mod 123.pdf
Document Details
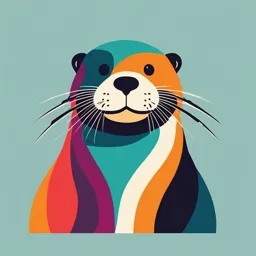
Uploaded by HardierMeadow
2023
Tags
Full Transcript
British Airways Global Learning Academy – Basic Aerodynamics - POTA 8.1 – Physics Of The Atmosphere Composition of the Earths’ Atmosphere Before discussing the fundamentals of the theory of flight, there are several basic ideas that must be considered. An aircraft operates in the air, therefore, the...
British Airways Global Learning Academy – Basic Aerodynamics - POTA 8.1 – Physics Of The Atmosphere Composition of the Earths’ Atmosphere Before discussing the fundamentals of the theory of flight, there are several basic ideas that must be considered. An aircraft operates in the air, therefore, the properties of air that affect aircraft control and performance must be understood. The air that surrounds the earth is a physical mixture of gases, and therefore follows the laws of gasses, it is also considered a fluid because it can be made to flow and change its shape even when a small pressure is applied. This mass of air is known as the atmosphere, which is a large mass of air extending upward hundreds of miles (approximately 500 miles or 804 km). So everything on the earth’s surface is under pressure due to the weight of the air. The pressure applied equals the weight of a column of air one square inch in cross sectional area that extends to the top of the atmosphere. This pressure equals 14.69 psi on the earth’s surface at sea level. Fig 1 – Composition Of The Atmosphere Air consists of several separate gases and the percentage as shown remains constant regardless of their altitude. The gasses referred to are shown in Figure 1 The region known as the Tropopause is a region, or boundary layer of atmosphere, somewhere between 20,000 feet and 60,000 feet above sea level. It is a portion of the troposphere and the stratosphere and is the region of the atmosphere where most fixed wing aircraft are confined to fly shown in figure 2. The ionosphere and exosphere are of little importance for commercial aircraft. The atmosphere is divided into four concentric gas layers starting from the earth’s surface and extending upward we have: a) b) c) d) Troposphere Stratosphere Ionosphere Exosphere 2 - (Approx. 7 miles /35,000 ft above sea level). - (Approx. 32 miles /160,000 ft above sea level). - (Approx. 200 miles above sea level). - (Approx. 500 miles above sea level). Module 8B ETBN 0492 October 2023 Edition 4 Possible Q Basic Aerodynamics – Physics Of The Atmosphere British Airways Global Learning Academy – Basic Aerodynamics - POTA The Tropopause marks the ceiling of the troposphere. It is found at levels varying from around 20,000ft over the Polar Regions to 60,000ft over the equator as seen in Figure 3. In the temperate latitudes, roughly mid-way between the tropics and the poles, the average altitude of the Tropopause is around 35000ft. The Tropopause is higher in summer than in winter, and it rises and falls as high- pressure and low pressure systems move under it. Fig 3 – Troposphere Altitude Variations Figure 2 – Earth’s Troposphere And Stratosphere Module 8B ETBN 0492 October 2023 Edition 5 Basic Aerodynamics – Physics Of The Atmosphere British Airways Global Learning Academy – Basic Aerodynamics - POTA International Standard Atmosphere (ISA) In order to have a reference for all aerodynamic computations, an organisation known as the International Civil Aeronautics Organisation (ICAO) has agreed upon an International Standard Atmosphere (ISA). The pressures, temperatures and densities in a standard atmosphere serve only as a reference. When all aerodynamic computations are related to this standard, a meaningful comparison of flight test data between aircraft can be made. The standard atmospheric conditions are based on a sea level temperature of 15°C or 59°F or 288K and a barometric pressure of 29.92 inches of mercury (inHg), or 1013.25 millibars (mb); also when we observe the chart we see the pressures, temperatures and densities all decrease with an increase in altitude. Temperature 15°C (59°F, 288.15K) Pressure 14.69 lbs/sq in (psi) 1013.25 millibars (mb) 29.92 inHg The ISA declares that the air pressure at sea level is 14.69 psi at a temperature of 15°C (59°F) and 6.75 psi at 20,000 feet indicating a 2 psi decrease for every 5,000 feet. Density Air density at Sea Level, although not on the chart in Figure 5, is 1.225kg/m3 (0.77lbs/ft3) 1.225 kg/m3 (0.77 lbs/ft3) Fig 4 -ISA Summary The standard temperature lapse rate, is 1.98°C or 3.5°F decrease in temperature every 1,000 feet in altitude up to 40,000 feet. However, air pressure is not constant; there are high and low pressure areas. The high-pressure air moves toward the low-pressure air, we witness this phenomenon as wind. Module 8B ETBN 0492 October 2023 Edition 6 Basic Aerodynamics – Physics Of The Atmosphere British Airways Global Learning Academy – Basic Aerodynamics - POTA Figure 5 – ISA Chart Module 8B ETBN 0492 October 2023 Edition 7 Basic Aerodynamics – Physics Of The Atmosphere British Airways Global Learning Academy – Basic Aerodynamics - POTA Pressure In the aviation industry there are four ways of referring to pressure: a) b) c) d) At the surface, pressure reduces at about one millibar per 27ft. By 20,000ft the rate of pressure reduction is about one millibar per 50ft, and by 30,000ft is about one millibar per 75ft Pounds per square inch (psi) Inches of mercury (inHg) Millibars (mb) Pascal (pa) Pressure is essentially caused by earth’s gravity pulling a column of air towards the earth’s surface. As the column of air nears the surface, the pressure increases over the given area, due to the air density and mass as seen in Figure 6. Gauge Pressure Pressures expressed in pounds per square inch (psi) usually do not take into consideration atmospheric pressure and refer to pressure in excess of atmospheric pressure. These pressures are generally read from a gauge and can be referred to as gauge pressure or psig. Fuel and oil pressures are measured in gauge pressure and indicate the amount a pump raises the pressure of the liquid above atmospheric pressure. You can see this when you open the valve on an oxygen cylinder. When this is done the oxygen rushes out until the pressure inside the cylinder equals that of the atmosphere or 14.69 psi. However, once the pressure equalises, the gauge on the cylinder reads zero. The same can be seen when you pump tyres, a flat tyre or empty tyre will indicate zero on the gauge. The reduction of pressure with height does not take place at a constant rate (Fig 6a). Module 8B ETBN 0492 October 2023 Edition Fig 6 – Air Pressure And The Effect Of Gravity 8 Basic Aerodynamics – Physics Of The Atmosphere British Airways Global Learning Academy – Basic Aerodynamics - POTA Absolute Pressure Absolute Pressure is pressure referenced from zero rather than from atmospheric pressure, it is known as absolute pressure, or psia. By definition, absolute pressure is gauge pressure plus atmospheric pressure. When a tube is filled with mercury and inverted in a bowl, the mercury drops in the tube until the atmospheric pressure exerted on the mercury in the bowl equals the weight of the mercury in the tube. Standard atmospheric pressure can support a column of mercury that is 29.92 inches tall. Fig 6a – Relationship Between Altitude And Pressure Figure 7 Gauge Pressure Module 8B ETBN 0492 October 2023 