MN Ch 6 (12th) SLIDE NOTES PDF
Document Details
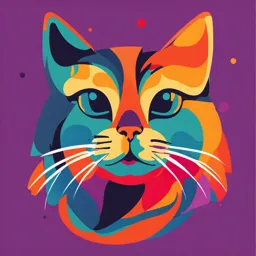
Uploaded by DauntlessSunstone
Tags
Summary
This document provides slide notes for Chapter 6 (Physics) of Egan's Fundamentals of Respiratory Care (12th Edition). It includes discussions of theories, laws, and scientific methods in the context of respiratory care.
Full Transcript
Slide Notes to Chapter 6 (Physics) for Egan’s Fundamentals of Respiratory Care (12th Ed.) Note to Slide 3: Introduction All scientific knowledge is tentative (subject to change) pg. 30-32. Theories are the best explanation we can come up with to explain the phenomena that we observe at our current...
Slide Notes to Chapter 6 (Physics) for Egan’s Fundamentals of Respiratory Care (12th Ed.) Note to Slide 3: Introduction All scientific knowledge is tentative (subject to change) pg. 30-32. Theories are the best explanation we can come up with to explain the phenomena that we observe at our current level of knowledge. • Theories are never proved; they represent the most logical explanation of phenomena based on the evidence available at the time. • Theories become stronger as scientists gather more supporting evidence. • Theories may be modified as new data are gathered or existing data are interpreted in different ways. Theories provide a context for further research and give us a basis for prediction. • For example: In 1916 Albert Einstein published his Theory of Relativity to explain the behavior of objects accelerating at velocities approaching the speed of light. • In 1916 the theory was widely accepted because it explained most of what physicists knew at the time. • Since then, scientists have had to modify the theory several times in order to account for the ever-expanding mass of newly acquired knowledge. Laws are fundamentally different from theories. • Laws can be described as universal generalizations that are based on long-term observations of the natural world. • Examples of laws include the effect of gravity on objects, the relationship of forces and motion, and the nature of planetary movement. • • 1 Scientists must employ rigorous methods while searching for the best explanations of observed phenomena. If they fail to apply rigor, they are practicing junk science. • Scientific explanations must adhere to the rules of evidence. o Evidence Based Medicine has become the standard to evaluate the best clinical management methods. • Scientists must share their evidence with other scientists and make predictions that can be independently confirmed by other researchers. • Explanations must be both logical and consistent with the evidence. o Scientists must look for evidence that their own conclusions are incorrect. o They are not looking for proof that they are correct, they are looking for evidence to disprove their theory. o If there is no evidence to disprove the theory, it is probably correct. o The value of this will become apparent when we look at verifying endotracheal tube placement in Airway Management during RTT 210. 1 of 62 • • Scientific conclusions must be based on the evidence scientists have gathered and evaluated. The current emphasis on Evidence Based Medical Practice attempts to apply the rigorous methods of scientific investigation to the application patient care. • Evidence based practice in an essential element of Respiratory Care. 1 Intellectual rigor means thinking clearly, deeply, and honestly about what we are learning. Honest thinking means skeptically analyzing all the available knowledge on the topic while controlling our biases and preconceptions about it. Resolving inconsistencies means looking for evidence that disproves your own theory. Note to Slide 4: Essential Terms Mass is a fundamental property of matter (all objects are matter): The symbol for mass is usually the lowercase English letter m. Newton defined mass as the quantity of matter that an object contains. o Mass is a numerical measure of the amount of matter (atoms, ions, and molecules) an object contains. In physics, mass is a numerical measure of an object’s inertia, its resistance to being moved (acceleration). Mass does not change with the object’s position, movement, or shape, unless material is added or removed. o An object with mass of 1 kg on earth would have the same mass of 1 kg on the moon. o Because of the moon’s lower gravity, the 1 kg mass would have less weight on the moon than on the earth. • Mass = Density x volume Weight is the effect of the force of gravity (gravitational force) acting on a mass. Weight is the force (acceleration) applied to an object by gravity Weight is calculated by multiplying mass by gravitational force o Mass X Gravitational Force (acceleration). The acceleration (force) of gravity on earth’s surface is about 9.8 meters/second/second (9.8 M/s2). o The speed of a falling object on earth increases about 9.8 meters per second. The SI (International System) unit for force is the Newton. o Earth’s gravity exerts a force of 9.81 Newtons on a mass of 1 kg. Newton's Second Law can be used to express weight as the force of gravity: 2 of 62 The force (F) of gravity (g) equals the mass (m) of the object times the acceleration produced by gravity (g): o Gravitational acceleration on earth is about 9.8 m/second2. The velocity of an object dropped from a building will double for every second it is falling. o Remember that weight = mass x gravity. Water in a cylinder exerts pressure because of water’s weight density plus the acceleration of gravity (see text pg. 92). Density (Text pg. 99) is the ratio of an object’s mass to its volume. • Density can be written with the Greek letter rho (ρ) or the uppercase English letter D • The most common examples of density can be seen in how different materials interact when they are placed together, for example: o Oil floats on top of vinegar in a salad dressing because oil is less dense than vinegar. o Wood floats in water because it has a lower density than water, while a steel anchor sinks because its density is higher than water. o Helium makes a balloon float because the density of the helium is lower than the density of air around the balloon. • Density is the ratio of the mass of a substance to the substance’s volume. o Density is not determined by either weight, mass, or volume alone. • Since density is a ratio, the density of a substance remains constant no matter how much or little (the mass) of the substance is present. The figure at left compares mass and density. o Five objects, five ping pong balls and five golf balls are compared. o Each ping pong ball has a mass of 2 grams. o Each golf ball has a mass of 40 grams. • The mass of a ping pong ball is about 2g, and its volume is about 34 cc (cubic centimeters). • The mass of a golf ball is about 40g, and its volume is about 41cc. o The ping pong ball’s density: D = m/V so 2g/34cc = 0.06 g/cc, o The golf ball’s density: D = m/V so 40g/41cc = 0.98 g/cc. • A golf ball is about 16 times denser than a ping pong ball. 3 of 62 • • • • • • • The Text, pg. 99, says that dense substances have “high atomic weight molecules packed closely together.” o Atomic mass is just the sum of the protons and neutrons of a single atom. o Atomic weight is a little more complicated, but for our purposes we can use atomic mass for atomic weight. The problem with the Text’s statement is that gases also have