Vision I - Cornea -> LGN Lecture Notes PDF
Document Details
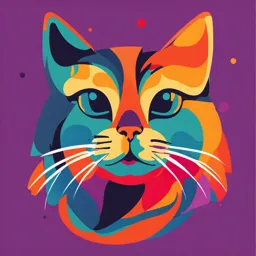
Uploaded by PermissibleBigfoot
Lincoln Memorial University-DeBusk College of Osteopathic Medicine
Tony Harper
Tags
Summary
This document presents a lecture on vision, specifically focusing on the pathway from the cornea to the lateral geniculate nucleus (LGN). The objectives and content cover the visual neural pathway, the relationship between visual fields and retinal fields, the role of the occipital lobe in visual processing, and various visual field deficits. The document also delves into the internal anatomy of the eye, including segment structures, and visual pathways.
Full Transcript
Vision I Cornea - > LGN Tony Harper DOSYS-715 MedNeuro Lecture 12 Tues April 23 @1pm Objectives After viewing this lecture and upon learning the subject matter in this lecture, the student will be able to: 1. Describe the visual neural pathway from the retina to the visual cerebral cortex. 2. Descri...
Vision I Cornea - > LGN Tony Harper DOSYS-715 MedNeuro Lecture 12 Tues April 23 @1pm Objectives After viewing this lecture and upon learning the subject matter in this lecture, the student will be able to: 1. Describe the visual neural pathway from the retina to the visual cerebral cortex. 2. Describe the relationship of visual fields to retinal fields to representation in the visual cortex in the occipital lobe. 3. Relate the important areas in the cerebral cortex to conscious processing of visual information. 4. Relate various lesions of the visual pathway to visual field deficits. Anterior Segment (Cavity) Eye – Internal Anatomy Cornea Cornea -> Lens Filled with watery aqueous humour Split into anterior and posterior “chambers” by iris Iris Scleral Venous Sinus Lens Suspensory Ligament of the Lens Ciliary Muscle Sclera Posterior Segment (Cavity) Lens -> Retina Vitreous Chamber Choroid/Uveal Layer Retina Optic Disc Filled with goopy vitreous humour/body Central Retinal Artery Optic Nerve Macula Lutea & Fovea Centralis Pigment Layer of Retina Canal of Schlemm (Sinus venosus sclerae) Sphincter Pupillae Anterior Lens Epithelium Ciliary Muscles Neural Layer of Retina Dilator Pupillae Sphincter Pupillae Aqueous humour supports metabolism of internal eye structure (especially in anterior segment). It is recycled every ~90mins into venous circulation through the “canal of Schlemm” Pigmented Layer of the Iris Ciliary Process Unpigmented Layer of the Iris Structures visible in anterior segment Sclera (covered with bulbar conjunctiva) – continuous with CNS dura mater Limbus - 1-2mm transition zone between opaque sclera and clear cornea Cornea – avascular (but has nerve endings) clear cover of eye Iris – Pigmented smooth muscle ring. Has dilator and constrictor muscles Pupil – Aperture for light through iris Lens – Clear light focusing structure deep to pupil and iris Fundoscopic Exam Central retinal a. Fovea centralis Optic disc (and cup) Macula lutea Central retinal v. 5 Physiologic Blind Spot and Optic Disc Location is in temporal visual field (3mm to nasal side of posterior pole of eye) Has small central pit – “optic cup” Due to projection of visual stimuli onto the optic disc Optic disc is where all ganglion cell neurons converge and become myelinated, to leave eyeball( at lamina cribrosa) and form optic nerve Papilledema Eyes and optic nerve are projections from CNS (diencephalon) Optic nerve contains all three layers of meninges Optic disc swelling from increased intracranial CSF pressure (e.g. hydrocephalus) Refraction When a wave moves at an angle into a denser medium the part of the wave entering the new medium gets “delayed” For the wavefronts to stay connected at the boundary the entire wave must change direction Since the entire wave does not slow down at once, waves “refract” at interface of two materials Amount of refraction is wavelength dependent (red and green wavelengths require slightly more accommodation than blue wavelengths) – but this is usually not noticeable n = refractive index of a material Snell’s Law: n1·sinθ1 = n2·sinθ2 Moving into denser medium (higher n) bends light towards normal Moving into less dense medium (lower n) bends away from normal Going through a translucent object (lens, prism, etc.) light rays coming from the air bend toward normal at “anterior” surface, and away from normal at “posterior” surface Each eye has many curved refractive interfaces, with precisely controlled refractive indices (n) and distances between structures Curved refractive surfaces cause the interface normal to project away from center of curvature radially ~80% of total refraction in eye happens at aircornea interface Only ~20% of refraction occurs at lens, however since adult eye can’t change shape, the internal