Microbial Growth & Genetics PDF
Document Details
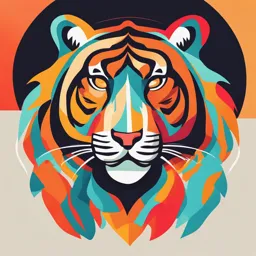
Uploaded by SensationalDifferential
UBC Okanagan
2024
Richard M Plunkett, PhD
Tags
Summary
These notes cover introductory microbiology, specifically focusing on microbial growth and genetics. The document includes diagrams and figures related to the foundational principles of genetics and microbes.
Full Transcript
BIOL 228 Introductory Microbiology 2024 Winter Term 1 Richard M Plunkett, PhD FIGURE 10.1 Siblings within a family share some genes with each other and with each parent. Identical twins, however, are genetically identical. Bacteria like Escherichia coli may acquire genes encoding virulen...
BIOL 228 Introductory Microbiology 2024 Winter Term 1 Richard M Plunkett, PhD FIGURE 10.1 Siblings within a family share some genes with each other and with each parent. Identical twins, however, are genetically identical. Bacteria like Escherichia coli may acquire genes encoding virulence factors, converting them into pathogenic strains, like this uropathogenic E. coli. (credit left: Senior Airman Lauren Douglas / U.S. Air Force; Public Domain.; credit right: modification of work by American Society for Microbiology) MICROBIAL GENETICS Eukaryotic Microbes Their molecular biology (i.e. genetics) is similar to ours Sexual reproduction Mitosis & meiosis Prokaryotic Microbes Do not carry out sexual reproduction Still can generate genetic diversity (they are not all identical, even within a strain) How do they do it? Reproduce Generate diversity FOUNDATIONS OF GENETICS Nucleic Acids (DNA & RNA) discovered in 19th c. (basis of molecular biology) Genetic Inheritance described in 19th c. (basis of classical genetics) Gregor Mendel & his pea plants FOUNDATIONS OF GENETICS Two ideas were not connected right away! Fruit fly Drosophila melanogaster served as model to link inheritance patterns to nucleic acids & chromosomes Corn plant Zea mays genetic studies added to knowledge of locations of genes on chromosomes Microbes & viruses have played important roles in genetics research In the words of French scientist Jacques Monod, “What is true for E. coli is also true for the elephant.” What does this mean? Two Examples: Acetabularia (single-celled algae) and bacterial transformation FIGURE 10.3 EXAMPLE: ACETABULARIA does (a) The cells of the single-celled alga to Acetabularia measure 2–6 cm and where have a cell morphology that can be observed with the naked eye. Each cell has a cap, a stalk, and a foot, 9 come which contains the nucleus. (b) Hämmerling found that if he removed the cap, a new cap would. 3 regenerate; but if he removed the foot, a new foot would not regenerate. He concluded that the genetic information needed for regeneration was found in the nucleus. (credit a: modification of work by James St. John) FIGURE 10.4 In a second set of experiments, Hämmerling used two morphologically different species and grafted stalks from each species to the feet of the other. He found that the properties of the regenerated caps were dictated by the species of the nucleus-containing foot. DNAwit is and structure no FIGURE 10.7 – BACTERIAL TRANSFORMATION dead dead cell DNA a in living transferring to live cells and creating Strain In his famous series of experiments, Griffith used two strains of S. pneumoniae. The S strain is pathogenic and causes death. Mice injected with the nonpathogenic R strain or the heat-killed S strain survive. However, a combination of the heat-killed S strain and the live R strain causes the mice to die. The S strain recovered from the dead mouse showed that something had passed from the heat-killed S strain to the R strain, transforming the R strain into an S strain in the process. STRUCTURE AND FUNCTION OF DNA & RNA REMEMBER The central dogma of modern genetics Flow of genetic information DNA RNA Protein (with a few exceptions…) Nucleic Acids (DNA & RNA) have similar structures (difference Linear macromolecules (polymers) made of nucleotides (monomers) Different nucleotides have different structures- how info stand Order of different nucleotides in strands of nucleic acids determine the sequence This is how information is stored! Let’s look at the structures FIGURE 10.11 (a) Each deoxyribonucleotide is made up of a sugar called deoxyribose, a phosphate group, and a nitrogenous base— in this case, adenine. (b) The five carbons within