density, and some gases are denser than others (pg.100). o But we know from the KMT that gas molecules are not “packed closely together.” The density of the air depends on the air’s temperature, pressure and how much water vapor is present. o Air density varies inversely with temperature and altitude (pressure). • The colder the air or the higher the altitude, the lower the density. o Water vapor molecules decrease the density of air by displacing (pushing aside) the higher molecular weight oxygen and nitrogen molecules. o The density of ambient air at sea level is essentially constant at about 1.225 3 kg/m3 or about 0.0012 g/mL (remember that 1 ml = 1 cm or 1 cc). Weight Density (dw) includes the effect of gravity on mass. Weight is often used for mass in clinical equations. o See the gas density calculations in the text (pg. 92, 100 & Box 6-1). Specific Gravity (aka relative density) is a measure that compares the density of a particular substance to water (see Fig. 6-5, pg. 100). - The density of water is 1g/mL, so water has a density of 1 and is used as the standard of comparison. - Objects with specific gravity less than 1 will float in water, materials with specific gravity greater than 1 will sink Units for measuring density: 3 o The FPS (Imperial System) unit for density is pounds per square foot (lb./ft. ). 3 o The SI (International System) unit of density is kilograms per cubic meter (kg/m ). 3 o The CGS (Metric System) unit of density, grams per cubic centimeter (g/cm ) is the measurement unit most often used in clinical practice. • 3 3 Converting kg/m to g/cm simply requires moving the decimal point, for example: o The density of mercury is 13593.0 kilograms per cubic meter (in math the decimal point after the last integer is assumed). o Moving the decimal point 3 places to the left gives 13.593 and rounding up gives 13.56 grams per cubic centimeter (cc). o The density of the air depends on the air’s temperature, pressure and how much water vapor (water molecules) are in the air. • Water vapor in the atmosphere is measured as humidity. 3 o The density of ambient air at sea level is essentially constant at about 1.225 kg/m or, stated in different units, about 0.0012 g/mL 3 o Remember that milliliters equal cubic centimeters (ml = cm ). 4 of 62 • • Air density varies inversely with temperature and altitude. • The warmer the air or the higher the altitude, the lower the air density will be. • The colder the air or the lower the altitude, the higher the density. • Water vapor molecules decrease the density of air because they have less density than the higher molecular weight oxygen and nitrogen molecules. In clinical practice, we usually substitute weight for mass and the weight density (dW) of a material is what is actually being measured (Text, pg. 92, Pressure in Liquids). o Dw is the ratio of weight to volume (weight/volume). For practice, calculate the density of a certain mass (weight) of a substance: • 6.54 milliliters (a volume) of the substance weighs 89 grams. 𝐦 • D = 𝐕 89 g / 6.54 mL = 13.60 g/mL • • • Each mL of the substance weighs 13.6 grams. • By the way, the substance in this example is mercury (Hg). Note that the / symbol can either mean divide (÷) OR it can mean for each or for every, as in 13.6 g for each mL of the substance. Now use different numbers and try the equation again! A clinical example of the relevance of measuring relative density: The term specific gravity is frequently used clinically to express the relative density of a substance compared to the density of water. • Specific gravity is measured with a hydrometer (Text, pg. 93, Fig. 6.5). 𝐦 Density = mass ÷ volume, so D = 𝐕 • • Volume is the three-dimensional space occupied by a substance or enclosed by a surface Volume can be written with uppercase English letter V. • • The International System of Units (SI) standard unit of volume is the cubic meter (m ). The metric system uses the liter (L) as a volume unit. • o One liter has the same volume as a 10-centimeter cube (10 cm ). 𝐦 Volume = mass ÷ density V = 𝝆 (remember, the Greek letter rho, 𝝆, means density). 3 3 Comparing Volume, Mass, and Density They are not the same thing. o Volume is the amount of three dimensional space occupied by a substance, o Mass is the amount of matter in the substance occupying the space. o Density is the amount of the substance mass per unit of volume. 5 of 62 • • • Comparing Volume and Capacity: Capacity is the interior volume (size) of the vessel that holds the liquid, gas. or any other material that takes the shape of its container. o See the Handout on Measurement of Lung Volumes and Capacities. Capacity is not automatically the same as volume. o A container may have a capacity of 100 milliliters but may only be half full, so while capacity = 100 mL content is only 50 mL. o This fact will be important when we calculate things like: • How long a gas cylinder will deliver gas, • How much oxygen a patient is getting from a nasal cannula • The absolute and relative humidity of a gas delivered to a patient. Units of capacity relevant to respiratory care include the milliliter, liter, and gallon. Measuring the Volume of Liquids, Solids, and Gases • Because gases completely fill their containers (cylinders), the volume of gas in the cylinder is the same as the internal volume of the cylinder. o This relationship is useful when we want to measure a patient’s lung capacity. • Gas cylinder contents are usually measured in units of volume, such as cubic 3 • • • centimeters, cm , or cubic liters. Liquids are commonly measured using containers, where the volume is marked on the container. o Examples of instruments used to measure liquid volume include measuring cups, graduated cylinders, flasks, and beakers. Mathematical formulas exist for calculating the volume of regular solid shapes. Another method of determining the volume of a solid is to measure how much liquid the solid displaces (Text pg. 92, Archimedes Principle). • Liquid is being poured from a beaker into a flask. • The liquid is enclosed within the threedimensional flask. • Notice that the liquid is a fluid, so it flows, gases are also fluids that can flow. o While liquids also take the shape of the container, o Gases completely fill the container. • If the size (volume) of the container is unknown, we can use the amount of fluid we put into it to measure the container’s volume. o Measuring the volume of gas inhaled by the patient is a technique used to measure lung volume in the Pulmonary Function Laboratory. 6 of 62 • Clinical Practice Note Medical gases, principally oxygen, are often administered form metal cylinders. o Gas cylinders often have slightly different specifications because they are made by many different manufactures. o Exact sizes, contents, and working pressures of gas cylinders will also vary among cylinder manufactures and compressed gas suppliers o While cylinders may be made from a variety of different materials, medical gas cylinders are usually made of steel so they are rigid and can’t change size or shape. Work: Thermodynamics defines work as: transferring energy to or from a system. Mechanics defines it as: force times distance as object is moved. Pulmonary Physiology defines work as: pressure times volume. Work requires three elements: 1. Force: must be applied to the object. 2. Displacement: the object must be moved. 