Edition 9 Basic Aerodynamics – Physics Of The Atmosphere British Airways Global Learning Academy – Basic Aerodynamics - POTA An altimeter (seen in Figure 9) measures absolute pressure and displays the result in feet above sea level. In aircraft, the absolute pressure within an engine induction system is indicated on a manifold pressure gauge. However, when the engine is not running, the gauge reads the existing atmospheric pressure. Another good example of this is the altimeter and readings are in feet rather than in inches of mercury. The metric unit of measure for barometric pressure is millibars. One millibar is approximately equal to 0.0295 inches of mercury. Figure 8 – Pressure Measurement Under standard sea-level conditions atmospheric pressure supports a column of 29.92 inches, or 760 millimetres high. Figure 9 – Altimeter (Three Pointer) Module 8B ETBN 0492 October 2023 Edition 10 Basic Aerodynamics – Physics Of The Atmosphere British Airways Global Learning Academy – Basic Aerodynamics - POTA Static And Dynamic Pressures Imagine a tube at rest. The tube is experiencing a pressure from the air around it. This pressure is known as the static pressure, and is the pressure exerted on all of us by the atmosphere all day and every day. Now let’s move the tube at 100Kts. There is now an additional pressure caused by the movement of the tube through the air. This pressure is known as the dynamic pressure, (pitot). Dynamic pressure increases as the tube’s speed increases; and will also increase if air density is increased. Dynamic pressure is given by: Fig 10 – Static And Dynamic (Pitot) Pressure Total Pressure Total pressure is the sum of all pressures acting on a surface. Normally this refers to static plus dynamic pressure. Module 8B ETBN 0492 October 2023 Edition 11 Basic Aerodynamics – Physics Of The Atmosphere British Airways Global Learning Academy – Basic Aerodynamics - POTA Temperature Air Density The temperature also varies throughout the atmosphere, and under normal circumstances it decreases with height. In the troposphere, the average rate of temperature change is about 1.98°C (2°C) for every 1000ft of altitude change. The rate of temperature change through the atmosphere is known as the lapse rate. Density is a term that means the mass of a material per unit volume and it varies with temperature and pressure in both liquids and gases. Two factors that are necessary to figure the density of a substance are mass and volume. Temperature is more critical when measuring the density of gases than when measuring the density of solids or liquids. The basic formula to find density is: There are two temperature scales with which you must be familiar. They are the Fahrenheit and the Celsius scales. The Celsius scale has 100 divisions between the freezing and boiling points of pure water. The Fahrenheit scale is not based on the freezing and boiling points of water so on this scale water freezes at 32° F and boils at 212°F. The standard temperature for all aerodynamic computations is 15°C or 59°F. 𝐷𝑒𝑛𝑠𝑖𝑡𝑦 = 𝑀𝑎𝑠𝑠 𝑉𝑜𝑙𝑢𝑚𝑒 Because air is a mixture of gases, it can be compressed. If the air in one container is under one-half as much pressure as the air in another identical container, the air under the greater pressure weighs twice as much as the air in the container under low pressure. The air under greater pressure is twice as dense as that in the other container. The density of gases is governed by the following rules: 1. Density varies in direct proportion with the pressure. 2. Density varies in inverse proportion with the temperature. Therefore air at high altitudes is less dense than air at low altitudes, and a mass of hot air is less dense than a mass of cool air. Air Density and Lift Changes in density affect the aerodynamic performance of an aircraft. Using the same thrust or power setting, an aircraft can fly faster at high altitudes where the density is low, than it can at lower altitudes where the density of the air is much greater. Figure 11 – UK VFR Temperature Conversion Module 8B ETBN 0492 October 2023 Edition 12 Basic Aerodynamics – Physics Of The Atmosphere British Airways Global Learning Academy – Basic Aerodynamics - POTA This is because air offers less resistance to the aircraft when it contains a smaller number of air particles per unit volume. On hot days, the density of the air is less than on cold days. On wet days, the density is less than on dry days. However, you will see that the amount of lift generated depends upon the shape of the aerofoil, the angle of attack, and the air speed. Another factor that affects lift is the air density. Lift varies directly with air density. Also density decreases or becomes less with an increase in altitude. When the density is low, the lift will also be comparatively lower. Therefore, the airspeed must increase as the density decreases in order to maintain the aircraft at the same angle of attack in level flight. The amount of lift produced by an aerofoil depends on the number of pounds of air it can push down. At 18,000 feet, where the density of the air is just half as much as at sea level, an aircraft would have to travel approximately 1.5 times as fast as it would at sea level to maintain altitude. Therefore aircraft can’t fly too high and it is between 30,000 feet and 45,000 feet that most commercial aircraft fly. It is at this altitude that the best power to weight ratio and lift coefficient is obtained. Humidity As we know, the air is seldom completely dry. It does contain a little moisture, either in the form of fog, or water vapour. Fog consists of minute droplets of water held in suspension by the air. Clouds are composed of fog. The moisture in the air is often referred to as humidity, and the maximum amount of water vapour that air can hold varies with the temperature. The higher the temperature of the air, the more water vapour it can absorb. By itself, water vapour weighs approximately 5/8 as much as an equal amount of perfectly dry air. Therefore, when air contains water vapour it is not as heavy as air containing no moisture. Remember, pure dry air contains about 78% nitrogen and 21% oxygen. Fig 12 – Air Density And Lift Module 8B ETBN 0492 October 2023 Edition 13 Basic Aerodynamics – Physics Of The Atmosphere British Airways Global Learning Academy – Basic Aerodynamics - POTA Assuming that the temperature and pressure remain the same, the density of the air varies inversely with the humidity. On damp days the air density is less than on dry days. For this reason, an aircraft requires a longer runway for take-off on damp days than it does on dry days. Absolute Humidity Absolute humidity refers to the actual amount of water vapour in a mixture of air and water. Relative Humidity Refers to the ratio between the amounts of moisture in the air, to the amount that would be present, if the air were saturated. Fog and humidity both affect the performance of an aircraft. For example, because humid air is less dense than dry air, the allowable take-off gross weight of an aircraft is generally reduced when operating in humid conditions. Dew Point This is the temperature of the air where it can no longer hold any more water vapour. When the dew point is reached, the air contains 100% of the moisture it can hold at that temperature and is now said to be saturated. If the temperature drops below the dew point, condensation