spinosum Sella turcica Middle cranial fossa Carotid canal Internal auditory canal Temporal bone Jugular foramen Foramen magnum Hypoglossal canal Parietal bone Occipital bone Posterior cranial fossa (a) Figure 6.14 Superior view (b) Superior view Floor of the Cranial Cavity (The names of bones are in bold.) (a) drawing of the floor of the cranial cavity. The roof of the skull has been removed, and the floor is viewed from above. (b) Photo of the same view. Universal Free E-Book Store 125 Skeletal System: Bones and Joints Incisive fossa Maxilla Skeletal Hard palate: Palatine process of maxillary bone Horizontal plate of palatine bone Inferior orbital fissure Zygomatic bone Sphenoid bone Vomer Styloid process Foramen ovale Mandibular fossa Foramen spinosum Carotid canal External auditory canal Mastoid process Jugular foramen Temporal bone Occipital condyle Occipital bone Foramen magnum Nuchal lines (b) Inferior view (a) Figure 6.15 Inferior view (b) Base of the Skull as Viewed from Below (The names of bones are in bold.) (a) drawing of skull’s inferior with the mandible removed. (b) Photo of the same view. Vertebral Column The vertebral column, or backbone, is the central axis of the skeleton, extending from the base of the skull to slightly past the end of the pelvis. In adults, it usually consists of 26 individual bones, grouped into five regions (figure 6.17; see table 6.1): 7 cervical (ser′ vı̆-kal; neck) vertebrae (ver′ tĕ-brē; verto, to turn), 12 thoracic (thō-ras′ik) vertebrae, 5 lumbar (lŭm′bar) vertebrae, 1 sacral (sā′ krăl) bone, and 1 coccyx (kok′ siks) bone. The adult sacral and coccyx bones fuse from 5 and 3–4 individual bones, respectively. For convenience, each of the five regions is identified by a letter, and the vertebrae within each region are numbered: C1–C7, T1–T12, L1–L5, S, and CO. You can remember the number of vertebrae in each region by remembering meal times: 7, 12, and 5. The adult vertebral column has four major curvatures. The cervical region curves anteriorly, the thoracic region curves posteriorly, the lumbar region curves anteriorly, and the sacral and coccygeal regions together curve posteriorly. Abnormal vertebral curvatures are not uncommon. Kyphosis (kı̄ -fō′ sis) is an abnormal posterior curvature of the spine, mostly in the upper thoracic region, resulting in a hunchback condition. Lordosis (lōr-dō′ sis; curving forward) is an abnormal anterior Body Anterior view Body Lateral view (from the left side) Figure 6.16 Hyoid Bone Universal Free E-Book Store 126 Chapter 6 Skeletal First cervical vertebra (atlas) Cervical region (curved anteriorly) Second cervical vertebra (axis) Seventh cervical vertebra First thoracic vertebra Thoracic region (curved posteriorly) Body Intervertebral disk Twelfth thoracic vertebra First lumbar vertebra Lumbar region (curved anteriorly) Intervertebral foramina Transverse process curvature of the spine, mainly in the lumbar region, resulting in a swayback condition. Scoliosis (skō-lē-ō′ sis) is an abnormal lateral curvature of the spine. The vertebral column performs the following five major functions: (1) supports the weight of the head and trunk; (2) protects the spinal cord; (3) allows spinal nerves to exit the spinal cord; (4) provides a site for muscle attachment; and (5) permits movement of the head and trunk. General Plan of the Vertebrae Each vertebra consists of a body, an arch, and various processes (figure 6.18). The weight-bearing portion of each vertebra is the body. The vertebral bodies are separated by intervertebral disks (see figure 6.17), which are formed by fibrocartilage. The vertebral arch surrounds a large opening called the vertebral foramen. The vertebral foramina of all the vertebrae form the vertebral canal, where the spinal cord is located. The vertebral canal protects the spinal cord from injury. Each vertebral arch consists of two pedicles (ped′ ı̆-klz), which extend from the body to the transverse process of each vertebra, and two laminae (lam′ i-nē; thin plates), which extend from the transverse processes to the spinous process. A transverse process extends laterally from each side of the arch, between the pedicle and lamina, and a single spinous process projects dorsally from where the two laminae meet. The spinous processes can be seen and felt as a series of lumps down the midline of the back (see figure 6.24b). The transverse and spinous processes provide attachment sites for the muscles that move the vertebral column. Spinal nerves exit the spinal cord through the intervertebral foramina, which are formed by notches in the pedicles of adjacent vertebrae (see figure 6.17). Each vertebra has a superior and an inferior articular process where the vertebrae articulate with each other. Each articular process has a smooth “little face” called an articular facet (fas′ et). Spinous process Posterior Fifth lumbar vertebra Sacral promontory Articular facet Spinous process Superior articular process Lamina Transverse process Sacrum Sacral and coccygeal regions (curved posteriorly) Vertebral arch Pedicle Vertebral foramen Body Coccyx Anterior Lateral view Figure 6.17 Vertebral Column Complete column viewed from the left side. Superior view Figure 6.18 Vertebra Universal Free E-Book Store 127 Skeletal System: Bones and Joints Regional Differences in Vertebrae cervical vertebra (figure 6.19b) is called the axis because a considerable amount of rotation occurs at this vertebra, as in shaking the head “no.” This rotation occurs around a process called the dens (denz), which protrudes superiorly from the axis. The thoracic vertebrae (figure 6.19d) possess long, thin spinous processes that are directed inferiorly. The thoracic vertebrae also have extra articular facets on their lateral surfaces that articulate with the ribs. The lumbar vertebrae (figure 6.19e) have large, thick bodies and heavy, rectangular transverse and spinous processes. Because the lumbar vertebrae have massive bodies and carry a large amount of weight, ruptured intervertebral disks are more common in this area than in other regions of the column. The superior Skeletal The cervical vertebrae (figure 6.19a–c) have very small bodies, except for the atlas, which has no body. Because the cervical vertebrae are relatively delicate and have small bodies, dislocations and fractures are more common in this area than in other regions of the vertebral column. Each of the transverse processes has a transverse foramen through which the vertebral arteries pass toward the brain. Several of