3. Cause: the force applied must be the cause of the movement. Breathing is a form of work: o The respiratory muscles generate force, in the form of pressure, o The pressure is needed to overcome the resistances of the airways and the chest wall. o The result will be a flow of air into and out of the lungs. Normally, the work of breathing (WOB) is so low that we don’t even realize we are doing any work. In lung disease WOB can be so high that the patients’ muscles can’t generate enough pressure to breathe. Note to Slide 5: The Classic States and (Phases) of Matter Intermolecular forces of attraction (IMF): • A chemical bond is the physical process that causes atoms and molecules to be attracted to each other and held together in more stable chemical compounds - When atoms bond, electrons are either shared or exchanged • At least five types of bonds hold atoms together within molecules: - Ionic, covalent, polar covalent and metallic. - Ionic bonds are electrostatic attractions between ions of opposite charge. - Ionic compounds (e.g. sodium chloride) are held together by ionic bonds. - Ionic solids have high melting and boiling points, are brittle in nature, have a lattice structure and are able to conduct electricity when in solution (making them electrolytes). - Covalent bonds involve two atoms equally sharing electrons between them (co means together, and valent refers to the valence shell of the atom). - The peptide bonds that hold DNA strands together are example of nonpolar covalent bonds. 7 of 62 - - Polar covalent bonds also involve sharing electrons between two atoms, but the electrons are shared unequally. - Water molecules are made up of two hydrogen atoms and bound to one oxygen atom held together by polar covalent bonds (often called hydrogen bonds). - Liquid water molecules are attracted to each other by electrostatic forces called van der Waals forces or van der Waals bonds. - These bonds produce the cohesion between water molecules that contributes to water’s viscosity and surface tension (Slide 53). Metallic bonds result from the electrostatic attraction between the positively charged nuclei of metal atoms and the electrons in the metal. - Metals can conduct heat and electricity, they have a metallic shine, they are both malleable (flexible) and ductile (stretchable) and they have a high melting point and density. • The oxygen end of the water molecule (H2O) has a slight negative (-) charge, and the hydrogen end has a slight positive (+) charge. • The + hydrogens of one water molecule are loosely attracted to the – oxygen of another water molecule. • The bond is weak, so a slight increase in kinetic energy will allow the liquid molecules to break apart and change state to water vapor. Plasma: • Plasmas, which the text calls a “fourth state of matter,” are clouds of charged sub-atomic particles. Plasmas are diagramed on Text pg. 88, Figure 6-1 D. • These extremely high temperature particle clouds have nothing to do with the liquid component of blood. o Plasmas produce the northern lights (Aurora Borealis), they are used in neon signs and in industrial welding applications. • While plasmas have applications in science and industry, they are completely irrelevant to medical practice and respiratory care. o Cosmic plasmas are included in the Text for informational purposes only. Most materials can be classified either as solids, liquids, or gases. The distinction between these phases deals with the strength of the forces of attraction between the atoms, ions, or molecules (constituents) that make up the material. 8 of 62 • • • • • Solids have strong intermolecular forces that hold the constituents together. The atoms or molecules have very little freedom to move, and they are arranged so closely that the material is virtually incompressible. Crystalline solids have a definite, rigid shape, examples include: • Sodium chloride, sugar, diamonds, etc. Amorphous solids are made of molecules whose positions are not rigidly ordered examples include: • Window glass, rubber, plastic, cotton candy, etc. Liquids have somewhat weaker bonds between the molecules. • The molecules can move past one another freely, so the liquid will be able to flow, and liquids will tend to take the shape of their container. Gases have little or no intermolecular attraction between the molecules. • The distance between adjacent molecules is many times larger than the distance between adjacent molecules in liquids and solids. • Gases generally diffuse evenly throughout the container. Since both liquids and gases can flow, they are both classified as fluids. • • Respiratory care is primarily concerned with the behavior of gases. RCP are also concerned with change of state, particularly evaporation and condensation of water. To help improve understanding of states of matter, look at some interesting materials: Quartz, Glass, and Water. Inspect the molecular structures • Quartz is a crystalline solid: • Each quartz molecule occupies a definite position in a perfectly ordered three-dimensional lattice. • Strong attractive forces hold quartz molecules rigidly in position. • Glass is an amorphous solid. • It has the mechanical rigidity of crystalline solids, but it also has the random, disordered molecular arrangement like liquids. • Like a liquid, glass flows but at an incredibly slow rate. • Since it flows, all glass will eventually fail. 9 of 62 Water is a unique substance: For most substances, the molecules move closer together when they get colder. This makes the substance more dense (bottom figure). Like most other materials, when water molecules get warmer, they also move further apart as their bonds weaken (middle figure). When water molecules reach the freezing point, the molecules move even further apart and form a stable, crystalline matrix (top figure). This anomalous characteristic of water explains why ice floats on water. It also explains why the water level does not rise as the ice cubes in a glass melt. Note to Slide 8: Energy & the Laws of Thermodynamics The concepts of thermodynamics apply to cardiopulmonary physiology when we consider work. • Physics texts can’t define energy, so they cop out by describing a characteristic of energy as: The ability to do work. • Then they describe the two major forms of energy: – Kinetic energy, the energy of movement, and – Potential energy, the energy of position or stored energy, as in Pel from the • equation of motion (Text, pg. 226). Work can be defined as the application of energy to matter to overcome resistance and cause movement i.e., flow. – The work of breathing (WOB) is the amount of energy, in the form of pressure that the respiratory muscles need to overcome airway, lung, and chest wall resistances in order to move air in and out of the lungs. 10 of 62 • • During inhalation, the respiratory muscles generate kinetic energy in the form of a pressure change in the thorax to draw air into the lungs. – As the elastic alveoli and chest wall structures expand during inhalation, some of that kinetic energy is stored as potential energy. During exhalation, the stored elastic energy is released and pressure in the alveoli increases to push the air out of the lungs. For Those Interested: A very basic explanation of the science of thermodynamics When heat (which is another term for kinetic energy) goes into a substance one of two things can happen: 1. The substance’s temperature can increase. • The added energy (heat) increases the average kinetic energy of the substance’s molecules. • This increase in average kinetic energy is measured as a number, temperature (in the various temperature scales). 