occurs. Secondly, because water vapour is incombustible, its presence in the atmosphere results in a loss of engine power output. This power loss is more on piston engines than on turbine engines. Figure 14 – Dew Point And Cloud Formation Fig 13 – Illustration Of Water Vapour In Air Module 8B ETBN 0492 October 2023 Edition 14 Basic Aerodynamics – Physics Of The Atmosphere British Airways Global Learning Academy – Basic Aerodynamics - POTA Acceleration Due to Gravity As the force is inversely proportional to the distance squared this means the attraction at the poles is greater than at the equator. If a small body is sufficiently close to the earth to experience the pull due to gravity the attraction will cause the body to move toward the surface of the earth. The acceleration is constant, i.e. gravity produces a constant acceleration of 9.81 m/s2 or 32.2 ft/s2 regardless of the mass of the body. 1. At the poles g = 32.3 ft/s2 2. In the U. K. g = 32.2 ft/s2 3. At the equator g = 32.1 ft/s2 If a body is allowed to fall freely under the influence of gravity it will suffer constant acceleration due to gravity. The term “freely” is used to indicate that there is no force resisting its downward movement. You can now see that flight through the atmosphere is not as simple as one thinks, and many graphs and calculations must be made and specialised equipment used like the highly sophisticated fuel control units used on modern commercial aircraft. In this condition the speed will continue to build up. In practice however, there is a force resisting the downward motion provided by the atmosphere, i.e. the friction due to movement through the air. These calculations and equipment have to take Pressure, Temperature, Density and Humidity into account when calculating the best power to weight ratio and the correct altitude for our commercial aircraft to fly safely and economically This limits the build-up of speed until a point is reached where retardation due to air friction is equal to the acceleration due to gravity, and no further acceleration occurs. The body has then reached its terminal velocity. Viscosity In streamline motion, layers of fluid travel at different speeds (like traffic in the lanes of a motor-way) and slide over one another. Forces which are in essence equivalent to frictional forces are set up between two layers of a fluid which are in relative motion. This is called viscosity. Because the acceleration due to gravity is constant and applies to all bodies irrespective of their mass, different bodies would undergo the same acceleration when allowed to fall freely, for example a stone and a feather both falling in a vacuum will reach the bottom together but have different accelerations when falling through the atmosphere. If you stir a cup of tea which has tea leaves floating on top so that a circular motion takes place you will see that the leaves near the centre revolve more quickly than do those near the edge. Acceleration (g) due to gravity, although said to be constant, does vary slightly at different parts of the earth’s surface. This is because the distance from the surface to the centre of the earth is not exactly the same at all points. The earth is flatter at the poles than at the equator. Module 8B ETBN 0492 October 2023 Edition If we imagine the liquid to consist of a number of concentric vertical thin cylinders, there is relative motion between these cylinders, the liquid in contact with the side of the cup being at rest. 15 Basic Aerodynamics – Physics Of The Atmosphere British Airways Global Learning Academy – Basic Aerodynamics - POTA Frictional forces exist between these cylinders, when they move relatively to each other, which eventually stop the motion. In a highly viscous liquid such as glycerine the motion ceases almost at once. The higher the viscosity of a liquid then the longer the time it will take to pour out of a container. An example of this situation could be an aircraft wing skin traveling through stationary air where the moving boundary is the wing skin and the fixed boundary is the stationary air, a small distance away from the skin. Viscosity is a property of both liquids and gases. Viscosity in gases can be explained in terms of molecular movement. Distance from stationary boundary y Very briefly, in the case of a gas it is due to molecules moving from the slower moving layers into the faster moving layers, and from the faster moving layers to the slower moving layers. The net result of this is that more momentum is carried one way than the other, which means that forces between the layers retard the faster moving layers and accelerate the slower moving ones. Moving boundary Velocity = v Stationary boundary Velocity = 0 We define viscosity as: “The property of a fluid that offers resistance to the relative motion of its molecules”. The energy losses due to friction within a fluid are dependent on its viscosity. As a fluid moves, there is developed a shear stress in it, the magnitude of which depends on the viscosity of the fluid. Figure 15 – Velocity Change Between Fixed And Moving Boundaries The diagram illustrates the concept of velocity change in a fluid by showing a thin layer of fluid (a boundary layer) sandwiched between a fixed and moving boundary. The boundary layer is a thin layer of fluid between a fixed and moving boundary, across which a velocity change takes place. Module 8B ETBN 0492 October 2023 Edition 16 Basic Aerodynamics – Physics Of The Atmosphere British Airways Global Learning Academy – Basic Aerodynamics UK Part 66 Module 8B Basic Aerodynamics 8.2 Aerodynamics Book 2 of 4 Module 08B ETBN 0492 October 2023 Edition Basic Aerodynamics – Aerodynamics British Airways Global Learning Academy – Basic Aerodynamics Contents Syllabus ................................................................................................................................................................................................................... 4 8.2 – Aerodynamics ................................................................................................................................................................................................. 5 Bernoulli’s Theorem ................................................................................................................................................................................................. 5 Convergent/Divergent Ducts ................................................................................................................................................................................. 5 Compressibility ..................................................................................................................................................................................................... 7 Application Of Bernoulli’s Principle ........................................................................................................................................................................ 9 Airflow Around A Body ........................................................................................................................................................................................... 11 Skin Friction and Viscosity .................................................................................................................................................................................. 