deoxyribose are designated as 1ʹ, 2ʹ, 3ʹ, 4ʹ, and 5ʹ. FIGURE 10.12 PAG - "Pure As Gold" base Nitrogenous bases within DNA are categorized into the two-ringed purines adenine and guanine and the single-ringed pyrimidines cytosine and thymine. Thymine is unique to DNA. FIGURE 10.13 Phosphodiester bonds form between the phosphate group attached to the 5ʹ carbon of one nucleotide and the hydroxyl group of the 3ʹ carbon in the next nucleotide, bringing about polymerization of nucleotides in to nucleic acid strands. Note the 5ʹ and 3ʹ ends of this nucleic acid strand. FIGURE 10.14 The X-ray diffraction pattern of DNA shows its helical nature. (credit: National Institutes of Health) FIGURE 10.15 In 1953, James Watson and Francis Crick built this model of the structure of DNA, shown here on display at the Science Museum in London. FIGURE 10.16 Watson and Crick proposed the double helix model for DNA. (a) The sugar-phosphate backbones are on the outside of the double helix and purines and pyrimidines form the “rungs” of the DNA helix ladder. (b) The two DNA strands are antiparallel to each other. (c) The direction of each strand is identified by numbering the carbons (1 through 5) in each sugar molecule. The 5ʹ end is the one where carbon #5 is not bound to another nucleotide; the 3ʹ end is the one where carbon #3 is not bound to another nucleotide. FIGURE 10.17 Hydrogen bonds form between complementary nitrogenous bases on the interior of DNA. if two FIGURE 10.18 can see single strands match from different organisms anywhere In the laboratory, the double helix can be denatured to single-stranded DNA through exposure to heat or chemicals, and then renatured through cooling or removal of chemical denaturants to allow the DNA strands to reanneal. (credit: modification of work by Hernández-Lemus E, Nicasio-Collazo LA, Castañeda-Priego R) FIGURE 10.20 (a) Ribonucleotides contain the pentose sugar ribose instead of the deoxyribose found in deoxyribonucleotides. (b) RNA contains the pyrimidine uracil in place of thymine found in DNA. FIGURE 10.21 an e Abreakage OH *double helix is Stable our to die that (compared groups the to RNA, muchless attack your Stable) back is DNA better for term (a) DNA is typically double stranded, whereas RNA is typically single stranded. Long storage - (b) Although it is single stranded, RNA can fold upon itself, with the folds stabilized by short areas of complementary base pairing within the molecule, forming a three-dimensional structure. FIGURE 10.22 A generalized illustration of how mRNA and tRNA are used in protein synthesis within a cell. FIGURE 10.23 Shape let comenecure Scan recoping A A.. A tRNA molecule is a single-stranded molecule that exhibits significant intramolecular base pairing, giving it its characteristic three-dimensional shape. FIGURE 10.24 GENOTYPE VS PHENOTYPE Both plates contain strains of Serratia marcescens that have the gene for red pigment. However, this gene is expressed at 28 °C (left) but not at 37 °C (right). (credit: modification of work by Ann Auman) FIGURE 10.25 DNA needs to be packaged + wounds Eukaryotic chromosomes typically have a significant amount of noncoding DNA, often found in intergenic regions. FIGURE 10.26 EUKARYOTIC GENOME IS IN NUCLEUS The genome of a eukaryotic cell consists of the chromosome housed in the nucleus, and extrachromosomal DNA found in the mitochondria (all cells) and chloroplasts (plants and algae). The cells shown in (b) represent cells obtained from a pap smear. The cells on the left are normal squamous cells whereas the cells on the right are infected with human papillomavirus and show enlarged nuclei with increased staining (hyperchromasia). FIGURE 10.27 GENOMIC DNA VS PLASMID DNA - not going to be in nucleus or wound up as much as eukaryotic (NUCLEOiD) Genome sequencing of Bacillus anthracis and its close relative B. cereus reveals that the pathogenicity of B. anthracis is due to the maintenance of two plasmids, pX01 and pX02, which encode virulence factors. FIGURE 10.28 GENOME SIZE & CRITTER COMPLEXITY There is great variability as well as overlap among the genome sizes of various groups of organisms and viruses. Log base 18 Scale FIGURE 11.1 Escherichia coli (left) may not appear to have much in common with an elephant (right), but the genetic blueprints for these vastly different organisms are both encoded in DNA. (credit left: modification