2. The substance’s state can change, for example: • If the substance is ice, it can melt into water. • The change doesn’t cause a rise in temperature because the average kinetic energy of the ice molecules is the same as the average kinetic energy of the water molecules at the instant before melting and at the instant after melting. Terms: Thermodynamics textbooks don’t seem able to adequately define the terms listed below. These “definitions” are the best that my research can come up with. • Temperature is a number that is related to the average kinetic energy of the molecules of a substance. o The number that we call temperature is related to energy, but it is not energy itself. o That definition does not say that temperature is the same as kinetic energy, o Nor does the definition state exactly what the relation between temperature and kinetic energy is. o Because physics does not actually know exactly what energy is. o The capacity to do work is a characteristic of energy, not a definition. o The best physics can say is that although temperature is not energy, it is a number proportional to the type of energy called kinetic energy. • Heat is actual energy measured in Joules, calories, BTU, or other energy units. o Heat is a measurement of some of the energy in a substance. o When heat is added to a substance, energy is added to the substance. 11 of 62 o This added heat (energy) is usually expressed as an increase in the kinetic energies of the molecules of the substance. • Work is done on an object if energy (heat in thermodynamics) is applied and the average kinetic energy of the object’s molecules changes. • Sensible heat is the energy required to change the temperature (which can be sensed) of a material without changing the material’s state. o Specific heat: is the amount of heat energy needed to raise the temperature o (sensible heat) of one gram of a material 1 C. o The specific heat of water is about 10 cal/kg. o The specific heat of CO2 is about 2 cal/kg • Latent heat is the energy absorbed by or released from a substance during a change of state (from gas-to-liquid-or-solid or vice versa). o In an endothermic phase change energy is absorbed by the material and used to break the intermolecular bonds. o In an exothermic phase change energy is released from the material and the molecular bonds are strengthened. Heat Energy Units: The most common units used to measure heat energy are Joules (J), Calories (Cal.), and British Thermal Units (BTU). • Joules (J) are the SI units for energy: o One Joule equals about 0.2388 (≈ 0.239) calories (cal). o One J equals about 0.000948 BTU. • Calories (Cal) are often used for heat energy, especially in physiology. o One calorie equals about 4.186 Joules (J). • (To convert calories to Joules, multiply by 4.18) (To convert Joules to calories, divide by 4.18) o One calorie equals about 0.003968 BTU. BTU: British Thermal Units: o One BTU equals 1055 Joules (1.055 kilo Joules (kJ). o One BTU equals about 252.0 calories. A more sophisticated explanation: The relationship between the concepts of heat and energy is still debated in science, so there is no widespread agreement about exactly what the term heat means and how it should be used. That is why textbook writers tap dance around a definition instead of actually giving one. One study (cited in Wikipedia) found that several popular textbooks used language that implied at least three different three meanings for heat: 12 of 62 1. That heat is the actual energy that is transferred (as if heat were a substance). 2. That heat is the process of transferring energy 3. That heat is an entity (a thing) contained within a physical system. (Note that the first two senses of the term define heat in terms of energy) Most physicists use the first sense of the term heat; as if it were a substance, and that is how I used it on Slide 9. This use implies that heat is a form of energy that is transferred between the matter and its environment when a change of state occurs. By the way, that is how heat transfer in a steam autoclave is described in most infection control textbooks. o In 2004, the physicist Friedrich Herrmann stated that we are confused because the concept of heat can’t be adequately explained in terms of energy. o Herrmann wrote that heat is best understood as entropy. o Entropy describes the tendency of nature to eliminate all gradients and distribute the total amount of mass and energy evenly throughout the universe. • When there are no gradients, there will be no movement. o Another term for entropy is disorder, and it can be thought of both in physics and biology: o Life requires an orderly arrangement of atoms, molecules, cells, tissues, etc. o Establishing and maintaining that order requires energy, energy gradients, and movement of both energy and matter. • See the note on muscle physiology and ATP production in the Chapter 9 Slides. Some Additional Terms: Classical thermodynamics is an “observational science” that describes macroscopic quantities, such as heat, work, internal energy, enthalpy, and entropy. The four “Laws” of thermodynamics are not laws, they are principles derived from observation over many years. They are called laws because there is no evidence that they that have ever been violated in nature. Quantum mechanics deals with nanoscopic (molecular, atomic and sub-atomic) properties of matter. The equation developed by Schrodinger is the basis of quantum mechanics. Most of the concepts of modern chemistry are based on quantum mechanics theory. The diagram of water molecules on Slide 26 is based on the Schrodinger equation‘s model for single molecules. Quantum mechanics provides information on the electronic structure (how atoms are bonded together and how electrons are shared to make up chemical bonds) and the geometrical structure of molecules. Statistical mechanics was developed in the late 19 century (1800s) by the American scientist J. Willard Gibbs. Statistical mechanics takes the physical properties, energy levels, and probabilities of individual molecules from quantum mechanics and average them in an appropriate way to predict the properties of macroscopic collections of molecules. th 13 of 62 Note to Slide 10: Evaporation, Condensation, & Boiling • In the liquid state (left) hydrogen bonds hold the water molecules together in a visible mass. • The bonds continually break and reform. o See animation on Slide 5. • In the vapor state (right), kinetic energy i.e., heat, has broken the hydrogen bonds that hold water molecules to each other. • The individual molecules now float around in the air and behave like a gas. o See animation on Slide 5. In everyday usage, most people use the terms vapor and gas interchangeably. o This is confusing because the terms have different meanings Gas is a state of matter where individual molecules move around at high speed, in accordance with the kinetic molecular theory of gases (KMT). o Individual molecules are too small to be seen by the naked eye, so gases and vapors are invisible. Vapor is a state of equilibrium between the liquid and gaseous state of the