11 Free Stream Flow ............................................................................................................................................................................................... 12 Relative Airflow (RAF) ........................................................................................................................................................................................ 16 Up-Wash, Downwash & Stagnation Point ............................................................................................................................................................ 17 Vortices .............................................................................................................................................................................................................. 17 Aerodynamics Terminologies ................................................................................................................................................................................. 18 Aerofoils ............................................................................................................................................................................................................. 18 Chord Line.......................................................................................................................................................................................................... 20 Camber And Wing Shape ................................................................................................................................................................................... 20 Angle of Attack ................................................................................................................................................................................................... 20 Fineness Ratio & Thickness/Chord Ratio ............................................................................................................................................................ 21 Aspect Ratio (AR) And Wing Planforms .............................................................................................................................................................. 22 Mean Aerodynamic Chord (MAC) ....................................................................................................................................................................... 26 Wash-In.............................................................................................................................................................................................................. 27 Module 08B ETBN 0492 October 2023 Edition Basic Aerodynamics – Aerodynamics British Airways Global Learning Academy – Basic Aerodynamics Wash-Out ........................................................................................................................................................................................................... 27 Total Reaction (TR) ............................................................................................................................................................................................ 28 Centre of Pressure (CoP) ................................................................................................................................................................................... 28 Aerodynamic Drag .............................................................................................................................................................................................. 29 Profile/Parasite Drag .......................................................................................................................................................................................... 30 Induced Drag ...................................................................................................................................................................................................... 34 Thrust, Weight And Aerodynamic Resultant............................................................................................................................................................ 37 Generation Of Lift And Drag ................................................................................................................................................................................... 38 Lift And Lift Co-efficient (CL)................................................................................................................................................................................ 39 Angle of Attack (AoA) ......................................................................................................................................................................................... 40 Aerodynamic Stall............................................................................................................................................................................................... 41 Drag And Drag Co-efficient (CD).......................................................................................................................................................................... 47 Drag Polar Curve ................................................................................................................................................................................................ 49 Lift/Drag Ratio .................................................................................................................................................................................................... 50 Aerofoil Contamination ........................................................................................................................................................................................... 51 Ice Formation And Effects of Ice, Snow And Frost............................................................................................................................................... 51 Types of Ice And Snow ....................................................................................................................................................................................... 54 Module 08B ETBN 0492 October 2023 Edition Basic Aerodynamics – Aerodynamics British Airways Global Learning Academy – Basic Aerodynamics Syllabus UK Part 66 Syllabus Level Basic Aerodynamics 8.2 Aerodynamics A B B2 / B2L B3 Airflow around a body; 1 2 2 1 Boundary layer, laminar and turbulent flow, free stream flow, relative airflow, upwash and downwash, vortices, stagnation; The terms: camber, chord, mean aerodynamic chord, profile (parasite) drag, induced drag, centre of pressure, angle of attack, wash in and wash out, fineness ratio, wing shape and aspect ratio; Thrust, Weight, Aerodynamic Resultant; Generation of Lift and Drag: Angle of Attack, Lift coefficient, Drag coefficient, polar curve, stall; Aerofoil contamination including ice, snow, frost. Module 08B ETBN 0492 October 2023 Edition 4 Basic Aerodynamics – Aerodynamics British Airways Global Learning Academy – Basic Aerodynamics 8.2 – Aerodynamics Convergent/Divergent Ducts Bernoulli’s Theorem The most important application of Bernoulli’s theorem is when looking at convergent and divergent duct. The principle of the conservation of energy has already been discussed, earlier in the physics module. A convergent duct (Figure 1) is defined as a duct that has a bigger entrance cross-sectional area (a1) than exit area (a2). This principle is equally valid for fluids in motion, as it is for solids. One such application of this principal to fluid flow is Bernoulli’s equation Then Bernoulli’s theorem states: From the principle of conservation of mass flow we have already seen that as the cross sectional area of the duct decreases so the velocity of the fluid increases. 