of work by NIAID; credit right: modification of work by Tom Lubbock) FIGURE 11.2 The central dogma states that DNA encodes messenger RNA, which, in turn, encodes protein. FIGURE 11.3 Phenotype is determined by the specific genes within a genotype that are expressed under specific conditions. Although multiple cells may have the same genotype, they may exhibit a wide range of phenotypes resulting from differences in patterns of gene expression in response to different environmental conditions. FIGURE 11.6 GTP of cut esphots No qued G 1 leftoverfor backbone CMP 5 S 3 This structure shows the guanosine triphosphate (GTP) deoxyribonucleotide that is incorporated into a growing DNA strand by cleaving the two end phosphate groups from the molecule and transferring the energy to the sugar phosphate bond. The other three nucleotides form analogous structures. FIGURE 11.7 At the origin of replication, topoisomerase II relaxes the supercoiled chromosome. Two replication forks are formed by the op ening of the double-stranded DNA at the origin, and helicase separates the DNA strands, which are coated by single-stranded binding proteins to keep the strands separated. DNA replication occurs in both directions. An RNA primer complementary to the parental strand is synthesized by RNA primase and is elongated by DNA polymerase III through the addition of nucleotides to the 3ʹ-OH end. On the leading strand, DNA is synthesized continuously, whereas on the lagging strand, DNA is synthesized in short stretches called Okazaki fragments. RNA primers within the lagging strand are removed by the exonuclease activity of DNA polymerase I, and the Okazaki fragments are joined by DNA ligase. Strand binding - single proteins (protect Strand) DNA bumps Polymerase I off RNA S & replaces / DNA ⑭ gyrase ↓ ⑧ 31 sin ⑪ - 3 sliding clamp it Initiation W Elongation u Termination. Topoisemase breakress an 3 ~ " # RNA Primase creates RNA Primers grase coils Strands Dron & S - prevent re-coiling 3 origin of replication aa replace ↳ proteins bird & otic - toshort erkauf a telom Forensi * Single-stranded binding proteins DNA REPLICATION BUBBLE The Molecular Machinery Involved in Bacterial DNA Replication Enzyme or Factor Function DNA pol I Exonuclease activity removes RNA primer and replaces it with newly synthesized DNA DNA pol III Main enzyme that adds nucleotides in the 5’ to 3’ direction Helicase Opens the DNA helix by breaking hydrogen bonds between the nitrogenous bases Ligase Seals the gaps between the Okazaki fragments on the lagging strand to create one continuous DNA strand Primase Synthesizes RNA primers needed to start replication Single-stranded Bind to single-stranded DNA to prevent hydrogen bonding between DNA strands, reforming binding proteins double-stranded DNA Sliding clamp Helps hold DNA pol in place when nucleotides are being added Topoisomerase (DNA Relaxes supercoiled chromosome to make DNA more accessible for the initiation of gyrase) replication; helps relieve the stress on DNA when unwinding, by causing breaks and then resealing the DNA FIGURE 11.8 Eukaryotic chromosomes are typically linear, and each contains multiple origins of replication. FIGURE 11.9 In eukaryotes, the ends of the linear chromosomes are maintained by the action of the telomerase enzyme. FIGURE 11.10 Casternate on , The process of rolling circle replication results in the synthesis of a single new copy of the circular DNA molecule. TRANSCRIPTION – MAKING AN RNA COPY OF DNA TRANSCRIPTION Information from one or more genes in DNA is copied as an RNA transcript Requires DNA; RNA polymerase; RNA nucleotides Only one strand of DNA is copied (coding strand) by using the other strand (noncoding strand) as the template for RNA THREE STAGES OF TRANSCRIPTION in BACTERIA Initiation RNA polymerase binds to DNA Elongation Nucleotides added by RNA polymerase to growing RNA strand using DNA template Termination Two ways to end process—both depend on DNA sequence FIGURE 11.11 During elongation, the bacterial RNA polymerase tracks along the DNA template, synthesizes mRNA in the 5ʹ to 3ʹ direction, and unwinds and rewinds the DNA as it is read. TRANSLATION – MAKING A PROTEIN FROM GENETIC INFORMATION TRANSLATION Information from one or more genes in RNA is translated to protein Requires mRNA; ribosomes; tRNAs (with amino acids attached) Information from a strand of mRNA is used to build