substance. o Don’t confuse vapors with dust (a fine suspension of solid particles in the air) or fog or mist, (a fine suspension of liquid droplets in the air). Confused terminology is not a problem as long as RCPs understand the difference: More differences between a vapor and gas: o A gas exerts pressure according to its fractional concentration (see Dalton’s law of partial pressure). o Water vapor is the term for water molecules (not particles) suspended in a gas o Water vapor exerts pressure in direct proportion to the temperature of the gas that contains the water vapor molecules e.g., water vapor in alveolar gas. Water vapor pressure increases exponentially with increases in gas temperature (Fig. 6-12). Vapor Pressure is the force per unit area exerted by a vapor that is in equilibrium with the pressure and temperature of its surrounding atmosphere i.e., alveolar gas. o Since vapor pressure is also a measure of a substance's tendency to evaporate it is used as a measure of chemical volatility. o A liquid that vaporizes at room temperature is a volatile liquid like gasoline or alcohol. While Evaporation & Condensation are State Changes, they are also Thermodynamic Processes involved in Heat Transfer (see Figures on Slide 13) Evaporation and Condensation are exactly opposite physical mechanisms. 14 of 62 • • Liquid water and other substances in the liquid state can undergo a phase change to the vapor state through the process of one of the two forms of vaporization called evaporation, the other form of vaporization is boiling (Text, pg. 95). o Evaporation is the form of vaporization that occurs at a temperature below the liquid’s boiling point. o Liquids evaporate when the kinetic (heat) energy of their molecules is high enough to break the hydrogen bonds that hold the molecules together. o The First Law of Thermodynamics tells us that evaporation requires heat to increase the liquid’s kinetic energy. o That heat energy comes from the surrounding air next to the water surface. o As the water evaporates the surrounding air loses heat energy and cools. o The best example of this is how evaporating perspiration cools the skin. Vapors can return to the liquid or even the solid state by the process of condensation. o As water molecules loose heat energy their kinetic activity decreases, and the weak hydrogen bonds reconnect vapor molecules back to liquid. o As the water condenses the surrounding air gains heat energy and is warmed. o Examples of this include the inside of a car’s windshield “fogging up” in the winter and the sudden increase in air temperature after a quick summer rainstorm. o Exhaled air is almost fully saturated with water vapor at body temperature o (37 C). o When the exhaled air hits the cold windshield, the temperature suddenly drops and liquid water forms on the glass. o When the rain stops and the sun comes out, water on the ground evaporates adding heat energy to the surrounding air. Vaporization happens in two different ways: evaporation and boiling. 1. Evaporation: is the endothermic process (see Figs. on Slide 15) process by which the polar bonds holding water molecules together at the liquid surface break apart. This allows individual water molecules to enter the gas phase above the liquid. The reaction is endothermic because the energy for evaporation must come from the surroundings, for example: evaporating perspiration takes heat energy from skin, so the skin is cooled. As high kinetic energy molecules leave the liquid the average kinetic energy of the liquid drops and it gets cooler. Evaporation occurs at any temperature, but the rate of evaporation is directly proportional to the temperature. 2. Boiling: is the rapid vaporization of a liquid that occurs when a liquid is heated to its boiling point (the temperature where a liquid’s vapor pressure equals the pressure of the gas above the liquid). Visible bubbles of vaporized liquid (the gas phase) form within the bulk of the liquid. Boiling is a bulk i.e., the entire mass of the liquid, rather than a surface phenomenon like surface tension. Vapor bubbles start at the bottom of the pot and rise to the surfaced where they burst and release vapor molecules into the air. In boiling the temperature remains constant at the boiling point. A liquid can be made to boil at low temperatures by placing it in a vacuum chamber or by taking it to a high altitude where the atmospheric pressure is lower. 15 of 62 This explains why some cake recipes call for longer cooking times at high altitudes. 3. Condensation, the opposite of evaporation, is the exothermic process (see Figs. on Slide 15) by which water vapor molecules turn back into liquid water. The KMT tells us that vapor (gas) molecules have high kinetic energy and no intermolecular attraction. If water vapor molecules encounter a cool surface they slow down, and their polar attractive forces overcome their kinetic energy. In other words, the individual vapor molecules reconnect and become liquid water molecules. The reaction in exothermic because excess molecular energy can’t just disappear, so it is released into the surroundings as heat. Saturation is the maximum amount of water vapor molecules that a volume of air can hold at any particular air temperature. When this limit is reached, the air is said to be saturated at that temperature. When air is saturated, the relative humidity will be 100 percent and no more water vapor molecules can be added unless the air temperature increases. 4. Dew Point is an important related concept: The dew point is the gas temperature at which condensation (in the atmosphere condensation is called precipitation) occurs. Terms related to the physical concept of Saturation: • In gas physics, a volume of gas is saturated when it cannot hold any additional water vapor molecules unless the gas temperature is increased (Fig.6-13). In chemistry, a solution is saturated when there is no more space in the solution’s solvent for any additional solute. o A Saturated Liquid contains as much thermal energy as it can hold without boiling, add just a little more energy (make it warmer) and the liquid will boil. o A Saturated vapor contains as little thermal energy as it can hold without condensing, take away just a little more heat energy (make it cooler) and the vapor will condense back to the liquid state. o The Saturation temperature of a liquid is another term for the liquid’s boiling point. The Saturation pressure is the pressure for a corresponding saturation temperature at which a liquid boils into its vapor phase. Super-saturation is another term that can be very confusing. o Fortunately, super-saturation is not a term commonly encountered in pulmonary medicine. A supersaturated solution has had outside pressure forces applied to it. o These outside forces allow for a higher concentration of a solute or gas in the liquid than would be possible if no outside pressure was applied. o The carbonated water in soda is a good example of a supersaturated solution. – The outside force in this case is the increased pressure inside the bottle. – The pressure allows more carbon dioxide to dissolve in the liquid than would be possible at ambient pressure (see Henry’s law of Solubility). – When the bottle is opened, the soda is subjected to atmospheric pressure and the excess carbon dioxide gas slowly from the supersaturated liquid. 