the cervical vertebrae also have partly split spinous processes. The first cervical vertebra (figure 6.19a) is called the atlas because it holds up the head, as Atlas in classical mythology held up the world. Movement between the atlas and the occipital bone is responsible for a “yes” motion of the head. It also allows a slight tilting of the head from side to side. The second Posterior arch Transverse process Vertebral foramen Superior articular facet (articulates with occipital condyle) Facet for dens (a) Transverse foramen Atlas (first cervical vertebra), superior view Anterior arch Spinous process Lamina Dens Vertebral foramen Transverse process Superior articular facet Facets for rib attachment Pedicle Body Body Dens (articulates with atlas) (b) Axis (second cervical vertebra), superior view Lateral view (d) Thoracic vertebra, superior view Spinous process Lamina Lamina Pedicle Vertebral foramen Transverse foramen Superior articular facet Transverse process (c) Spinous process Transverse process Superior articular facet Pedicle Vertebral foramen Body Body Cervical vertebra, superior view (e) Figure 6.19 Lumbar vertebra, superior view Regional Differences in Vertebrae The posterior portion lies at the top of each illustration. Universal Free E-Book Store Skeletal 128 Chapter 6 articular facets of the lumbar vertebrae face medially, whereas the inferior articular facets face laterally. This arrangement tends to “lock” adjacent lumbar vertebrae together, giving the lumbar part of the vertebral column more strength. The articular facets in other regions of the vertebral column have a more “open” position, allowing for more rotational movement but less stability than in the lumbar region. The five sacral vertebrae are fused into a single bone called the sacrum (figure 6.20). The spinous processes of the first four sacral vertebrae form the median sacral crest. The spinous process of the fifth vertebra does not form, leaving a sacral hiatus (hı̄ -ā-tŭs) at the inferior end of the sacrum, which is often the site of “caudal” anesthetic injections given just before childbirth. The anterior edge of the body of the first sacral vertebra bulges to form the sacral promontory (prom′ on-tō-rē) (see figure 6.17), a landmark that can be felt during a vaginal examination. It is used as a reference point to determine if the pelvic openings are large enough to allow for normal vaginal delivery of a baby. The coccyx, or tailbone, usually consists of four more-orless fused vertebrae. The vertebrae of the coccyx do not have the typical structure of most other vertebrae. They consist of extremely reduced vertebral bodies, without the foramina or processes, usually fused into a single bone. The coccyx is easily broken when a person falls by sitting down hard on a solid surface or in women during childbirth. Rib Cage The rib cage protects the vital organs within the thorax and prevents the collapse of the thorax during respiration. It consists of the thoracic vertebrae, the ribs with their associated cartilages, and the sternum (figure 6.21). A Case in Point Rib Fractures Han D. Mann’s ladder fell as he was working on his roof, and he landed chest-first on the ladder. Three ribs were fractured on his right side. It was difficult for Han to cough, laugh, or even breathe without severe pain in the right side of his chest. The middle ribs are those most commonly fractured, and the portion of each rib that forms the lateral wall of the thorax is the weakest and most commonly broken. The pain from rib fractures occurs because the broken ends move during respiration and other chest movements, stimulating pain receptors. Broken rib ends can damage internal organs, such as the lungs, spleen, liver, and diaphragm. Fractured ribs are not often dislocated, but dislocated ribs may have to be set for proper healing to occur. Binding the chest to limit movement can facilitate healing and lessen pain. Ribs and Costal Cartilages The 12 pairs of ribs can be divided into true ribs and false ribs. The true ribs, ribs 1–7, attach directly to the sternum by means of costal cartilages. The false ribs, ribs 8–12, do not attach directly to the sternum. Ribs 8–10 attach to the sternum by a common cartilage; ribs 11 and 12 do not attach at all to the sternum and are called floating ribs. Sternum The sternum (ster′nŭm), or breastbone (figure 6.21), is divided into three parts: the manubrium (mă-nū′brē-ŭm; handle), the body, and the xiphoid (zif′oyd, zı̄ ′foyd; sword) process. The sternum resembles a sword, with the manubrium forming the handle, the body forming the blade, and the xiphoid process forming the tip. At the Vertebral canal (sacral canal) Articular facet (articulates with fifth lumbar vertebra) Sacral promontory Anterior sacral foramina Median sacral crest Posterior sacral foramina Sacral hiatus Coccyx (a) Anterior view Figure 6.20 Sacrum Coccyx (b) Posterior view Coccyx (c) Posterior view Universal Free E-Book Store 129 Skeletal System: Bones and Joints Seventh cervical vertebra Clavicle First thoracic vertebra Jugular notch 2 Sternal angle True ribs (1–7) Skeletal 1 3 4 Costal cartilage Manubrium 5 Sternum Body 6 Xiphoid process 7 11 8 False ribs (8–12) 9 Floating ribs T12 L1 12 10 Anterior view Figure 6.21 Rib Cage superior end of the sternum, a depression called the jugular notch is located between the ends of the clavicles where they articulate with the sternum. A slight elevation, called the sternal angle, can be felt at the junction of the manubrium and the body of the sternum. This junction is an important landmark because it identifies the location of the second rib. This identification allows the ribs to be counted; for example, it can help a health professional locate the apex of the heart, which is between the fifth and sixth ribs. The xiphoid process is another important landmark of the sternum. During cardiopulmonary resuscitation (CPR), it is very important to place the hands over the body of the sternum rather than over the xiphoid process. Pressure applied to the xiphoid process can drive it into an underlying abdominal organ, such as the liver, causing internal bleeding. 