a strand of amino acids (polypeptide) that is the precursor to a protein THREE STAGES OF TRANSLATION in BACTERIA Initiation Ribosome binds to mRNA Elongation Amino acids (atch. to tRNAs) added by ribosome to growing polypeptide using codons in mRNA sequence Termination Depends on mRNA sequence (stop codons) and release factors—no tRNAs involved FIGURE 11.12 This figure shows the genetic code for translating each nucleotide triplet (codon) in mRNA into an amino acid or a termination signal in a nascent protein. The first letter of a codon is shown vertically on the left, the second letter of a codon is shown horizontally across the top, and the third letter of a codon is shown vertically on the right. (credit: modification of work by National Institutes of Health) FIGURE 11.13 In prokaryotes, multiple RNA polymerases can transcribe a single bacterial gene while numerous ribosomes concurrently translate the mRNA transcripts into polypeptides. In this way, a specific protein can rapidly reach a high concentration in the bacterial cell. FIGURE 11.14 (a) After folding caused by intramolecular base pairing, a tRNA molecule has one end that contains the anticodon, which interacts with the mRNA codon, and the CCA amino acid binding end. (b) A space-filling model is helpful for visualizing the three-dimensional shape of tRNA. (c) Simplified models are useful when drawing complex processes such as protein synthesis. FIGURE 11.15 Translation in bacteria begins with the formation of the initiation complex, which includes the small ribosomal subunit, the mRNA, the initiator tRNA carrying N-formyl-methionine, and initiation factors. Then the 50S subunit binds, forming an intact ribosome. FIGURE 11.16 (a) In prokaryotes, the processes of transcription and translation occur simultaneously in the cytoplasm, allowing for a rapid cellular response to an environmental cue. (b) In eukaryotes, transcription is localized to the nucleus and translation is localized to the cytoplasm, separating these processes and necessitating RNA processing for stability. coding protein dsDNA arno Sequence Translata, · Shire-Dalg e /(stop b ribosom mRNA created RNAPOT site 31 > - ave / "35'ver Trailer ( S'UTR same as (Pribrone , template strand translation L coding 513) promoter terminata Leader Start poly protect gene Istate RNA exits Strand in blon so Site Start it gets translated > - transcription direction Copposite ab oftempla I GENETIC MUTATIONS – CHANGES TO DNA SEQUENCES MUTATIONS Heritable Changes to DNA Sequence DNA in genome gets changed (several mechanisms exist) DNA replication produces copies carrying the newly-changed sequence Changes in genes affect mRNA protein TYPES OF CHANGES TO DNA (Mutations) Point mutation Substitution of one nucleotide for another (changes one letter in sequence) Insertion Extra nucleotide(s) added to sequence (makes sequence longer) Deletion Removal of nucleotide(s) from sequence (makes sequence shorter) GENETIC MUTATIONS – CHANGES TO DNA SEQUENCES CHANGES TO PROTEIN Point mutation Changes to codon can affect translation Silent mutation: change has no effect on protein sequence Missense mutation: change substitutes one amino acid for another Nonsense mutation: change adds stop codon where none previously existed Addition or Deletion mutation Causes frameshift mutation Changes sequence of every codon (thus amino acids in protein) downstream from mutation FIGURE 11.18 FIGURE 11.18 GENETIC MUTATIONS – CHANGES TO DNA SEQUENCES WHAT CAUSES MUTATIONS? Spontaneous Errors in DNA Replication DNA Polymerase is not perfect! DNA replication process can produce errors If not repaired by cellular mechanisms, errors become mutations Induced Mutations Caused by external agents: Mutagens Chemical Mutagens Radiation FIGURE 11.20 – CHEMICAL MUTAGEN EXAPMLES (a) 2-aminopurine nucleoside (2AP) structurally is a nucleoside analog to adenine nucleoside, whereas 5- bromouracil (5BU) is a nucleoside analog to thymine nucleoside. 2AP base pairs with C, converting an AT base pair to a GC base pair after several rounds of replication. 