16 of 62 – Another example is the scuba diver who rises (ascends) to the surface too quickly. Here, the outside force is the water pressure on the diver’s body at depth. The water pressure allows more nitrogen to dissolve in the blood. Too rapid ascent quickly lowers the pressure on the diver’s body and allows the dissolved N2 to form gas bubbles in the blood vessels. Thermodynamics Note Water vapor and steam are different. While water vapor is usually called the gaseous form of water, it is not really a gas, because it does not actually follow Dalton’s law. Steam is produced when water is heated to a temperature greater than its boiling point. o This can only happen when the gas pressure above the water is greater than ambient, such as in a pressure cooker or in a hospital autoclave. The cap on a boiling tea kettle allows enough pressure to develop above the water for the water to flash to high temperature steam (Fig. 6.3). There appears to be nothing in the space between the spout and the cloud of water vapor. o That’s because the space contains individual molecules of pressurized water gas (steam) which can’t be seen. The steam in that space much hotter than 100oC. If you stick your finger into that space the steam will release its latent heat energy when it condenses on your cooler finger. DON’T TRY IT • Two Additional Thermodynamic Concepts: Sublimation and Deposition: 1. Sublimation (in physics) is the change from the solid to the gaseous or vapor phase without passing through an intermediate liquid stage. • An example occurs when the volume of snow on the ground decreases without melting. • The snow has sublimated into water vapor in the air without first melting into water. 2. Deposition (in physics) is the opposite of sublimation. • It occurs when vapor solidifies without passing through the liquid phase. • Deposition is seen when water vapor turns directly into ice (frost) on a cold windshield or when water vapor in the air turns into snowflakes or hailstones. A very good site for looking at phase changes and energy: 17 of 62 http://phet.colorado.edu/en/simulation/states-of-matter Note to Slide 11: Temperature Scales • To convert degrees Fahrenheit to degrees Celsius: • The Fahrenheit to Celsius and the Celsius to Fahrenheit conversion formulas on pg.90 use 1.8 in the conversion. • The 1.8 factor represents the difference in the range of the Fahrenheit and Celsius scales. • Fahrenheit is a fractional scale while Celsius is a decimal scale. • In the Fahrenheit scale water freezes at 32 degrees and it boils at 212 degrees. • This means that there are 180 Fahrenheit divisions between freezing and boiling (212 - 32 = 180). • In the Celsius scale water freezes at zero degrees and boils at 100 degrees. • This means that there are 100 Celsius divisions between freezing and boiling. • The relationship between the two scales can therefore be expressed as 180/100 =1.8. • The conversion factor 9/5 (nine fifths) is just the fractional conversion of the decimal 1.8. • Whether you use 9/5 or 1.8 depends on which you prefer to use: fractions or decimals. Historical Note A thermometer is an instrument that measures the temperature of a system in a quantitative i.e., numerical way. In 1724, Gabriel Fahrenheit, an instrument maker in Amsterdam, Holland, used mercury as the thermometric liquid. • Fahrenheit measured the boiling point of water as 212 and he adjusted the freezing point of water to 32 so that the interval between the boiling and freezing points of water could be represented by the more rational number 180. • Temperatures measured on this scale are designated as degrees Fahrenheit (° F). • In 1745, Carolus Linnaeus of Uppsala, Sweden, described a scale in which the freezing point of water was zero and the boiling point 100. • This made a centigrade (one hundred steps) scale. • Anders Celsius (1701-1744) Celsius was accustomed to using the thermometer made by the French astronomer Joseph-Nicolas Delisle. • Delisle’s thermometer used zero as the boiling point, thus creating a reversed scale with increasing numbers for decreasing temperatures. • Celsius’ scale also has 100 steps (degrees) between the two defining points. • In 1948 the International Standards Organization dropped the Linnaeus’s Centigrade scale in favor of Celsius’ scale. • In clinical practice we measure temperature in degrees Celsius (° C). • Clinicians often use the term degrees centigrade. • In 1787, French physicist Jacques Charles observed that the volume of a gas under a constant pressure increases or decreases with temperature. • This finding (Charles’ Law) was refined around 1808 by another French scientist, Joseph Gay-Lussac. 18 of 62 Note to Slide 12: Absolute Zero (Kelvin Scale) Converting temperatures from one scale to another: • To convert degrees Celsius (Centigrade) to Kelvin: o • K = C + 273. o • C = K – 273. • There is NO degree symbol used in the Kelvin scale. • In most calculations (such as the gas laws) the 0.15 is ignored. Note to Slide13: Change of State: Liquid Solid State Change Change of State from: Name of the State Change1: Heat Energy is: Enthalpy2 (H): Enthalpy ∆ (H∆) Solid to Liquid Melting (or) Fusion Absorbed Latent Heat of Fusion + Liquid to Solid Solidification (or) Freezing Released Latent Heat of Fusion - Liquid to Gas Vaporization (or) Boiling (or) Evaporation Adsorbed Latent Heat of Vaporization + Gas to Liquid Condensation or Liquefaction Released Latent Heat of Vaporization - Solid to Gas Sublimation Absorbed Latent Heat of Sublimation + Gas to Solid Condensation or Deposition Released Latent Heat of Sublimation - 1 The terms Fusion, Vaporization, and Sublimation are used correctly. Pay attention to the enthalpy ∆ (H∆) + and – signs that indicate whether energy is being released (exothermic) or absorbed (endothermic). 2 Enthalpy is a measure of heat in a thermodynamic system. They use the formula H = U + PV. H is the enthalpy value, U is the amount of internal energy, and P and V are pressure and volume of the system. Change in enthalpy is used to measure heat flow in calorimetry. It also works well for gases. • Sensible heat is the temperature of a substance caused by the average kinetic energy of the material’s molecules when there is no change in the material’s state (solid, liquid, or gas). • Latent heat is the amount of heat energy that is absorbed or released by a material during a change of state. • A change of state changes the internal arrangement of the material’s molecules but there is no change in the kinetic energy of the molecules, so the temperature (sensible heat) doesn’t change. 19 of 62 • • • • A higher level of internal energy requires absorption of thermal energy from the surroundings (called an endothermic process). • A lower level of internal energy requires a release thermal energy into the surroundings (called an exothermic process). Melting: the latent heat of fusion is the amount of energy that must be added to a substance to change its state from solid to liquid without changing its temperature. Freezing: the latent heat of solidification is the amount of energy that must be removed from a substance to change it from a liquid into a solid. Vaporization: the latent heat of vaporization is the amount of energy that must be added to a substance to change its state from a boiling liquid to a gas without changing its temperature. • The bubbles that appear in boiling water are made as water molecules in the hottest water (near the bottom of the pot) vaporize. Latent Heat Example: • • • • • • • • 0 0 Steam at 100 C causes more serious burns then are caused by boiling water at 100 C. The reason is latent heat. About 1,000 British Thermal Units (BTU) of energy must be added to each kilogram (kg) to vaporize boiling water to steam. 