6.7 Appendicular Skeleton Learning Outcomes After reading this section, you should be able to A. Identify the bones that make up the pectoral girdle, and relate their structure and arrangement to the function of the girdle. B. Name and describe the major bones of the upper limb. C. Name and describe the bones of the pelvic girdle and explain why the pelvic girdle is more stable than the pectoral girdle. D. Name the bones that make up the coxal bone. Distinguish between the male and female pelvis. E. Identify and describe the bones of the lower limb. The appendicular (ap′ en-dik′ ū-lăr; appendage) skeleton consists of the bones of the upper and lower limbs, as well as the girdles, which attach the limbs to the axial skeleton. Pectoral Girdle The pectoral (pek′ tō-răl) girdle, or shoulder girdle (figure 6.22), consists of four bones, two scapulae and two clavicles, which attach the upper limb to the body. The scapula (skap′ ū-lă), or shoulder blade, is a flat, triangular bone with three large fossae where muscles extending to the arm are attached (figures 6.23 and 6.24; see figure 6.10). A fourth fossa, the glenoid (glen′ oyd) cavity, is where the head of the humerus connects to the scapula. A ridge, called the spine, runs across the posterior surface of the scapula. A projection, called the acromion (ă-krō′ mē-on; akron, tip + omos, shoulder) process, extends from the scapular spine to form the point of the shoulder. The clavicle (klav′ i-kl), or collarbone, articulates with the scapula at the acromion process. The proximal end of the clavicle is attached to the sternum, providing the only bony attachment of the scapula to the remainder of the skeleton. The clavicle is the first bone to begin ossification in the fetus. This relatively brittle bone may be fractured in the newborn during delivery. The bone remains slender in children and may be broken as a child attempts to take the impact of a fall on an outstretched hand. The clavicle is thicker in adults and is less vulnerable to fracture. Even though it is the first bone to begin ossification, it is the last to complete ossification. The coracoid (kōr′ ă-koyd) process curves below the clavicle and provides for the attachment of arm and chest muscles. Universal Free E-Book Store 130 Chapter 6 Clavicle Pectoral girdle Skeletal Scapula Humerus evidence that a person was engaged in lifting heavy objects during life. If the humerus of a person exhibits an unusually large deltoid tuberosity for her age, it may indicate, in some societies, that she was a slave and was required to lift heavy loads. The distal end of the humerus is modified into specialized condyles that connect the humerus to the forearm bones. Epicondyles (ep′ i-kon′ d ı̄ lz; epi, upon) on the distal end of the humerus, just lateral to the condyles, provide attachment sites for forearm muscles. Forearm Ulna Upper limb Radius Carpal bones Metacarpal bones Phalanges Anterior view Figure 6.22 Bones of the Pectoral Girdle and Right Upper Limb Upper Limb The upper limb consists of the bones of the arm, forearm, wrist, and hand (see figure 6.22). Arm The arm is the region between the shoulder and the elbow; it contains the humerus (hū′ mer-ŭs; shoulder) (figure 6.25). The proximal end of the humerus has a smooth, rounded head, which attaches the humerus to the scapula at the glenoid cavity. Around the edge of the humeral head is the anatomical neck. When the joint needs to be surgically replaced, this neck is not easily accessible. A more accessible site for surgical removal is at the surgical neck, located at the proximal end of the humeral shaft. Lateral to the head are two tubercles, a greater tubercle and a lesser tubercle. Muscles originating on the scapula attach to the greater and lesser tubercles and hold the humerus to the scapula. Approximately one-third of the way down the shaft of the humerus, on the lateral surface, is the deltoid tuberosity, where the deltoid muscle attaches. The size of the deltoid tuberosity can increase as the result of frequent and powerful pulls from the deltoid muscle. For example, in bodybuilders, the deltoid muscle and the deltoid tuberosity enlarge substantially. Anthropologists, examining ancient human remains, can use the presence of enlarged deltoid tuberosities as The forearm has two bones: the ulna (ŭl′ nă) on the medial (little finger) side of the forearm and the radius on the lateral (thumb) side (figure 6.26). The proximal end of the ulna forms a trochlear notch that fits tightly over the end of the humerus, forming most of the elbow joint. Just proximal to the trochlear notch is an extension of the ulna, called the olecranon (ō-lek′ ră-non; elbow) process, which can be felt as the point of the elbow (see figure 6.28). Just distal to the trochlear notch is a coronoid (kōr′ ŏ-noyd) process, which helps complete the “grip” of the ulna on the distal end of the humerus. The distal end of the ulna forms a head, which articulates with the bones of the wrist, and a styloid process is located on its medial side. The ulnar head can be seen as a prominent lump on the posterior ulnar side of the wrist. The proximal end of the radius has a head by which the radius articulates with both the humerus and the ulna. The radius does not attach as firmly to the humerus as the ulna does. The radial head rotates against the humerus and ulna. Just distal to the radial head is a radial tuberosity, where one of the arm muscles, the biceps brachii, attaches. The distal end of the radius articulates with the wrist bones. A styloid process is located on the lateral side of the distal end of the radius. The radial and ulnar styloid processes provide attachment sites for ligaments of the wrist. Wrist The wrist is a relatively short region between the forearm and the hand; it is composed of eight carpal (kar′ păl; wrist) bones (figure 6.27). These eight bones are the scaphoid (skaf′ oyd), lunate (lū′ nāt), triquetrum (tr ı̄ -kwē′ trŭm), pisiform (pis′ i-fōrm), trapezium (tra-pē′ zē-ŭm), trapezoid (trap′ ĕ-zoyd), capitate (kap′ i-tāt), and hamate (ha′ māt). The carpal bones are arranged in two rows of four bones each and form a slight curvature that is concave anteriorly and convex posteriorly. A number of mnemonics have been developed to help students remember the carpal bones. The following one allows students to remember them in order from lateral to medial for the proximal row (top) and from medial to lateral (by the thumb) for the distal row: So Long Top Part, Here Comes The Thumb—that is, Scaphoid, Lunate, Triquetrum, Pisiform, Hamate, Capitate, Trapezoid, and Trapezium. Hand Five metacarpal (met′ ă-kar′ păl) bones are attached to the carpal bones and form the bony framework of the hand (figure 6.27). The metacarpal bones are aligned with the five digits: the thumb and fingers. They are numbered 1 to 5, from the thumb to the little finger. The ends, or heads, of the five