elasticity of lens is used to change focal depth of vision Usually, the segment of light within lens is negligible (the “Thin Lens approximation”) Light refracts towards normal-line at anterior margin of lens, away from normal-line at posterior margin Rays parallel to the optical axis entering the convex lens will converge at focal point behind lens Rays passing through anterior focal point of convex lens will be “collimated” (made parallel) inside eye Rays with intermediate orientations will be refracted to intermediate degrees (e.g. rays passing through exact center of the lens won’t be net-refracted). Ultimately causing all light originating from a single point of an external object to be “bundled together” at one point on the retina The more curved the convex lens, the closer the focal points move toward the center of the lens Aphakia (Lack of Lens) Lens also filters out ultraviolet light Individuals lacking a lens (aphakia) have cyanopsia (vision with permanent bluish tint), and can actually see UV light! Lens also helps hold iris in place, so individuals with aphakia also exhibit “wobbly iris” (iridodonesis) Monet, Young girls in a rowboat 1887 Cataract (Opaque Lens) Lens tends to stiffen and become “slightly yellowish” with age (Xanthopsia) Pathological opacity of lens is called a cataract “Celestina” Van Gogh self 1904, portrait from Pablo Picasso’s blue phase Ideally, we want to create accurate representation of visual field on a 2D sensory sheet However, light travels out from sources in many directions. This would produce a spread out and blended representation at the receptor Nautilus is an invertebrate with an open pinhole eye. Seawater freely flows through pupil One way to limit the directions that light can reach the sensory sheet from is to place the sensory sheet in a “dark box”, with only a pinhole opening for light to enter This kind of “Pinhole Camera” creates an upside-down and left-right flipped representation of the visual field on the back of the “box” To make the image sharper the size of the pinhole must be reduced, which limits the amount of light that can reach the detector To increase the amount of light that can be used to form images (number of photons) a convex lens can be placed behind opening to “focus” light onto sensory sheet However, the curvature of the lens needs to be precisely matched to its distance from the light source and its distance to the sensory sheet The Shape of Eyeball is Fixed, So The Lens is Swishy To focus on close-up objects, the ciliary muscles contract causing the suspensory ligaments to relax resulting in a more spherically shaped lens. The ciliary muscles receive parasympathetic innervation from the oculomotor nerve. To focus on far-off objects, the ciliary muscles relax causing the ligaments to pull the lens into a flattened shape. With age, the lens loses it’s elasticity and can not change shape – a condition called Presbyopia. Ciliary Muscle “Zoomed Out” To focus on near objects Lens “Zoomed In” To focus on far objects Suspensory Ligament of the Lens Ciliary Body Function Ciliary Muscles: Circumferential Müller’s Muscle Radial Brüke’s Muscle Ciliary m. relaxed – zonular fibers tensed – front side of lens is flatter – good for far vision Ciliary m. tensed - ↓ pull on zonular fibers – lens rounds up for near vision This process is called accommodation Requires parasympathetic innervation to ciliary m. CN III – oculomotor n. Presbyopia – normal loss of accommodation to near vision Due to stiffening of lens Normal process of aging – 40-45 y.o. Emmetropia Ametropia Staphyloma: irregular bulges (ectasia) of eye can cause myopia Unfocused vision due to refractive error Geometry of eye correct. Light passing through lens focuses correctly on back of eye (retina) “Nearsightedness” Eyeball too long (very common) “Farsightedness” Eyeball too short Image focuses inside posterior cavity, not on retina Image focuses posterior to retina Fixed with “negative” (concave) lens Fixed with “positive” (convex) lens Blurry vision caused by irregular geometry in cornea or lens Fixed by custom made corrective lenses Retina Outer – pigmented Inner - neural Axons of ganglion cells travel across retina to form optic nerve at the optic disc Pigment Layer of Retina Neural Layer of Retina Lens Fibers Hyaloid Artery (remnant becomes central retinal artery in adult) Optic Nerve Ectoderm Pigment layer of retina just 1 cell thick lining of choroid layer of eyeball. Surface of detachment in “detached retina” is between neural and pigmented layers of retina Mesenchyme Eyelid Forebrain Retina and Optic Nerve are outgrowths of diencephalon, not technically part of PNS Optic vesicle and lens placode interact during early development to form adult retina and lens Lens Placode