5BU pairs with G, converting an AT base pair to a GC base pair after several rounds of replication. (b) Nitrous acid is a different type of chemical mutagen that modifies already existing nucleoside bases like C to produce U, which base pairs with A. This chemical modification, as shown here, results in converting a CG base pair to a TA base pair. FIGURE 11.21 – INTERCALATING MUTAGEN Intercalating agents, such as acridine, introduce atypical spacing between base pairs, resulting in DNA polymerase introducing either a deletion or an insertion, leading to a potential frameshift mutation. FIGURE 11.22 (a) Ionizing radiation may lead to the formation of single-stranded and double-stranded breaks in the sugar-phosphate backbone of DNA, as well as to the modification of bases (not shown). (b) Nonionizing radiation like ultraviolet light can lead to the formation of thymine dimers, which can stall replication and transcription and introduce frameshift or point mutations. GENETIC MUTATIONS – CHANGES TO DNA SEQUENCES IDENTIFYING MUTANTS Evaluating Possible Mutagens Chemicals that cause mutations can be identified Test Organism is Salmonella typhimurium Normal cells can synthesize histidine—an essential amino acid Expose cells to possible mutagen Grow cells on universal growth medium (complete medium) Colonies will grow on petri dish Try to replicate growth on plates containing defined medium that contains no histidine What does it mean if some cells cannot grow on defined medium? FIGURE 11.24 Identification of auxotrophic mutants, like histidine auxotrophs, is done using replica plating. After mutagenesis, colonies that grow on nutritionally complete medium but not on medium lacking histidine are identified as histidine auxotrophs. GENETIC MUTATIONS – CHANGES TO DNA SEQUENCES THE AMES TEST Evaluates Possible Carcinogenic Mutagens Mutations can cause cancer Test Organism is Salmonella typhimurium strain See the previous experiment Auxotrophic for histidine (it cannot make its own histidine—an amino acid) Expose cells to possible mutagen + rat liver extract Some chemicals become mutagenic after processing by the liver Grow cells on defined growth medium (no histidine) Colonies will grow on petri dish What does it mean if lots of colonies grow, compared to a control? FIGURE 11.25 The Ames test is used to identify mutagenic, potentially carcinogenic chemicals. A Salmonella histidine auxotroph is used as the test strain, exposed to a potential mutagen/carcinogen. The number of reversion mutants capable of growing in the absence of supplied histidine is counted and compared with the number of natural reversion mutants that arise in the absence of the potential mutagen. GENERATING GENETIC DIVERSITY WITHOUT SEX PROKARYOTES REPRODUCE BY BINARY FISSION Genes are inherited by vertical transmission Cells are exact copies of parent cell BUT: genes can also be passed along by horizontal transmission Genes passed from one cell to another MECHANISMS OF HORIZONTAL TRANSMISSION Transformation: naked DNA taken up from environment Transduction: genes transferred between cells by a virus Conjugation: genes passed from one cell to another through a tube “JUMPING GENES” Transposition: genes moved from one location to another within a chromosome FIGURE 11.26 There are three prokaryote-specific mechanisms leading to horizontal gene transfer in prokaryotes. (a) In transformation, the cell takes up DNA directly from the environment. The DNA may remain separate as a plasmid or be incorporated into the host genome. (b) In transduction, a bacteriophage injects DNA that is a hybrid of viral DNA and DNA from a previously infected bacterial cell. (c) In conjugation, DNA is transferred between cells through a cytoplasmic bridge after a conjugation pilus draws the two cells close enough to form the bridge. FIGURE 11.28 Typical conjugation of the F plasmid from an F + cell to an F − cell is brought about by the conjugation pilus bringing the two cells into contact. A single strand of the F plasmid is transferred to the F − cell, which is then made double stranded. FIGURE 11.31 – “JUMPING GENES” Transposons are segments of DNA that have the ability to move from one location to another because they code for the enzyme transposase. In this example, a nonreplicative transposon has disrupted gene B. The consequence of that the transcription of gene B may now have been interrupted. FIGURE 11.32 – REGULATION OF GENE EXPRESSION Polycistronic mRNA contains more than one gene Transcribe together Translated into multiple proteins In prokaryotes, structural genes of related function are often organized together on the genome and transcribed together under the control of a single promoter. The operon’s regulatory region includes both the promoter and the operator. If a repressor binds to the operator, then the structural genes will not be transcribed. Alternatively, activators may bind to the regulatory region, enhancing transcription. 