metacarpal bones associated with the thumb and fingers form the knuckles (figure 6.28). Each finger consists of three small bones called phalanges (fă-lan′ jēz; Universal Free E-Book Store Skeletal System: Bones and Joints CLINICAL IMPACT Carpal Tunnel Syndrome also cause the tendons in the carpal tunnel to enlarge. The accumulated fluid and enlarged tendons can apply pressure to a major nerve passing through the tunnel. The pressure on this nerve causes carpal tunnel syndrome, characterized by tingling, burning, and numbness in the hand. Treatments for carpal tunnel syndrome vary, depending on the severity of the syndrome. mild cases can be treated nonsurgically with either anti-inflammatory medications or stretching exercises. However, if symptoms have lasted for more than 6 months, surgery is recommended. Surgical techniques involve cutting the carpal ligament to enlarge the carpal tunnel and ease pressure on the nerve. Acromion process Skeletal The bones and ligaments on the anterior side of the wrist form a carpal tunnel, which does not have much “give.” Tendons and nerves pass from the forearm through the carpal tunnel to the hand. Fluid and connective tissue can accumulate in the carpal tunnel as a result of inflammation associated with overuse or trauma. The inflammation can 131 Acromion process Coracoid process Coracoid process Glenoid cavity Supraspinous fossa Glenoid cavity Spine Subscapular fossa Lateral border Infraspinous fossa Medial border View in (d) Lateral border Inferior angle (a) Anterior view (b) Spine of scapula Posterior view Posterior Supraspinous fossa of scapula Body of clavicle Acromion process of scapula Proximal end Distal end of clavicle Coracoid process of scapula Distal end Body of clavicle (c) Figure 6.23 Superior view (d) Anterior Superior view Right Scapula and Clavicle (a) Right scapula, anterior view. (b) Right scapula, posterior view. (c) Right clavicle, superior view. (d) Photograph of the right scapula and clavicle from a superior view, showing the relationship between the distal end of the clavicle and the acromion process of the scapula. Universal Free E-Book Store 132 Chapter 6 Spinous process of seventh cervical vertebra Skeletal Distal end of clavicle Superior border of scapula Jugular notch Acromion process Spine of scapula Clavicle Scapula Sternum Medial border of scapula Inferior angle of scapula Lumbar spinous processes (a) (b) (b) Figure 6.24 Surface Anatomy of the Pectoral Girdle and Thoracic Cage (a) Bones of the pectoral girdle and the anterior thorax. (b) Bones of the scapula and the posterior vertebral column. Head Greater tubercle Greater tubercle Anatomical neck Lesser tubercle Surgical neck Deltoid tuberosity Olecranon fossa Lateral epicondyle Lateral epicondyle Medial epicondyle Capitulum Condyles Trochlea (a) Trochlea Anterior view Posterior view (b) Anterior view Figure 6.25 Right Humerus Universal Free E-Book Store 133 Skeletal System: Bones and Joints Olecranon process Radius Ulna Trochlear notch Carpal bones (proximal row) Coronoid process Radial tuberosity Ulna (shaft) Radius (shaft) Scaphoid Lunate Triquetrum Pisiform Metacarpal bones Hamate Capitate Trapezoid Trapezium Carpal bones (distal row) Skeletal Head 1 5 4 3 2 Proximal phalanx of thumb Distal phalanx of thumb Proximal phalanx of finger Digits Styloid process (a) Anterior view Head Styloid process Middle phalanx of finger (b) Anterior view Distal phalanx of finger Posterior view Olecranon process Trochlear notch Head of radius (c) Figure 6.26 Superior view Figure 6.27 Bones of the Right Wrist and Hand Coronoid process Right Ulna and Radius (a) Anterior view of the right ulna and radius. (b) Photo of the same view of the right ulna and radius. (c) Proximal ends of the right ulna and radius. sing. phalanx, fā′ langks), named after the Greek phalanx, a wedge of soldiers holding their spears, tips outward, in front of them. The phalanges of each finger are called proximal, middle, and distal, according to their position in the digit. The thumb has two phalanges, proximal and distal. The digits are also numbered 1 to 5, starting from the thumb. Pelvic Girdle The pelvic girdle is the place where the lower limbs attach to the body (figure 6.29). The right and left coxal (kok′sul) bones, or hip bones, join each other anteriorly and the sacrum posteriorly to form a ring of bone called the pelvic girdle. The pelvis (pel′vis; basin) includes the pelvic girdle and the coccyx (figure 6.30). The sacrum and coccyx form part of the pelvis but are also part of the axial skeleton. Each coxal bone is formed by three bones fused to one another to form a single bone (figure 6.31). The ilium (il′ē-ŭm) is the most superior, the ischium (is′kē-ŭm) is inferior and posterior, and the pubis (pū′bis) is inferior and anterior. An iliac crest can be seen Heads of metacarpal bones (knuckles) Acromion process Medial border of scapula Head of ulna Olecranon process Olecranon process Figure 6.28 Surface Anatomy Showing Bones of the Pectoral Girdle and Upper Limb along the superior margin of each ilium, and an anterior superior iliac spine, an important hip landmark, is located at the anterior end of the iliac crest. The coxal bones join each other anteriorly at the pubic (pū′bik) symphysis and join the sacrum posteriorly at the sacroiliac (sā-krō-il′ē-ak) joints (see figure 6.30). The acetabulum (as-ĕ-tab′ū-lŭm; vinegar cup) is the socket of the hip joint. The obturator (ob′too-r ā-tŏr) foramen is the large hole in each coxal bone that is closed off by muscles and other structures. Universal Free E-Book Store 134 Chapter 6 Sacrum Coxal bone Pelvic girdle during childbirth. The pelvic inlet is formed by the pelvic brim and the sacral promontory. The pelvic outlet is bounded by the ischial spines, the pubic symphysis, and the coccyx (figure 6.32c). Skeletal Lower Limb The lower limb consists of the bones of the thigh, leg, ankle, and foot (see figure 6.29). Thigh Femur The thigh is the region between the hip and the knee (figure 6.33a). It contains a single bone called the femur. The head of the femur articulates with the acetabulum of the coxal bone. At the distal end of the femur, the condyles articulate with the tibia. Epicondyles, located medial and lateral to the condyles, are points of ligament attachment. The femur can be distinguished from the humerus by its long neck, located between the head and the trochanters Patella Tibia Lower limb Fibula Tarsal bones Metatarsal bones Phalanges Anterior view Figure 6.29 Bones of the Pelvic