1 1 𝜌𝑣 + 𝑝 = 𝜌𝑣 + 𝑝 = 𝐶 2 2 Where P1 and P2 are the static pressures in the fluid flow 𝜌𝑣 If we now apply this change in fluid velocity to Bernoulli’s equation we see that the dynamic pressure (PD) is actually a function of the fluid velocity (v), such that: 𝜌𝑣 and are the dynamic pressures in the fluid flow 𝑃 = This equation is only valid for incompressible flow and yields a wealth of information. The equation tells us that, as the flow progresses from one point to another, an increase in velocity is accompanied by a decrease in pressure. This follows because ( 1 × 𝜌 × 𝑣 2 This means that as the velocity of the fluid increases as it passes into the narrowing duct, so the dynamic pressure will also increase. 𝜌𝑣 ) is the sum of the static pressure (p) and dynamic pressure is a constant along a streamline We already know that Bernoulli’s equation says that the dynamic pressure plus the static pressure gives a constant value. The total pressure being the sum of the static and dynamic pressures, while the name stagnation arises from the fact that when the velocity is reduced to 0 (stagnation), the stagnation pressure is equal to the total pressure. Since the value of the dynamic pressure is rising, this must mean that the value of the static pressure must drop. Module 08B ETBN 0492 October 2023 Edition 5 Basic Aerodynamics – Aerodynamics British Airways Global Learning Academy – Basic Aerodynamics A divergent duct is the complete opposite of a convergent duct. Here we have the cross-sectional area of the duct increases as the fluid travels through the duct. Again by the principle of conservation of mass flow we see that as the cross sectional area of the duct increases, so the velocity of the fluid travelling through it will decrease. Again by Bernoulli’s equation we see that the dynamic pressure is a function of the velocity of the fluid, so since the velocity of the fluid is decreasing, the dynamic pressure will also decrease. Since the sum of the dynamic and static pressure is constant, we can now see that since the dynamic pressure is decreasing, so the static pressure must increase. Table 1 summarises how static and dynamic pressure change in both a converging and diverging duct below the speed of sound (340 m/s at sea level under standard ISA conditions). Table 1 – Converging/Diverging Duct Summary Table Figure 1 Convergent Duct Module 08B ETBN 0492 October 2023 Edition q 6 FLUID VELOCITY DYNAMIC PRESSURE PRESSURE Converging Increasing Increasing Decreasing Diverging Decreasing Decreasing Increasing POSSIBLE Q STATIC I DUCT TYPE Basic Aerodynamics – Aerodynamics British Airways Global Learning Academy – Basic Aerodynamics This will cause the air flow to slow down and give a reduced velocity (V2). Compressibility So far all of our work on fluids has been based on the assumption that fluids are incompressible. The static pressure of the air will increase (Bernoulli’s theorem and Table 1) to become P2 in the wider section of the duct but, because air is compressible, the air density will increase as it is compressed by the rise in pressure in the divergent part of the duct. This is certainly true for the practical application of fluid theory to liquids such as water but not so for air, which is most definitely compressible! In Figure 2, For the supersonic, compressible air flow, the air entering the convergent part of the duct, consists of decreasing air pressure (P1) and velocity (V1); then as the air enters the divergent part of the duct, with the increased area, air will spread out to fill the increased area. Our theory based on the incompressible behaviour of fluids is still sufficiently valid for air when it flows below speeds of approximately 130–150 m/s. As speed increases compressibility effects become more apparent and Table 2 demonstrates the reverse effects of fluid velocity, static and dynamic pressures due to air compressibility at speeds approaching, near too or above the speed of sound (Mach 1.0). This will cause the air flow to increase and give a reduced velocity (V2). The static pressure of the air will decrease (Table 2) to become P2 in the wider section of the duct and, because air is compressible, the air density will decrease as it is de-compresses by the fall in pressure in section B of the duct. Therefore, when we study high-speed flight where aircraft fly at velocities approaching, close too, or in excess of, the speed of sound, also known as Mach 1.0 (340 m/s at sea level under standard ISA conditions), then the compressibility effects of air must be considered. Table 2 – Convergent/Divergent Duct Summary (At/above Mach1.0) This is particularly true when considering the possible inaccuracies in aircraft pitot–static instruments, where such instruments depend on true static and dynamic air pressures for their correct operation. In Figure 2, For the subsonic air flow, the air entering the convergent part of the duct, consists of increasing air pressure (P1) and velocity (V1); then as the air enters the divergent part of the duct, with the increased area, air will spread out to fill the increased area. Module 08B ETBN 0492 October 2023 Edition 7 DUCT TYPE FLUID VELOCITY DYNAMIC PRESSURE STATIC PRESSURE Converging Decreasing Decreasing Increasing Diverging Increasing Increasing Decreasing Basic Aerodynamics – Aerodynamics British Airways Global Learning Academy – Basic Aerodynamics Fig 2 – Convergent/Divergent Sub Sonic/Supersonic comparisons Module 08B ETBN 0492 October 2023 Edition 8 Basic Aerodynamics – Aerodynamics British Airways Global Learning Academy – Basic Aerodynamics Application Of Bernoulli’s Principle We see how lift is produced, using the venturi tube in Figure 3.. If we remove the upper surface, we find that the streamlines themselves provide the upper boundary of the venturi tube since the flow is subsonic and cannot compress. The velocity of the airflow increases until it reaches the narrowest point in the tube. We know that as the velocity increases the static pressure decreases and the dynamic pressure increases. The next step is to change the lower surface of the venturi tube into an aerodynamic profile and to add some streamlines below it. The velocity decreases again after the narrowest point and returns to the inlet level by the time the airflow reaches the outlet. During this phase the static pressure increases again and the dynamic pressure decreases. Now we have a surface with an area of low static pressure above it and area of unchanged static pressure below it. We, now have a difference in pressure across the top and bottom of the wing. Now let’s imagine replacing the upper surface of the venturi tube with a straight line and see what happens to the airflow. This