Microscopy Techniques PDF
Document Details
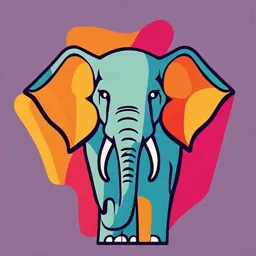
Uploaded by StableOcarina8423
Shashi SINGH
Tags
Summary
This document provides an overview of various microscopy techniques, including how they work, their applications, and the components required. It's a useful guide for understanding different types of microscopes.
Full Transcript
Microscopy Shashi SINGH To visualize a world beyond the visibility of human eye one needs to take help of magnifying systems and resolving systems. Resolution- ability to clearly see two close points Magnification- enlargement of samples A microscope comprises three subsystems: an illumination...
Microscopy Shashi SINGH To visualize a world beyond the visibility of human eye one needs to take help of magnifying systems and resolving systems. Resolution- ability to clearly see two close points Magnification- enlargement of samples A microscope comprises three subsystems: an illumination system to illuminate the sample an imaging system that produces a magnified image of the light that has interacted with the sample, A Viewing system by eye or using a camera system. A Simple schematic of a Microscope Visualization Magnifying or Analyser illumination Resolving power of a system is an important feature in the microscopy. The smallest feature size that can be observed with a standard light microscope is set by the optical wavelength (λ) used and the resolving power of the microscope objective, defined by its numerical aperture (NA), with the maximum value of NA = 1 for an objective in air. The resolution limit, which defines the smallest feature size (r) that can be distinguished is given by Rayleigh r = 0.61 × (λ/NA) Resolving power determines what is the minimal sample size one can observe clearly or resolve Magnification power of the microscope is dependent on the lenses available in the microscope; the total magnification of a compound microscope, which is the product of the objective magnification and the eyepiece magnification, typically lies in the range of 400-1000 x. Besides this two close points can only be resolved if there is contrast. So one can either impart contrst by stains or use optical methods to enhance contrast Phase contrast microscopy visualizes changes of optical phase caused by variations of the sample’s optical path length. optical phase shifts are not easily observable by eye, phase-contrast microscopy requires additional optical components that convert phase shifts caused by the sample into visible changes of brightness in the final image. By using apertures in incident light (with help of phase annulus) and filters in objective lens both the illumination system and the imaging system, one can enhance contrast. The phase plate shapes and selectively phase shift both the light from the sample, which carries the phase information of interest, and the illumination light such that they constructively interfere on the eye or detector to create a visible image. Similarly one can enhance contrast by DIC interference microscopy that uses polarizer or prism to rotate light. After traversing the sample, both beams are recombined such that they interfere with each other. This creates an image with contrast proportional to the difference in optical phase between the two polarized beams, hence the name “differential” interference microscopy. Both these microscopes are suitable for visualizing live unstained samples. And essential in a TC lab. Phase contrast often results in a halo around object at edges so focussing thicker objects is an issue. Dic DOES NOT CAUSE THE HALO EFFECT Dic phase Fluorescence microscopy Most cellular components are colorless and cannot be clearly distinguished under a microscope. To observe a cell organelle on needs to stain cells with chemicals that will react with organelles in a specific manner and make them visible. Most of these dyes work on fixed cells. In cell culture one needs to visualize and work with live cells many times. Certain dyes, also known as fluorophores or fluorochromes, are molecules that absorb excitation light at a given wavelength (generally UV), and after a short delay emit light at a longer wavelength. The delay between absorption and emission is negligible, generally on the order of nanoseconds. The emission light can then be filtered from the excitation light to reveal the location of the fluorophores. Often tagged to biomolecules for use in immunostaining, or tracking molecules in cells etc. Fluorescence microscopy uses a much higher intensity of a short wavelength light to illuminate the sample. This light excites fluorescence species in the sample, which then emits light of a longer wavelength. The image produced is based on the second light source or the emission wavelength of the fluorescent species rather than from the light originally used to illuminate, and excite, the sample. Most fluorescence microscopes in use are epifluorescence microscopes, where excitation of the fluorophore and detection of the fluorescence are done through the same light path through the objective The “fluorescence microscope” also known as an epifluorescence microscope, could have a more complicated design such as a confocal microscope, which uses optical sectioning to get better resolution of the fluorescent image. Typical components required in a fluorescence microscope are: A light source Four main types of light sources are used, including xenon arc lamps or mercury-vapor lamps with an excitation filter, lasers, and high- power LEDs. Lasers are mostly used for complex fluorescence microscopy techniques ike confocal, while xenon lamps, and mercury lamps, and LEDs with a dichroic excitation filter are commonly used for wide-field epifluorescence microscopes. The excitation filter The exciting filter is typically a bandpass filter that passes only the wavelengths absorbed by the fluorophore, thus minimizing the excitation of other sources of fluorescence and blocking excitation light in the fluorescence emission band. The dichroic mirror A dichroic filter or thin-film filter is a very accurate color filter used to selectively pass light of a small range of colors while reflecting other colors. The emission filter. The emitter is typically a bandpass filter that passes only the wavelengths emitted by the fluorophore and blocks all undesired light outside this band – especially the excitation light. By blocking unwanted excitation energy (including UV and IR) or sample and system autofluorescence, optical filters ensure the darkest background Fluorescent dyes (Fluorophore) A fluorophore is a fluorescent chemical compound that can re-emit light upon light excitation. Fluorophores typically contain several combined aromatic groups, or plane or cyclic molecules with several π bonds. Many fluorescent stains have been designed for a range of biological molecules. Some of these are small molecules that are intrinsically fluorescent and bind a biological molecule of interest. Major examples of these are nucleic acid stains like DAPI and Hoechst, phalloidin which is used to stain actin