Girdle and Right Lower Limb The male pelvis can be distinguished from the female pelvis because it is usually larger and more massive, but the female pelvis tends to be broader (figure 6.32; table 6.3). Both the inlet and the outlet of the female pelvis are larger than those of the male pelvis, and the subpubic angle is greater in the female (figure 6.32a,b). The increased size of these openings helps accommodate the fetus Table 6.3 Differences Between Male and Female Pelvic Girdles Area Description of Difference General Female pelvis somewhat lighter in weight and wider laterally but shorter superiorly to inferiorly and less funnel-shaped; less obvious muscle attachment points in female than in male Sacrum Broader in female, with the inferior portion directed more posteriorly; the sacral promontory projects less anteriorly in female Pelvic inlet Heart-shaped in male; oval in female Pelvic outlet Broader and more shallow in female Subpubic angle Less than 90 degrees in male; 90 degrees or more in female Ilium More shallow and flared laterally in female Ischial spines Farther apart in female Ischial tuberosities Turned laterally in female and medially in male (not shown in figure 6.32) Sacroiliac joint Iliac crest Sacrum Sacral promontory Ilium Anterior superior iliac spine Coccyx Pubis Acetabulum Coxal bone Pubic symphysis Ischium Obturator foramen Subpubic angle Anterosuperior view Figure 6.30 Pelvis Universal Free E-Book Store Skeletal System: Bones and Joints 135 Articular surface (area of articulation with sacrum) Iliac crest Ilium Skeletal Iliac fossa Pelvic brim Greater sciatic notch Acetabulum Greater sciatic notch Pubis Ischial spine Ischial spine Ischium Pubic symphysis Ischial tuberosity Obturator foramen Lateral view (a) Figure 6.31 Pelvic inlet (red dashed line) Ischium (b) Medial view (c) Lateral view Right Coxal Bone Sacral promontory Ischial spine Pelvic brim Coccyx Pubic symphysis Pubic symphysis Pelvic outlet (blue dashed line) Subpubic angle Male Female (a) Anterosuperior view (b) Anterosuperior view Sacral promontory Figure 6.32 Comparison of the Male Pelvis to the Female Pelvis (a) In a male, the pelvic inlet (red dashed line) and outlet (blue dashed line) are small, and the subpubic angle is less than 90 degrees. (b) In a female, the pelvic inlet (red dashed line) and outlet (blue dashed line) are larger, and the subpubic angle is 90 degrees or greater. (c) A midsagittal section through the pelvis shows the pelvic inlet (red arrow and red dashed line) and the pelvic outlet (blue arrow and blue dashed line). Pelvic brim Pelvic inlet Coccyx Pelvic outlet (c) Medial view Universal Free E-Book Store 136 Chapter 6 Head Head Greater trochanter Greater trochanter Neck Skeletal Neck Lesser trochanter Linea aspera Body (shaft) of femur Medial epicondyle Lateral epicondyle Intercondylar fossa Lateral epicondyle Patellar groove (a) Lateral condyle Medial condyle Anterior view Posterior view (b) Anterior view Anterior surface Anterior view (c) Figure 6.33 Bones of the Thigh (a) Right femur. (b) Photo of anterior right femur. (c) Patella. (trō′ kan-terz). A “broken hip” is usually a break of the femoral neck. A broken hip is difficult to repair and often requires pinning to hold the femoral head to the shaft. A major complication can occur if the blood vessels between the femoral head and the acetabulum are damaged. If this occurs, the femoral head may degenerate from lack of nourishment. The trochanters are points of muscle attachment. The patella (pa-tel′ ă), or kneecap (figure 6.33c), is located within the major tendon of the anterior thigh muscles and enables the tendon to bend over the knee. Leg The leg is the region between the knee and the ankle (figure 6.34). It contains two bones, called the tibia (tib′ ē-ă; shinbone) and the fibula (fib′ ū-lă). The tibia is the larger of the two and is the major weight-bearing bone of the leg. The rounded condyles of the femur rest on the flat condyles on the proximal end of the tibia. Just distal to the condyles of the tibia, on its anterior surface, is the tibial tuberosity, where the muscles of the anterior thigh attach. The fibula does not articulate with the femur, but its head is attached to the proximal end of the tibia. The distal ends of the tibia and fibula form a partial socket that articulates with a bone of the ankle (the talus). A prominence can be seen on each side of the ankle (figure 6.34). These are the medial malleolus (mal-ē′ ō-lŭs) of the tibia and the lateral malleolus of the fibula. Ankle The ankle consists of seven tarsal (tar′ săl; the sole of the foot) bones (figure 6.35). The tarsal bones are the talus (tā′ lŭs; ankle bone), calcaneus (kal-kā′ nē-ŭs; heel), cuboid (kū′ boyd), and navicular (nă-vik′ yū-lăr), and the medial, intermediate, and lateral cuneiforms (kū′ nē-i-fōrmz). The talus articulates with the tibia and fibula to form the ankle joint, and the calcaneus forms the heel (figure 6.36). A mnemonic for the distal row is MILC—that is, Medial, Intermediate, and Lateral cuneiforms and the Cuboid. A mnemonic for the proximal three bones is No Thanks Cow—that is, Navicular, Talus, and Calcaneus. Universal Free E-Book Store 137 Skeletal System: Bones and Joints Medial condyle Head Tibial tuberosity Fibula Foot The metatarsal (met′ ă-tar′ săl) bones and phalanges of the foot are arranged and numbered in a manner very similar to the metacarpal bones and phalanges of the hand (see figure 6.35). The metatarsal bones are somewhat longer than the metacarpal bones, whereas the phalanges of the foot are considerably shorter than those of the hand. There are three primary arches in the foot, formed by the positions of the tarsal bones and metatarsal bones, and held in place by ligaments. Two longitudinal arches extend from the heel to the ball of the foot, and a transverse arch extends across the foot. The arches function similarly to the springs of a car, allowing the foot to give and spring back. Tibia Skeletal Lateral condyle 6.8 Joints Learning Outcomes Medial malleolus Lateral malleolus Anterior view (a) Figure 6.34 (b) Anterior view Bones of the Leg The right tibia and fibula are shown. After reading this section, you should be able to A. Describe the two systems for classifying joints. B. Explain the structure of a fibrous joint, list the three types, and give examples of each type. C. Give examples of cartilaginous joints. D. Illustrate the structure of a synovial joint and explain the roles of the components of a synovial joint. E. Classify