Invaginating Lens Placode Invaginating Optic Vesicle Optic Vesicle Wall of Forebrain Optic Grooves Ectoderm Outer Retina Pigment Layer of Retina Organization of the Retina Retina has 10 histologic layers (don’t need to memorize) Photoreceptors ~120 million rods ~7million cones some books call photoreceptors 1° Sensory Neurons Neural Layer of Retina Bipolar Cells 1° Sensory Neurons (in our book) In vertebrate retina, light and visual information (action potentials) travel in opposite directions Retinal Interneurons and Glia Organization of the Retina Interneurons Horizontal Cells Interconnect photoreceptors and bipolar cells in outer plexiform layer Graded potentials and hyperpolarize in light Amacrine (“not long process”) Cells No axons, few dendrites Connect bipolar cells to ganglion cells Some have action potentials Glia Müller Cells (not shown) Similar to astrocytes Run between (and create) inner and outer limiting membranes Light is scattered as it passes through cells to reach the photoreceptors. Layers are thinnest in the fovea and macula. Anatomy of Rods and Cones Rods (scotopic vision) Pigment Layer of Retina ~1000 x more sensitive than cones (possible to stimulate from single photon) ~100 discs per rod, 10,000 rhodopsins per disc But longer adaptation time than cones ~ 15-30 converge onto 1 bipolar cell Contain rhodopsin (visual purple) in outer segment “discs” Cones (photopic vision) Contain iodopsin in outer segment “folds” 1:1 convergence with bipolar cells 64% “Long Wavelength”(red/erythrolabe) 32% “Medium” (green/chlorolabe) 2-4% “Short” (blue/cyanolabe, more sensitive to light than other cones) Because s-cones are much rarer, need to develop “blue amplifier” in brain to reliably detect bluish light Protanopia/anomaly Peak region for pigment melanopsin (12.5%/12.5%): no/few red cones. Scotopic Vision Photopic Deuteranopia/anomaly Precise color vision (only Low-light (mostly rods)cones) vision (12.5%/62.5%): Perceived “Monochromatic” colorsrods correspond to different firing In absence of light stimulation have spontaneous rate (0.01Hz) Individuals have no/few frequencies(wavelengths), withblack different cones having producing “Eigengrau” not green cones. peakofsensitivities different Perception blackness requires active inhibition of photoreceptors Tritanopia/anomaly(very However, color is not a linear or 1-dimensional perception rare): Individuals have Many colors can only be formed by a mixture of different wavelengths Uniform activation of all photoreceptors are used to detectno/few “luminance” blue cones. (e.g. white,pink, purple) (lightness/darkness) of visualofscene (notcolor just in low light) Some women are Luminance is also a component perceived (e.g. brown) In digital images luminance is usually just set to the “green” channel “tetrachromats” Percieved Color vs. Component Wavelength Perceived color of an object depends not only on the wavelength reflected by the object, but also on wavelengths reflected by the surroundings (color constancy; e.g., perceived colour of object does not change when viewed during sunset). http://www.thenakedscientists.com/HTML/articles/article/martinwestwellcolumn9.htm/ Some neurons in V4 are ‘color’-sensitive and respond to wavelengths in the centre of their receptive field, depending on the wavelengths reflected from the background. Neurons in the primary visual pathway and V2 are only ‘wavelength’sensitive. Inner Layers of Retina Retinal Ganglion Cells RGCs ~1.5 million ganglion cells per eye (40% of all cranial nerve fibers) Rate of visual information leaving each eye ~ 10Mb/sec Cell bodies present in “ganglion cell layer”, axons make up “optic nerve layer” Some RGCs are intrinsically photosensitive, using pigment melanopsin Two Categories of RGCs: Parasol/M(Magnocellular)-RGCs Larger diameter axons (faster), and more transient action potential responses Bigger receptive fields Motion sensitive (can’t detect color) Project to layers 1 & 2 of LGN Midget/P(Parvocellular)-RGCs Thinner diameter axons, and more sustained action potential responses Smaller receptive fields More detail sensitive (can detect gradients of color) Project to layers 3-6 of LGN This is a separate classification from “On/Off center Dark/Light” cells other lectures Organization of the Retina This retina diagram has more detail than what is required for exam S = Sclera C = Choroid coat PE = Pigmented epithelium P = Outer segments of rods and cones O = Nuclei of rods and cones OPL = Outer synaptic layer I = Nuclei of bipolar neurons PL = Inner synaptic layer G = Ganglion cell layer fovea Macula lutea (yellow spot)is area in fundus of eye where UV absorbing Xanthophyll molecules are enriched Fovea centralis (fovea) is ~ 1mm diameter pit where External plexiform, internal nuclear, internal