doesn’t change things very much. From fluid dynamics you should remember that the product of the resultant pressure acting across a given surface area creates a force in the direction of the resultant pressure hence the force of lift is produced. The streamlines are still closer to each other in the centre and the static pressure decreases in this area. Module 08B ETBN 0492 October 2023 Edition 9 Basic Aerodynamics – Aerodynamics British Airways Global Learning Academy – Basic Aerodynamics Top of tunnel replaced with flat surface Area of compressed streamlines Flat surface removed Bottom surface profile smoothed Figure 3 - Application Of Bernoulli’s Principle Module 08B ETBN 0492 October 2023 Edition 10 Basic Aerodynamics – Aerodynamics British Airways Global Learning Academy – Basic Aerodynamics Airflow Around A Body Skin Friction and Viscosity Skin Friction is caused by a tendency of the particles of air to cling to the surface of the aircraft. There are two reasons for this tendency. Firstly, the aircraft has a certain amount of roughness, relatively speaking, it is impossible to make the skin perfectly smooth. It could also be dirty which would have the same affect. Secondly, the air tends to cling to the surface and this is due to the air having viscosity. Technically, viscosity is the resistance to flow. It is commonly used to describe the adhesive or sticky characteristics of a fluid (see figure 4 with the effect of air layers). Figure 4 – Effect Of Viscosity On Airflow Think of oil and water; oil is much more viscous than water. The oil finds it more difficult to flow than water, therefore has a higher viscosity. Viscosity is generally a function of temperature alone; a decrease in temperature increases the viscosity (thickness). Therefore, viscosity usually increases with altitude. Fig 4a – Effect Of Viscosity Around An Aerofoil Module 08B ETBN 0492 October 2023 Edition 11 Basic Aerodynamics – Aerodynamics British Airways Global Learning Academy – Basic Aerodynamics Free Stream Flow When a fluid, liquid or gas is flowing steadily over a smooth surface, narrow layers of it follow smooth paths that are known as streamlines. This smooth flow is also known as laminar flow and will be discussed later in this section. When air flows around a non-streamlined object the air swirls into eddies, the streamlines distort and intermingle, and finally disappear. The airstream has now become turbulent. If this stream meets large irregularities, the streamlines are broken up and the flow becomes irregular or turbulent, as may be seen when a stream comes upon rocks in the river bed. The effort required to force a flat plate through the air is about 20 times that required for a streamlined shape for the same speed and with a similar frontal area presented to the air. By introducing smoke into the airflow in a wind tunnel (as we can see in figure 4b) or coloured jets into water tank experiments, it is possible to see and photograph these streamlines and eddies. Much of the difference arises from the energy needed to form air eddies behind the flat plate. A tube, which comes smoothly to a narrow constriction and then widens out again is known as a venturi tube. The following rules apply to streamlines: Streamlines never cross The closer streamlines are together the faster the fluid velocity and conversely the lower the static pressure. Module 08B ETBN 0492 October 2023 Edition 12 Basic Aerodynamics – Aerodynamics British Airways Global Learning Academy – Basic Aerodynamics REAL LIFE STREAMLINES COMPUTER GENERATED STREAMLINES Figure – 4b – Free Streamline Flows Module 08B ETBN 0492 October 2023 Edition 13 Basic Aerodynamics – Aerodynamics British Airways Global Learning Academy – Basic Aerodynamics Boundary Layer Laminar And Turbulent Flow The term laminar flow describes the situation when air is flowing in thin sheets, or layers, close to the surface of an aircraft's wing with no disturbance between the layers of air, that is there is no cross flow or sideways movement of the air particles with respect to the direction of the airflow. Normally the airflow at the leading edge of a wing will be laminar, but as the air moves toward the trailing edge of the wing, the boundary layer becomes thicker and turbulent. (Fig 5a) Laminar flow is most likely to occur where the surface is extremely smooth. The area where the airflow changes from laminar to turbulent is called the transition point or region. It is desirable to keep the air flow as laminar or as smooth as possible over the aerofoil. The boundary layer (Fig 5) is that layer of air adjacent to the aerofoil surface and is approximately 1/16 of an inch thick. The air velocity in the boundary layer varies from zero, on the surface of the aerofoil, to the velocity of the free air stream at the outer edge of the boundary layer. The boundary layer is caused by the viscosity of the air sticking to the surface of the wing and the succeeding layers of air. Fig 5a – Laminar to Turbulent Airflow over An Aerofoil Figure 5 - Variation In Airflow Speed in the Boundary Layer Module 08B ETBN 0492 October 2023 Edition 14 Basic Aerodynamics – Aerodynamics British Airways Global Learning Academy – Basic Aerodynamics Due to the friction and pressure gradient existing within the boundary layer, the boundary layer, itself, may be brought to rest. This is due to the fact that the turbulent air produces an increase in static pressure above that of the boundary layer in front of it. At this point the streamlined flow will now not have the energy to push through this area of high pressure. When this occurs, the layer will break away from the surface to form a turbulent wake. The point at which this takes place is called the Separation Point We can now define the Boundary Layer as that area where shearing takes place between successive layers of air or between the surface and the full flow velocity of the airstream. It is worth noting that the boundary layer can be either ‘lamina’ or turbulent. We can see the full range of Boundary Layer behaviour in figure 5b Figure 5b – Boundary Layer Behaviour Module 08B ETBN 0492 October 2023 Edition 15 Basic Aerodynamics – Aerodynamics British Airways Global Learning Academy – Basic Aerodynamics Relative Airflow (RAF) The Relative Airflow (Wind) is the angle or direction that air passes over or across an aerofoil surface (wing) of an aircraft. As can be seen in figure 6 and 6a the Relative Air Flow is not the angle at which the aircraft is climbing or descending but the direction the airflow passes over the aerofoil, relative to the aerofoil and is a critical distinction as the Relative Airflow provides the lift for an aerofoil. A very high or low Relative Airflow (Wind) is a crucial factor on the lift or drag produced by the aerofoil and this will be discussed later. Relative Airflow is always in the opposite the direction to the aircrafts’ forward flight path velocity Fig 6a – Relative Airflow with respect to the Aerofoil Fig 6 – Relative Airflow (Wind) with respect to an aircraft Module 08B ETBN 0492 October 2023 Edition 16 Basic Aerodynamics – Aerodynamics British Airways Global Learning Academy – Basic Aerodynamics Up-Wash, Downwash & Stagnation Point As air flows towards an aerofoil it will be turned towards the lower pressure at upper surface, this is termed up-wash. After passing over the aerofoil the air flow returns to its