synovial joints based on the shape of the bones in the joint and give an example of each type. F. Demonstrate the difference between the following pairs of movements: flexion and extension; plantar flexion and dorsiflexion; abduction and adduction; supination and pronation; elevation and depression; protraction and retraction; opposition and reposition; inversion and eversion. Calcaneus Talus Cuboid Tarsal bones Navicular Medial cuneiform Fibula Intermediate cuneiform Metatarsal bones Tibia Lateral cuneiform 5 Navicular 4 3 2 1 Talus Proximal phalanx Digits Middle phalanx Distal phalanx Proximal phalanx of great toe Distal phalanx of great toe (a) Figure 6.35 Superior view Calcaneus Cuboid Phalanges (b) Metatarsal bones Tarsal bones Medial view Bones of the Right Foot Universal Free E-Book Store 138 Chapter 6 be reinforced by additional collagen fibers. This type of cartilage, called fibrocartilage (see chapter 4), forms joints such as the intervertebral disks. Skeletal Medial epicondyle of femur Head of fibula Patella Tibial tuberosity Calcaneus Anterior crest of tibia Lateral epicondyle of femur Medial malleolus Lateral malleolus Synovial Joints Synovial (si-nō′ vē-ăl) joints are freely movable joints that contain fluid in a cavity surrounding the ends of articulating bones. Most joints that unite the bones of the appendicular skeleton are synovial joints, whereas many of the joints that unite the bones of the axial skeleton are not. This pattern reflects the greater mobility of the appendicular skeleton compared to that of the axial skeleton. Parietal bone Figure 6.36 Surface Anatomy Showing Bones of the Lower Limb A joint, or an articulation, is a place where two bones come together. A joint is usually considered movable, but that is not always the case. Many joints exhibit limited movement, and others are completely, or almost completely, immovable. One method of classifying joints is a functional classification. Based on the degree of motion, a joint may be called a synarthrosis (sin′ ar-thrō′ sis; nonmovable joint), an amphiarthrosis (am′ fi-ar-thrō′ sis; slightly movable joint), or a diarthrosis (d ı̄ -arthrō′ sis; freely movable joint). However, functional classification is somewhat restrictive and is not used in this text. Instead, we use a structural classification whereby joints are classified according to the type of connective tissue that binds the bones together and whether there is a fluid-filled joint capsule. The three major structural classes of joints are fibrous, cartilaginous, and synovial. Fibrous Joints Fibrous joints consist of two bones that are united by fibrous tissue and that exhibit little or no movement. Joints in this group are further subdivided on the basis of structure as sutures, syndesmoses, or gomphoses. Sutures (soo′ choorz) are fibrous joints between the bones of the skull (see figure 6.11). In a newborn, some parts of the sutures are quite wide and are called fontanels (fon′ tă-nelz′ ), or soft spots (figure 6.37). They allow flexibility in the skull during the birth process, as well as growth of the head after birth. Syndesmoses (sin′ dez-mō′ sēz) are fibrous joints in which the bones are separated by some distance and held together by ligaments. An example is the fibrous membrane connecting most of the distal parts of the radius and ulna. Gomphoses (gom-fō′ sēz) consist of pegs fitted into sockets and held in place by ligaments. The joint between a tooth and its socket is a gomphosis. Cartilaginous Joints Cartilaginous joints unite two bones by means of cartilage. Only slight movement can occur at these joints. Examples are the cartilage in the epiphyseal plates of growing long bones and the cartilages between the ribs and the sternum. The cartilage of some cartilaginous joints, where much strain is placed on the joint, may Frontal bone Squamous suture Coronal suture Occipital bone Lambdoid suture Mastoid (posterolateral) fontanel Sphenoidal (anterolateral) fontanel Temporal bone (a) Lateral view Frontal bones (not yet fused into a single bone) Frontal (anterior) fontanel Parietal bone Occipital bone (b) Sagittal suture Occipital (posterior) fontanel Superior view Figure 6.37 Fetal Skull Showing Fontanels and Sutures Universal Free E-Book Store Skeletal System: Bones and Joints Types of Synovial Joints Synovial joints are classified according to the shape of the adjoining articular surfaces (figure 6.39). Plane joints, or gliding joints, consist of two opposed flat surfaces that glide over each other. Examples of these joints are the articular facets between vertebrae. Saddle joints consist of two saddle-shaped articulating surfaces oriented at right angles to each other. Movement in these joints can occur in two planes. The joint between the metacarpal bone and the carpal bone (trapezium) of the thumb is a saddle joint. Hinge joints permit movement in one plane only. They consist of a convex cylinder of one bone applied to a corresponding concavity of the other bone. Examples are the elbow and knee joints (figure 6.40a,b). The flat condylar surface of the knee joint is modified into a concave surface by shock-absorbing fibrocartilage pads called menisci (mĕ-nis′ s ı̄ ). Pivot joints restrict movement to rotation around a single axis. Each pivot joint consists of a cylindrical bony process that rotates within a ring composed partly of bone and partly of ligament. The rotation that occurs between the axis and atlas when shaking the head “no” is an example. The articulation between the proximal ends of the ulna and radius is also a pivot joint. Ball-and-socket joints consist of a ball (head) at the end of one bone and a socket in an adjacent bone into which a portion of the ball fits. This type of joint allows a wide range of movement in almost any direction. Examples are the shoulder and hip joints (figure 6.40c,d). Ellipsoid (ē-lip′ soyd) joints, or condyloid (kon′ di-loyd) joints, are elongated ball-and-socket joints. The shape of the joint limits its range of movement nearly to that of a hinge motion, but in two planes. Examples of ellipsoid joints are the joint between the occipital condyles of the skull and the atlas of the vertebral column and the joints between the metacarpal bones and phalanges. Skeletal Several features of synovial joints are important to their function (figure 6.38). The articular surfaces of bones within synovial joints are covered with a thin layer of articular cartilage, which provides a smooth surface where the bones meet. The joint cavity is filled with