0 When steam condenses back into water at 100 C, it releases all of this latent heat energy. This extra energy in the latent heat is what causes more severe burns. Obviously, to produce steam, the water must be held at a pressure above atmospheric (760 mmHg at sea level). That is how home pressure cookers and hospital autoclaves work. Latent heat energy is released when steam condenses on the cooler instruments is how hospital autoclaves work. Note to Slide 14: Temperature and Pressure: Effect on Phase The Mini-Clini on Text, pg. 104 uses the terms isothermal, Joule-Thompson effect, and adiabatic process. The author completely fails to explain the terms, so I will try: 1. First: there are three types of thermodynamic systems: Open, Closed, and Isolated 20 of 62 • An open system (1st figure) can exchange both energy and matter with the surroundings. • A closed system (2nd figure) can only exchange energy, not matter, with the surroundings. • An isolated system (3rd Figure) does not exchange either energy or matter with the surroundings. • A living creature is an example of an open thermodynamic system. 2. Isothermal process (“iso” is a word root that means equal): •In an isothermal process heat energy is transferred from a system to its surroundings but the temperature remains constant. • A good example is a pot of boiling water: As the water boils, heat energy is transferred to the room (the surroundings), but the temperature of the water remains constant at the boiling point (110oC). 3. Joule-Thompson effect: • When a compressed gas is released from a cylinder the molecules expand rapidly. • Rapid expansion increases the space between the molecules, reduces kinetic energy, and causes the gas temperature to drop. Several of the gas laws explain this. The Joule-Thompson effect explains how refrigerators and air conditioners work. The whimsical figure at left shows Drs. Joule (left) and Thompson (right) superimposed on a compressed gas compressed gas pipeline. • A large red valve controls flow through the pipe. Dr. Joule’s side has a pressure of 900 PSI and a temperature of 80oF. When the valve is opened, the gas rapidly expands into Dr. Thompson’s side. Dr. Thompson’s pressure quickly drops to 200 PSI and the temperature drops to 30oF. 21 of 62 4. Adiabatic Process (from the GR word adiabatos "not to be passed”). Adiabatic processes occur when energy is transferred into or out of a system by work but there is no change in temperature. • Work can be defined as adding or removing energy from an object. • An example of an adiabatic process, as mentioned in the Mini Clini. • A well-insulated cylinder contains a volume of gas. • When the piston (arrows) is pushed down or pulled up the gas is compressed or expanded (rarefied). • The insulation prevents heat from being transferred into or out of the cylinder. Note to Slide 16: Properties of Liquids: Introduction • In physics, a fluid is defined as a material continuum that is unable to withstand a static shear stress (also called shear force). • Unlike an elastic solid (like a rubber band) which responds to shear stress with a recoverable deformation (it snaps back). • A fluid responds with an irrecoverable flow (you can’t push the ketchup back into the bottle). • Shear stress is the term for forces that tend to cause deformation of a material by slippage (movement) along a plane or planes parallel to the imposed stress. o (Fig. 6.8) shows shear stress separating the streamlines in column of gas moving through a tube. • Shear stress also occurs in layers of tissue including airways and blood vessels. An everyday example occurs when a bolt is over torqued (tightened) with a wrench. o The tightening motion applies a shear stress to the bolt. o If the force is sufficiently high, the head of the bolt will be “sheared off.” Blood flowing through blood vessels applies a shear force to the endothelial lining of the vessel. o In nature shear forces are related to landslides and avalanches as well as to earthquakes. Note to Slide 17: Liquid Pressure 22 of 62 3 Water density is 1g/cm but the PL formula in the text gives an answer in cm 2 The explanation is that water density is exerted in three dimensions (height, width, and depth) while water pressure is exerted on a two-dimensional unit area (A) at the bottom of the column. The classic form of the equation is P = pVg/A • P = Hydrostatic pressure. • p = Density of the liquid (p = m/v). Remember, p is the Greek letter rho. • V = Volume of the liquid (V = h x A) 2 2 • g = Acceleration of gravity is 9.80665 m/s (32.174 ft/s ). Notes on the weight of gas and water: The weight of all the molecules of gas in the atmosphere exerts about 14.7 pounds of pressure on every square inch (PSI) of the earth’s surface at sea level. • This atmospheric pressure is reported as: 1 ATM, 14.7 PSI, 1,013.2 mb, or 101,325 kPa. • Creatures who live on the surface of the planet don’t feel the pressure because their bodies exert an equal outward pressure. Water is much denser than air (33 feet of sea water has the same density as 33.9 feet of fresh water) • 33 feet of sea water exerts 14.7 PSI, the same pressure per square inch as the entire atmosphere. • Every 33 feet below the sea surface adds another ATM of pressure. – A scuba diver 33 feet below the surface experiences a pressure of 2 ATM (29.4 PSI). – The pressure of the gaseous atmosphere pushing down on the water must be added. • At 66 feet the pressure is 3 ATM or 44.1 PSI. • At 99 feet the pressure is 4 ATM or 58.8 PSI. • • • • • Pressure vs Force: Pressure and force are closely related concepts. • But just like heat and temperature, they are not the same. Pressure is the amount of force, applied on a surface per unit area: P =F /A. It is the force acting perpendicular to the surface of the object, causing the force to spread over a certain area. Therefore, pressure considers the area over which the force is exerted: • When force extended to a large area, the pressure is low. • If the same force is stretched to a small area, the resulting pressure would be high. Force is a push or a pull, caused when two objects interact to change the state of rest or motion of the object. Force is a vector expression because it has both magnitude and direction. Magnitude is the quantity of a force, i.e. the larger the magnitude, the more force applied, and vice versa. In this vector the magnitude is shown by the diagonal line. 23 of 62 Direction in a circular vector diagram is the counterclockwise angle the magnitude line makes with the positive x-axis of the graph. In this vector the direction is northeast. The graphics displayed on a mechanical ventilator screen are scalars because they only show magnitude. Scalars don’t have direction; they are only moving forward in time. 3 Water density is 1g/cm but the PL formula in the text gives an answer in cm 2 The explanation is that water density is exerted in three dimensions (height, width, and depth) while pressure is exerted on a two-dimensional unit area (A) at the bottom of the column. The classic form of the equation is P = pVg/A • • • • • • • P = Hydrostatic pressure. p = Density of the liquid (p = m/v) (remember, p is the Greek letter rho). V = Volume of the liquid (V = h x A) 2 2 g = Acceleration of gravity is 9.80665 m/s (32.174 ft/s ). Notes on pressure measurement across the SI, CGS, and FPS systems: 2 The SI unit of pressure is the N/m (newton per square meter). 