original position and state, this is termed downwash. Figure 8 – Wing Tip Vortices Upper and Lower Surfaces Direction Fig 7 - Up-wash and Downwash The point on the leading edge of an aerofoil (A) and trailing edge (B) where the airflow separates, some going over the surface and some below. This is known as stagnation point. Vortices Wing tip vortices are formed because the wing develops lift. That is to say the pressure on the top of the wing is lower than on the bottom; and near the tips of the wing this pressure difference causes the air to move around the edge from the bottom surface to the top (Fig 8). This results in a rotational movement of the fluid (air) forming the trailing vortex. As viewed from the rear, they rotate clockwise from the left wing and anti-clock wise from the right wing (Fig 8a and 8b). Module 08B ETBN 0492 October 2023 Edition Figure 8a – Wing Tip Vortices Being Generated 17 Basic Aerodynamics – Aerodynamics British Airways Global Learning Academy – Basic Aerodynamics The strength of the vortex is related to the amount of lift generated by the wing so they become particularly strong in high-lift conditions such as take-off and landing. Aerodynamics Terminologies They also increase in strength with the size of the airplane, because the lift is equal to the weight of the aeroplane. For a large transport aeroplane such as an Airbus A380 or Boeing 747, the vortices are strong enough to roll a small aeroplane onto its back if it gets too close. Aerofoils The aerofoil is a shaped surface designed to produce a reaction when moved through an airstream. Present day aerofoils are devised to produce a high reaction force with as little resistance as possible. When comparing the flat plate to the aerofoil, it was discovered that a curved instead of a flat plate produced a much greater lift force than the drag. Thus the modern aerofoil was developed. As stated, an aerofoil is a surface designed to obtain a desirable reaction from the air through which it moves. Therefore, we can say that any part of an aircraft, which converts air velocity into a force useful for flight, has an aerofoil shape. The blades of a propeller are so designed that when they rotate, their shape and position cause a low pressure to form in front of them and a higher pressure behind them so they pull the aircraft forward. Likewise, the blades on a helicopter and also those used in a jet engine are aerofoil shaped. Figure 9 shows a selection of aero foil designs Figure 8b – Wing Tip Vortices On Starboard Wingtip PORT Module 08B ETBN 0492 October 2023 Edition 18 Basic Aerodynamics – Aerodynamics British Airways Global Learning Academy – Basic Aerodynamics m Figure 9 – Aerofoil Design Shapes Module 08B ETBN 0492 October 2023 Edition 19 Basic Aerodynamics – Aerodynamics British Airways Global Learning Academy – Basic Aerodynamics Chord Line The straight line joining the centres of curvatures of the leading and trailing-edges (Figure 10a). Chord length is the distance between the leading and the trailing edge measured along the chord line Camber And Wing Shape The curvature of the aerofoil from the leading edge to the trailing edge; "upper camber” refers to the curvature of the upper surface, "lower camber" refers to the curvature of the lower surface (Fig 10). my On the upper surface a large camber will give good lift (increased area), but high drag (greater mass) and therefore, low speed (Fig 10) The Mean Camber Line is the line joining the leading and trailing edges of the section equidistant from the upper and lower surfaces (Fig 10). Fig 10. – Aerofoil Key Terminologies If the upper camber is larger than the lower camber the wing is said to be asymmetrical and the velocity differential increases (Fig 9). If the upper and the lower camber are the same, the wing is said to be symmetrical. This type of wing relies on a positive angle of attack to generate lift and is generally used on high performance, acrobatic aircraft (Figure 9). Angle of Attack The angle between the chord line of the section and the direction of the oncoming airflow (Figure 10a) This should not be confused with the Angle of Incidence, which is the angle between the chord line and the longitudinal axis of the aircraft (Fig 10a). Module 08B ETBN 0492 October 2023 Edition Figure 10a – Angle of Incidence And Chord Line 20 Basic Aerodynamics – Aerodynamics British Airways Global Learning Academy – Basic Aerodynamics Fineness Ratio & Thickness/Chord Ratio Two ratios used for discussing the cross section of a wing. The Fineness ratio looks at the length of the chord compared to the maximum thickness, whilst the Thickness/Chord ratio looks at it the other way around, as shown (Figure 11). A thick aerofoil section with a large cambered top surface will give high lift characteristics accompanied with high drag characteristics also but is suitable where high forward speeds are not required. The large camber also allows the use of deep spars. The maximum thickness is about 25% to 30% of the chord aft of the leading-edge. Where high forward speed is of more importance, the high lift aerofoil section can be modified. The maximum thickness remains at about 25% to 30% chord, but the thickness/chord ratio is reduced whilst the top surface is given a less pronounced camber. This results in a section which possesses smaller lift and drag characteristics than that of a high lift section. Where speed is the only criteria, drastic changes in the section are made. The Chord/Thickness ratio is somewhere in the region of 5% to 10%, the leading edge is given a knife-edge whilst the top surface has little or no camber. These changes, of course, lead to low lift and drag characteristics, but experiments to improve the air circulation around the section are continuously in progress to increase the lift whilst retaining the low drag. Module 08B ETBN 0492 October 2023 Edition Figure 11 – Fineness Ratio 21 Basic Aerodynamics – Aerodynamics British Airways Global Learning Academy – Basic Aerodynamics Wing Planforms And Characteristics Aspect Ratio (AR) And Wing Planforms The Aspect Ratio (AR) of a wing is the ratio of the wing span to the average chord. It is determined with this formula. The greater number of times the chord will divide into the span the higher the aspect ratio will be. Wing Area (S) - The plan surface area of the wing. Wing Span (b) - The distance from tip to tip. Average Chord (c) - The geometric average. Root Chord (CR) - The chord length at the wing centre line Tip Chord (CT) - The chord length at the tip. Taper Ratio (CT/CR) - The ratio of the tip chord to the root chord. The taper ratio affects the lift distribution and structural weight of the wing. A rectangular wing has a taper ratio of 1.0 while the point tip delta wing has a taper ratio of 0.0. The wing with a high aspect ratio gives a better lift/drag ratio than the wing with a low aspect ratio for reasons to do with drag which are dealt with later. As can be seen in Figures 12 and 12a, a wing with high Aspect Ratio is ideal for a non-powered aircraft, which requires lower speeds, higher lift/drag ratio but lower manoeuvrability. Conversely the lower Aspect Ratio wing is for higher speeds and higher manoeuvrability but has a lower lift/drag ratio. The aspect ratio is one of the primary factors in determining wing lift/drag characteristics. Sometimes it is expressed as the ratio of the square