fluid. The cavity is enclosed by a joint capsule, which helps hold the bones together and allows for movement. Portions of the fibrous part of the joint capsule may be thickened to form ligaments. In addition, ligaments and tendons outside the joint capsule contribute to the strength of the joint. A synovial membrane lines the joint cavity everywhere except over the articular cartilage. The membrane produces synovial fluid, which is a complex mixture of polysaccharides, proteins, lipids, and cells. Synovial fluid forms a thin, lubricating film covering the surfaces of the joint. In certain synovial joints, the synovial membrane may extend as a pocket, or sac, called a bursa (ber′ să; pocket). Bursae are located between structures that rub together, such as where a tendon crosses a bone; they reduce friction, which could damage the structures involved. Inflammation of a bursa, often resulting from abrasion, is called bursitis. A synovial membrane may extend as a tendon sheath along some tendons associated with joints (figure 6.38). 139 Bone Synovial membrane Blood vessel Nerve Fibrous part of joint capsule Joint capsule Bursa Joint cavity (filled with synovial fluid) Articular cartilage Tendon sheath Tendon Bone Figure 6.38 Outer layer Inner layer Periosteum Structure of a Synovial Joint Universal Free E-Book Store 140 Chapter 6 Skeletal Class and Example of Joint Plane Acromioclavicular Carpometacarpal Costovertebral Intercarpal Intermetatarsal Intertarsal Intervertebral Plane Intervertebral Sacroiliac Tarsometatarsal Saddle Carpometacarpal pollicis Intercarpal Sternoclavicular Saddle Hinge Pivot Slight Slight Slight Slight Slight Slight Slight Slight Slight Two axes Hinge Cubital (elbow) Knee Interphalangeal Talocrural (ankle) Humerus, ulna, and radius Femur and tibia Between phalanges Talus, tibia, and fibula One axis One axis One axis Multiple axes; one predominates Pivot Atlantoaxial Proximal radioulnar Distal radioulnar Atlas and axis Radius and ulna Radius and ulna Rotation Rotation Rotation Coxal bone and femur Scapula and humerus Multiple axes Multiple axes Atlas and occipital bone Metacarpal bones and phalanges Metatarsal bones and phalanges Radius and carpal bones Mandible and temporal bone Two axes Two axes Slight Slight Carpometacarpal Cubital Proximal radioulnar Glenohumeral Ellipsoid Atlantooccipital Metacarpophalangeal (knuckles) Metatarsophalangeal (ball of foot) Radiocarpal (wrist) Temporomandibular Ellipsoid Acromion process of scapula and clavicle Carpals and metacarpals 2–5 Ribs and vertebrae Between carpal bones Between metatarsal bones Between tarsal bones Between articular processes of adjacent vertebrae Between sacrum and coxal bone (complex joint with several planes and synchondroses) Tarsal bones and metatarsal bones Movement Carpal and metacarpal of thumb Between carpal bones Manubrium of sternum and clavicle Ball-and-Socket Coxal (hip) Glenohumeral (shoulder) Ball-and-socket Structures Joined Atlantooccipital Two axes Multiple axes Multiple axes; one predominates Figure 6.39 Types of Synovial Joints Universal Free E-Book Store 141 Skeletal System: Bones and Joints Humerus Joint capsule Femur Synovial membrane Fat pad Joint cavity Patella Olecranon bursa Articular cartilage Coronoid process Elbow Articular cartilage of the trochlear notch (a) Fat pad Patellar ligament Deep infrapatellar bursa Knee Articular cartilage Meniscus Trochlea Ulna Tibia Sagittal section Acromion process (articular surface) Subacromial bursa Joint cavity (b) Sagittal section Pelvic bone Articular cartilage over head of humerus Articular cartilage over glenoid cavity Tendon sheath on tendon of long head of biceps brachii Scapula (cut surface) Biceps brachii tendon Articular cartilage Joint cavity Ligamentum teres Joint capsule Head of femur Greater trochanter Neck of femur Shoulder Joint capsule Humerus Hip Lesser trochanter Biceps brachii muscle (c) Skeletal Quadriceps femoris tendon Suprapatellar bursa Subcutaneous prepatellar bursa Femur Frontal section (d) Frontal section Figure 6.40 Examples of Synovial Joints (a) Elbow. (b) Knee. (c) Shoulder. (d) Hip. Types of Movement The types of movement occurring at a given joint are related to the structure of that joint. Some joints are limited to only one type of movement, whereas others permit movement in several directions. All the movements are described relative to the anatomical position. Because most movements are accompanied by movements in the opposite direction, they are often illustrated in pairs (figure 6.41). Flexion and extension are common opposing movements. The literal definitions are to bend (flex) and to straighten (extend). Flexion occurs when the bones of a particular joint are moved closer together, whereas extension occurs when the bones of a particular joint are moved farther apart, such that the bones are now arranged somewhat end-to-end (figure 6.41a,b). An example of flexion occurs when a person flexes the forearm to “make a muscle.” There are special cases of flexion when describing movement of the foot. Movement of the foot toward the plantar surface (sole of the foot), as when standing on the toes, is commonly called plantar flexion. Movement of the foot toward the shin, as when walking on the heels, is called dorsiflexion. Universal Free E-Book Store 142 Chapter 6 Skeletal Abduction Posterior to frontal plane Anterior to frontal plane Flexion Abduction Extension Adduction Flexion Extension (a) (b) (c) Frontal plane Pronation (d) Supination Circumduction Medial rotation (e) Figure 6.41 Lateral rotation (f) Types of Movement (a) Flexion and extension of the elbow. (b) Flexion and extension of the neck. (c) Abduction and adduction of the fingers. (d) Pronation and supination of the hand. (e) Medial and lateral rotation of the arm. (f ) Circumduction of the arm. Universal Free E-Book Store Skeletal System: Bones and Joints A Case in Point Dislocated Shoulder Abduction (ab-dŭk′ shun; to take away) is movement away from the median or midsagittal plane; adduction (to bring together) is movement toward the median plane (figure 6.41c). Moving the legs away from the midline of the body, as in the outward movement of “jumping jacks,” is abduction, and bringing the legs back together is adduction. Pronation (prō-nā′ shŭn) and supination (soo′ pi-nā′ shun) are best demonstrated with the elbow flexed at a 90-degree angle. When the elbow is flexed, pronation is rotation of the forearm so that the palm is down, and supination is rotation of the forearm so that the