2 • N/m is commonly called the Pascal (Pa) (Medical Terminology Slide 57). The CGS unit of pressure is dynes/cm. 2 The FPS unit of pressure is pounds/in . The Newton is defined as “the force required to accelerate a mass of one kilogram by one meter per second squared.” This has clinical relevance because some journal articles talk about pressing on a part of the body with a force of X Newtons. It helps to know how much force is being called for. Notes on Pascal’s Principle: (pg. 93, Fig. 6.4) Pascal’s Principle states that: “A change in pressure applied to an enclosed fluid is transmitted undiminished (equally) to every point of the fluid and to the walls of the container”. • The classic examples of the principle are Pascal’s Vases and the hydraulic car lift in a garage. • The text uses Pascal only to illustrate that pressure of any liquid depends only on the depth, and not on the volume or shape of the container. 24 of 62 The figure at left is another example of Pascal’s Principle. When external pressure is applied to a confined fluid the pressure is distributed equally throughout the fluid. If a water filled syringe is connected to a perforated blub, water will flow out of the perforations equally when the syringe plunger is depressed. This is the principle of the hydraulic jacks and car lifts found in a mechanic’s garage. • • Does Pascal’s Principle have any relevance to human physiology? It is usually a bad idea to try to apply many of the basic concepts of physics and engineering to complex biological structures. You will see many examples such confusing misuse in the literature, including the text. o There are too many variables involved for an accurate answer to be obtained: • The human body does not behave like a bag full of water. • The body is covered with skin that stretches with different rates of elasticity in different directions, • The lungs are full of air which obeys Boyle's law not Pascal's Principle, • The heart and vasculature work to create pressure differentials throughout the circulatory system, • Membranes in the kidneys and digestive track change osmotic pressures to filter materials out of the blood, • Note to Slide 18: • • Finally, bones, tendons, and connective tissues are fairly solid. Buoyancy Buoyancy (aka the buoyant force) is the vector force exerted on an object that is wholly or partly immersed in a fluid. • The symbol for the magnitude of buoyancy is B or FB • Since buoyancy is a vector quantity it must be expressed with units of both magnitude and direction. • For our usual day-to-day experiences, buoyancy acts in an upward. direction • The SI unit of buoyancy is the newton [N]. Buoyancy is caused by differences in pressure acting on opposite sides of an object immersed in a static fluid. • A typical example: • The pressure on the bottom of a submerged submarine is greater than the pressure on the top because static fluid pressure increases with depth (dw). 25 of 62 The force on the bottom pushes up and the force on the top pushes down. • But since the bottom of the submarine is deeper in the water than the top, the direction of the net force due to the water is upward. Pressure variations in a fluid are typically caused by gravity, but in general buoyant forces act opposite the direction of the gravitational acceleration. • • • Archimedes' Principle • The magnitude of the buoyant force on an object is equal to the weight of the fluid the object displaces. B = dw x Vdisplaced The factors that affect buoyancy are: • the density of the fluid. • the volume of the fluid displaced. • the local acceleration due to gravity. • The buoyant force is not affected by either: • the mass of the immersed object, or • the density of the immersed object. If you are interested, here is an 8-minute video by Eric Strong, MD about buoyancy and medicine. • https://www.youtube.com/watch?v=8V32tdkxgNw • • Note to Slide 19: Viscosity • Viscosity is an intrinsic property of fluids (both liquids and gases). • The property can be thought of as the internal friction between adjacent fluid layers as they slide past each other. • This internal friction contributes to the total resistance to flow that the pressure driving the fluid has to overcome. • The amount of viscosity exhibited by a fluid depends on a number of factors, including: • The chemical nature of the fluid. • Whether the fluid’s composition is homogeneous or heterogeneous. • All gases, and most simple liquids, obey Newton's law of viscosity and are accordingly called Newtonian fluids. • Water is a Newtonian fluid: • During non-turbulent conditions water’s viscosity does not change with changes in velocity. • Blood and pulmonary mucus are described as non-Newtonian fluids. 26 of 62 Viscosity Applied to Pathophysiology Although plasma is mostly water, it also contains other molecules such as electrolytes, proteins (especially albumin and fibrinogen), and other macromolecules. The various molecular interactions between these different plasma components gives plasma a viscosity higher than water. At 37°C plasma is about 1.8-times more o viscous than water at 37 C, so the relative viscosity of plasma is about 1.8. The presence of formed elements (red cells, white cells, and platelets) in the plasma further increases blood viscosity. Of these formed elements, red cells have the greatest effect on viscosity under normal conditions. • The viscosity of whole blood is determined in vitro using a device called a viscometer. • An increase in red cell hematocrit leads to an increase in relative viscosity. • Therefore, blood viscosity strongly depends on hematocrit. • At a normal hematocrit of 40-45%, the relative viscosity of blood is 4-5. o Note that the increase is non-linear, so that doubling hematocrit more than doubles the relative viscosity. • Polycythemia is a condition that produces an abnormal elevation in red cell hematocrit and an increase in blood viscosity. o This increases the resistance to blood flow and therefore increases the work of the heart and can impair organ perfusion. • Some patients with anemia have low hematocrits, and therefore reduced blood viscosities. • A second important factor that influences blood viscosity is temperature. • Just like molasses, when blood gets cold, it gets "thicker" and flows more slowly. o Therefore, there is an inverse relationship between temperature and viscosity. • Viscosity increases about 2% for each degree centigrade decrease in temperature. o Normally, blood temperature does not change much in the body. o However, if a person's hand is exposed to a cold environment and the fingers become cold, the blood temperature in the fingers will fall and the local blood viscosity will increase. o If this occurs together with sympathetic-mediated vasoconstriction blood flow will decrease in the colder region. 27 of 62 • • • • • • o Whole-body hypothermia is often induced (used) in critical care or surgical situations, blood viscos