61 REGULATION OF TRANSCRIPTION INITIATION Different kinds of genes, based on transcriptional control Constitutive genes are housekeeping genes that are expressed continuously by the cell Inducible genes are genes that code for inducible enzymes needed only in certain conditions Repressible genes are genes that are expressed most of the time, but can be repressed in certain conditions 62 CONSTITUTIVE GENES Housekeeping genes that are expressed continuously by the cell Think of your utilities (water, electricity, internet): you want it “on” all the time 63 REPRESSIBLE GENES Enzymes that function in biosynthetic pathways are products of repressible genes Generally these enzymes are always present unless the end product in the biosynthetic pathway is available Think of your mail delivery: you want it “on” all the time, but can turn it off when you are away on holiday (put it on hold) In cells: don’t make more of what you already have 64 INDUCIBLE GENES Are genes that code for inducible enzymes needed only in certain conditions Inducible enzymes are present only when their substrate (inducer - effector molecule) is available Think of your plumber, or the fire department: you only want them “on” when needed—you do not need the fire department at your house every day! 65 CONTROL OF TRANSCRIPTION INITIATION BY REGULATORY PROTEINS Induction and repression occur because of the activity of regulatory elements like proteins and DNA binding domains These proteins either inhibit transcription (negative control) or promote transcription (positive control) Regulated genes are under positive or negative control This refers to how the process of transcription is controlled (not whether a gene is inducible or repressible) 66 NEGATIVE TRANSCRIPTIONAL CONTROL Binding of regulatory protein at DNA regulatory site inhibits transcription initiation mRNA expression is reduced Repressor proteins (negative control) exist in active and inactive forms inactive protein is activated by inhibitor OR active protein is inactivated by inducer 67 POSITIVE TRANSCRIPTIONAL CONTROL Binding of a regulatory protein at a regulatory region on DNA promotes transcription initiation mRNA synthesis is increased Activator proteins (positive control) exist in active and inactive forms inactive protein is activated by inducer active protein is inactivated by inhibitor FIGURE 11.33 – TRP OPERON EXAMPLE The five structural genes needed to synthesize tryptophan in E. coli are located next to each other in the trp operon. When tryptophan is absent, the repressor protein does not bind to the operator, and the genes are transcribed. When tryptophan is plentiful, tryptophan binds the repressor protein at the operator sequence. This physically blocks the RNA polymerase from transcribing the tryptophan biosynthesis genes. FIGURE 11.34 – LAC OPERON INDUCTION EXAMPLE The three structural genes that are needed to degrade lactose in E. coli are located next to each other in the lac operon. When lactose is absent, the repressor protein binds to the operator, physically blocking the RNA polymerase from transcribing the lac structural genes. When lactose is available, a lactose molecule binds the repressor protein, preventing the repressor from binding to the operator sequence, and the genes are transcribed. FIGURE 11.35 – WHAT IF GLUCOSE AND LACTOSE ARE BOTH PRESENT? When grown in the presence of two substrates, E. coli uses the preferred substrate first (in this case glucose) until it is depleted. Then, enzymes needed for the metabolism of the second substrate are expressed and growth resumes, although at a slower rate. FIGURE 11.36 – CAMP IS “LOW GLUCOSE” SIGNAL When ATP levels decrease due to depletion of glucose, some remaining ATP is converted to cAMP by adenylyl cyclase. Thus, increased cAMP levels signal glucose depletion. FIGURE 11.37 – LAC OPERON REPRESSION EXAMPLE (a) In the presence of cAMP, CAP binds to the promoters of operons, like the lac operon, that encode genes for enzymes for the use of alternate substrates. (b) For the lac operon to be expressed, there must be activation by cAMP-CAP as well as removal of the lac repressor from the operator.