palm faces up (figure 6.41d). Eversion (ē-ver′ zhŭn) is turning the foot so that the plantar surface (bottom of the foot) faces laterally; inversion (in-ver′ zhŭn) is turning the foot so that the plantar surface faces medially. Rotation is the turning of a structure around its long axis, as in shaking the head “no.” Rotation of the arm can best be demonstrated with the elbow flexed (figure 6.41e) so that rotation is not confused with supination and pronation of the forearm. With the elbow flexed, medial rotation of the arm brings the forearm against the anterior surface of the abdomen, and lateral rotation moves it away from the body. Circumduction (ser-kŭm-dŭk′shŭn) occurs at freely movable joints, such as the shoulder. In circumduction, the arm moves so that it traces a cone where the shoulder joint is at the cone’s apex (figure 6.41f ). In addition to the movements pictured in figure 6.41, several other movement types have been identified: Protraction (prō-trak′ shŭn) is a movement in which a structure, such as the mandible, glides anteriorly. In retraction (rē-trak′ shŭn), the structure glides posteriorly. Elevation is movement of a structure in a superior direction. Closing the mouth involves elevation of the mandible. Depression is movement of a structure in an inferior direction. Opening the mouth involves depression of the mandible. Excursion is movement of a structure to one side, as in moving the mandible from side to side. Opposition is a movement unique to the thumb and little finger. It occurs when the tips of the thumb and little finger are brought toward each other across the palm of the hand. The thumb can also oppose the other digits. Reposition returns the digits to the anatomical position. Most movements that occur in the course of normal activities are combinations of movements. A complex movement can be described by naming the individual movements involved. When the bones of a joint are forcefully pulled apart and the ligaments around the joint are pulled or torn, a sprain results. A separation exists when the bones remain apart after injury to a joint. A dislocation is when the end of one bone is pulled out of the socket in a ball-and-socket, ellipsoid, or pivot joint. Hyperextension is usually defined as an abnormal, forced extension of a joint beyond its normal range of motion. For example, if a person falls and attempts to break the fall by putting out a hand, the force of the fall directed into the hand and wrist may cause hyperextension of the wrist, which may result in sprained joints or broken bones. Some health professionals, however, define hyperextension as the normal movement of a structure into the space posterior to the anatomical position. Skeletal The shoulder joint is the most commonly dislocated joint in the body. Loosh Holder dislocated his shoulder joint while playing basketball. As a result of a “charging” foul, Loosh was knocked backward and fell. As he broke his fall with his extended right arm, the head of the right humerus was forced out of the glenoid cavity. While being helped up from the floor, Loosh felt severe pain in his shoulder, his right arm sagged, and he could not move his arm at the shoulder. Most dislocations result in stretching of the joint capsule and movement of the humeral head to the inferior, anterior side of the glenoid cavity. The dislocated humeral head is moved back to its normal position by carefully pulling it laterally over the inferior lip of the glenoid cavity and then superiorly into the glenoid cavity. Once the shoulder joint capsule has been stretched by a shoulder dislocation, the shoulder joint may be predisposed to future dislocations. Some individuals have hereditary “loose” joints and are more likely to experience a dislocated shoulder. 143 Predict 5 What combination of movements at the shoulder and elbow joints allows a person to perform a crawl stroke in swimming? 6.9 Effects of Aging on the Skeletal System and Joints Learning Outcome After reading this section, you should be able to A. Describe the effects of aging on bone matrix and joints. The most significant age-related changes in the skeletal system affect the joints as well as the quality and quantity of bone matrix. The bone matrix in an older bone is more brittle than in a younger bone because decreased collagen production results in relatively more mineral and less collagen fibers. With aging, the amount of matrix also decreases because the rate of matrix formation by osteoblasts becomes slower than the rate of matrix breakdown by osteoclasts. Bone mass is at its highest around age 30, and men generally have denser bones than women because of the effects of testosterone and greater body weight. Race and ethnicity also affect bone mass. African-Americans and Latinos have higher bone masses than caucasians and Asians. After age 35, both men and women experience a loss of bone of 0.3–0.5% a year. This loss can increase 10-fold in women after menopause, when they can lose bone mass at a rate of 3–5% a year for approximately 5–7 years (see Systems Pathology, “Osteoporosis”). Significant loss of bone increases the likelihood of bone fractures. For example, loss of trabeculae greatly increases the risk of fractures of the vertebrae. In addition, loss of bone and the resulting fractures can cause deformity, loss of height, pain, and stiffness. Loss of bone from the jaws can also lead to tooth loss. A number of changes occur within many joints as a person ages. Changes in synovial joints have the greatest effect and often present major problems for elderly people. With use, the cartilage covering articular surfaces can wear down. When a person Universal Free E-Book Store SySTEmS PaTHOLOGY Skeletal Osteoporosis background Information illiams Betty W : e Nam le : Fema Gender 65 age: nts Comme ed as smok Betty h ast for at le heavily es not o d. She s r a e y 50 goes , seldom e is rc e x e a poor rs, has o o td u o ht. derweig n u Betty ly t h g li s picnic, y is il d m n a fa ga right hip diet, ttendin d severe te r o p While a ight on he re any we d fell. S t n u a p d to e R. tripp ot able in the E d was n arrived e ss of h lo s pain an t n n e g wh nifica ig le s t h d ig le r a ve her r neck raphs re d femu re u t c Radiog a nd a fr ensity a bone d. 6B, 6C) (figures Osteoporosis, or porous bone, is a loss of bone matrix. This loss of bone mass makes bones so porous and weakened that they become deformed and prone to fracture (figure 6B). The occurrence of osteoporosis increases with age. In