of the wing-span to the wing area. Figure 12 – Wing Planforms Module 08B ETBN 0492 October 2023 Edition 22 Basic Aerodynamics – Aerodynamics British Airways Global Learning Academy – Basic Aerodynamics Fig 12a – Wing-Shape And Aspect Ratio Comparisons Module 08B ETBN 0492 October 2023 Edition 23 Basic Aerodynamics – Aerodynamics British Airways Global Learning Academy – Basic Aerodynamics Dihedral/Anhedral The Dihedral of the wing is the angle formed between the wing and the horizontal plane passing through the root of the wing. We have a positive dihedral when the tip of the wing is above the horizontal plane and a negative dihedral when the tip of the wing is below the horizontal plane – also known as Anhedral. Ior Sweep Angle The sweep angle is the angle between the quarter cord, or the 25 % line and the pitch axis. Positive sweep = Backwards Negative sweep = Forwards Figure 12b – B787 with Dihedral Wing Design Figure 12d illustrates positive sweep angle, its’ measurement datum and some typical swept wing design configurations. Fig 12c – BAe146 with Anhedral Wing Design Module 08B ETBN 0492 October 2023 Edition 24 Basic Aerodynamics – Aerodynamics British Airways Global Learning Academy – Basic Aerodynamics Low sweep angle Moderate sweep angle High sweep angle Figure 12d – Sweep Angle Illustrations Module 08B ETBN 0492 October 2023 Edition 25 Basic Aerodynamics – Aerodynamics British Airways Global Learning Academy – Basic Aerodynamics Mean Aerodynamic Chord (MAC) The MAC is the mean (average) chord of the wing. This measurement is actually the determining factor used by the manufacturer to select the C of G location on aircraft Today, on transport aircraft, irregularly shaped wings are used almost exclusively. In most cases a taper, crescent, swept back or swept forward wing or a combination of these types are used. For this reason it is advantageous to express the C of G range in MAC percentages rather than in inches from the datum (Figure 13). Figure 13a - MAC Position On a Taper Wing Figure 13b - MAC On A Straight Wing Figure 13 – C of G MAC Range On Aircraft Module 08B ETBN 0492 October 2023 Edition 26 Basic Aerodynamics – Aerodynamics British Airways Global Learning Academy – Basic Aerodynamics Wash-In This is a condition in which a wing has an increase in its angle of incidence near the tip (Figure 14 Lower). Wash-Out A tendency to stall at the tip section first has dangerous implications for the lateral control and stability of the aircraft. The wing can be designed so that the root stalls before the tip and the aircraft remains controllable. This is achieved by physically, geometrically twisting the wing. (Figure 14 Upper), or by aerodynamically twisting the wing (camber of the profile at the root is greater than the camber at the tip and the angle of incidence is constant across the wing span). The outboard sections (toward the wing tips) will reach the stalling angle after the inboard sections, thus allowing effective wing flight control the stall progresses. Figure 13c – MAC On A Swept Wing To determine the MAC on a typical sweptback wing as in Figure 13c, it would be measured chord wise at the root and the tip. These measurements would be used to determine the average aerofoil section. For example: the root measurement of a certain wing is 144 inches and the tip is 72 inches. The MAC would be 108 inches. The leading edge of the mean aerodynamic chord is abbreviated LEMAC. The trailing edge of the mean aerodynamic chord is abbreviated TEMAC. The centre of gravity will always lie between LEMAC and TEMAC. The centre of gravity is expressed as a percentage behind LEMAC. Figure 14 – Twisted Wing Geometric Wash-In And Wash-Out Module 08B ETBN 0492 October 2023 Edition 27 Basic Aerodynamics – Aerodynamics British Airways Global Learning Academy – Basic Aerodynamics POSSIBLE Total Reaction (TR) The result of all the aerodynamic forces acting on the wing or aerofoil section is shown in Figure 15. Centre of Pressure (CoP) A point, usually on the chord line through which the total reaction (minimum pressure) may be considered to act. At a low Angle Of Attack (AOA) the Centre Of Pressure is set further back on the Chord line when compared to an increasing AoA when the Centre Of Pressure moves forward toward the Leading Edge of the aerofoil (Figure 16). This will be explained in more detail under the Generation of Lift And Drag section of these notes. Figure 16 - Centre of Pressure Figure 15 - Total Reaction Module 08B ETBN 0492 October 2023 Edition 28 Basic Aerodynamics – Aerodynamics Q British Airways Global Learning Academy – Basic Aerodynamics Total Drag Aerodynamic Drag Drag is the aeronautical term for the air resistance experienced by the aircraft as it moves relative to the air. Subsonic It acts in the opposite direction to the motion through the air. i.e. It opposes the motion and acts parallel to and in the same direction as, the relative airflow. Total Drag is the total resistance to the motion of the aircraft through the air. Induced Drag Supersonic Profile Drag The total Drag is the sum total of the various Drag forces (Figure 17) acting on the aircraft. These forces may be divided up to: Subsonic Drag Supersonic Drag Supersonic Drag will be discussed in the “High Speed Flight”, section of module 11 notes. So let us consider Subsonic Drag and its component parts. Profile Drag is made up from, Skin Friction, Form Drag and Interference Drag. Let us look at these types of Drag in more detail. Figure 17 – Types Of Drag Forming Total Drag Module 08B ETBN 0492 October 2023 Edition 29 Basic Aerodynamics – Aerodynamics British Airways Global Learning Academy – Basic Aerodynamics Profile/Parasite Drag The layer or layers in which this shearing action takes place, i.e. between the surface and that point of the flow which is travelling at the full velocity of the flow, is called the Boundary Layer (Figure 17a) When an aircraft is passed through an airflow, the air offers a resistance to the passage of the aircraft. This aerodynamic resistance is known as drag. The initial airflow on a smooth surface gives evidence of a very thin boundary layer with the flow occurring in smooth laminations of air sliding smoothly over one another. Therefore, the term for this type of flow is the laminar boundary Layer. Experiments show that this aerodynamic resistance or drag that is present whether or not the aircraft produces lift – unlike induced drag; may be divided into 3 parts: Referring to Figure 17a - As the flow continues back from the leading edge, friction forces in the boundary layer continue to dissipate the energy of the airstream, slowing it down. The laminar boundary layer increases in thickness with increased distance from the wing leading edge. Skin friction Form drag Interference drag Induced drag will be discussed in more detail in the Generation Of Lift and Drag section later in these notes. Some distance back from the leading edge, the laminar flow begins an oscillary disturbance which is unstable. Skin Friction A waviness occurs in the laminar boundary layer which ultimately grows larger and more severe and destroys the smooth laminar flow. This is due to the surface roughness of the aircraft. The velocity of the air decreases as it nears the surface concerned, i.e. the ‘layers’ of air tend to shear due to the internal viscosity of each layer. Its effect is dependent upon: 1. 2. 3.