fibers in mammalian cells. Some metallic compounds also exhibit fluorescence especially quantum dots like CdS, BismuthSulfide etc Some fluorescent molecules are protein in nature obtained form marine animals like GFP The original green fluorescent protein (GFP) was discovered back in the early 1960s when researchers studying the bioluminescent properties of the Aequorea victoria jellyfish isolated a blue-light-emitting bioluminescent protein called aequorin together with another protein that was eventually named the green- fluorescent protein. Jellyfish-derived GFP has since been engineered to produce a vast number of useful blue, cyan and yellow mutants, and fluorescent proteins from a variety of other species have also been identified, resulting in further expansion of the available color palette into the orange, red and far-red spectral regions. FPs are beneficial for many applications, in particular for live-cell and whole-animal imaging. FPs are genetic labels and thus can be ‘built in’ using transgenic approaches. Antibody staining Immunostaining uses antibodies to detect an antigen in cells or tissue. The major benefit of immunostaining in immunohistochemistry (IHC) is the ability to see the desired target component/proteins /DNA anything in a tissue sample while cell while maintaining the spatial context and tissue architecture. Immunostaining can be performed either directly or indirectly. Conjugating our antibodies to a number of fluorophores enables direct labeling. Or one can use a indirect method against the first antibody Indirect method helps in specific staining as well moderate to high signal amplification depending on use of intermediate molecules like biotin etc. Confocal microscopy The confocal microscope uses fluorescence optics. But instead of illuminating the whole sample at once, laser light is focused onto a defined spot at a specific depth within the sample. This leads to the emission of fluorescent light at exactly this point. A pinhole inside the optical pathway cuts off signals that are out of focus, thus allowing only the fluorescence signals from the illuminated spot to enter the light detector. By scanning the specimen in a raster pattern, in one plane, one point at a time, images of one single optical plane are created. Thicker objects or 3D objects can be examined by scanning several optical planes and stacking them using a suitable microscopy software (z-stack). One can also analyze multicolor immunofluorescence staining using state-of-the-art confocal microscopes that include several lasers and emission/excitation filters. Lasers can be obtained with a specific emission wavelength or bandwidth. Confocal image The basic components of a modern confocal microscope are the pinholes, the objective lenses, and low-noise detectors in common with the original design but also typically include fast scanning mirrors, filters for wavelength selection, and laser illumination. The laser is directed onto a pair of scanning mirrors sweeping the beam in x and y directions of a single field of view and then moved incrementally across the entire sample to produce an image of the optical section, or slice. To collect a z-stack, the focal point is changed, and the scanning process repeated over the new slice. Upon collection of all optical sections from top to bottom, a 3-dimensional (3D) image can be reconstructed of the sample. The principal advantages of the LSCM are the optical sectioning capability, resolution, and versatility with 3D imaging. Most systems provide multi-color imaging, the ability to adjust the pinhole size to set the optical section thickness, and region-of-interest selection. Two Photon or Multi photon microscopy Imaging thicker samples is always an issue in any microscopy, Biological tissues strongly scatter light, making high-resolution deep imaging impossible for traditional—including confocal— fluorescence microscopy. So to examine thicker specimens or do deep imaging one can use fluorescence imaging using multiple low energy long wavelengths to excited a molecule. Since the energy of a photon is inversely proportional to its wavelength, the two absorbed photons must have a wavelength about twice that required for one-photon excitation. For example, a fluorophore that normally absorbs ultraviolet light (approximately 350 nanometers wavelength) can also be excited by two photons of near-infrared light (approximately 700 nanometers wavelength) if both reach the fluorophore at the same time. Advantage: because of longer wavelength Penetration is better and bleaching is Less. Total internal reflection is only possible in situations in which the propagating light encounters a boundary to a medium of lower refractive index. Specimen cells incorporating fluorescent molecules are supported on a glass microscope slide. The refractive indices of the glass slide (1.518) and the aqueous specimen medium (approximately 1.35) are appropriate to support total internal reflection within the glass slide. With adjustment of the laser excitation incidence angle to a value greater than the critical angle, the illuminating beam is entirely reflected back into the microscope slide upon encountering the interface, and an evanescent field is generated in the specimen medium immediately adjacent to the interface. The fluorophores nearest the glass surface are selectively excited by interaction with the evanescent field, and secondary fluorescence from these emitters can be collected by the microscope optics. This technique can be used to study phenomenon at cell surface or near it, changes in P membrane, adherence of cells, etc Wide field FRAP if a very intense light is used to excite the fluorophores, or they are exposed for too long, the fluorescent molecules lose their ability to emit light and are referred to as bleached or photobleached. Bleached fluorophores permanently lose their ability to fluoresce, the process is an irreversible photochemical alteration. Because of the dynamic nature of cells constituents; there are chances we can recoversome fluorescence in the bleache d pocket over a period of time. This phenomenon is called fluorescence recovery after photobleaching. Uses for FRAP have been identified such as for studying protein binding, including protein-protein interactions and protein trafficking, membrane. FLIP fluorescence loss in photobleaching (FLIP) reveals the connectivity between different compartments in the cell or the mobility of a molecule within the whole compartment, Fluorescence in the region of interest is monitored after repeated bleaching in qn adjacent area/compartment. FRET FRET is a process in which energy is transferred (non-radiating) from an excited donor fluorochrome to another molecule or acceptor. FRET happens on the close physical interaction of the two molecules (donor and acceptor) and can only occur if the distance between donor and acceptor is less than approximately 10 nm. FRET isused to determine molecular interaction/molecular proximity beyond the resolution limits of the classical light microscope Some FRET couples