plexiform, ganglion cell, and optic nerve fiber layers are pushed away (keeps internal limiting membrane) Foveola is 0.3mm area in center of fovea which lacks blood vessels and where only densely packed cones (mostly M and L) are present (200,00/mm2) Fundoscopic view of Right Eye: Optic Disc Superior Temporal Retina Fovea Nasal Retina (Papillomacular Bundle) Nasal Retina Inferior Temporal Retina Fundoscopic view of Optic Disk Temporal Retina Nasal Retina Axons from ganglion cells from the temporal hemiretina enter optic disc along its superior and inferior borders Axons from the nasal retina enter into horizontal band along optic disc (papillomacular bundle enters center of optic nerve) Lesions to the 2° neuron (RGC) axons within the brain can cause retrograde “Wallerian” atrophy of RGCs in optic nerve. Degeneration of axons leave behind myelin sheaths, causing atrophied regions of optic disc to become whiter (“pallor”) So for example, lesions of the axons of the nasal hemiretina can produce horizontal “band atrophy” or “bow-tie atrophy” leaving behind horizontal band of atrophy in optic dis. Atrophy of temporal RGCs leave behind more diffuse vertical band. Visual Fields / Retinal Fields Temporal visual field is larger than the nasal visual field. More ganglion cells in nasal retina Binocular visual field is wider superiorly than inferiorly Temporal hemiretinas: 40% of ganglion cell fibers. Receive images from medial visual field. Ganglion cells project to ipsilateral LGN (layers 2,3,5 prime numbers) Nasal hemiretinas: 60% of ganglion cell fibers. Receive images from lateral visual field. Ganglion cells project to contralateral LGN (layers 1,4,6) Left visual field Temporal retina Nasal retina Right visual field Nasal retina Temporal retina Goldmann Visual Field Right Left Chart is viewed from perspective of patient looking into the bowl. Note the blind spot in the temporal field. Visual field - #2 – purple line Lines #3 and #4 – smaller isopters with dimmer light Main Visual Pathway (Retino-Geniculo-Calcarine) Retina glutamate Photoreceptors Bipolar cells 1° Ganglion cells 2° Optic Nerve 2° Optic chiasm 2° Optic tract 2° Lateral geniculate nucleus 3° Optic radiation 3° Visual Cortex – occipital lobe Left visual field Right visual field Retinal fields Visual Pathway Lesions Scotoma Local lesion in retina Blind spot Central scotomas and claudication(worn out from use) often symptom of optic nerve ischemia Cecocentral scotomas are lesions of retina that fuse with blind spot Visual Pathway Lesions Monocular Visual Loss Damage to retina or optic nerve looses visual information without ability to compensate from contralateral side. Lesions before optic chiasm can cause blurred vision and loss of color vision (achromotopsia, red usually lost first) while lesions after chiasm will not X Marks the Spot “X” Latin Decem e.g. Decussation “χ” Greek Chi e.g. Chiasm Inputs: Optic Nerves Outputs: Optic Tracts and Hypothalamus (suprachiasmatic nucleus) Ganglion cell axons from each nasal hemiretina cross at chiasm Suprachiasmatic nucleus of hypothalamus receives direct ganglion cell fibers (e.g. those expressing melanopsin) – controls circadian rhythms Degree of axon divergence at the optic chiasm in different vertebrate species Criss-crossing of ganglion cell fibers is actually ancient feature Humans and other primates are “advanced” because they have a large number of ganglion cell fibers that do not cross These uncrossed fibers are from the temporal hemiretina (medial visual field) and still only represent 40% of total ganglion cells Bitemporal Hemianopsia Midsagittal lesion of optic chiasm Visual Pathway Lesions Bitemporal Hemianopsia Bilateral “bowtie” atrophy of optic disk Post-fixational blindness Very rarely: See-saw nystagmus Inferior chiasm has inferior retinal fibers (from superior visual field) Superior chiasm has superior retinal fibers (from inferior visual field) Bitemporal Hemianopia “curtain” lowers from superior visual field Pituitary Adenoma Compresses inferior-nasal fibers Bitemporal Hemianopia “curtain” raises from superior inferior field Different sides of optic chiasm have fibers from differing parts of retina Progression of vision loss can therefore be clue to location of lesion Not able to count fingers – bad vision Not able to detect hand motion – very bad vision Craniopharyngioma Compresses superior-nasal fibers Optic Nerve and Chiasm Lateral compression of optic chiasm can produce an opposite kind of visual field defect – Binasal Hemianopia Again, progression can be used to locate lesion, since vision will degrade earlier on side with aneurism/tumor Lesions to the proximal 4mm of the optic nerve can damage contralateral inferonasal retinal fibers too The reason for this was hypothesized to be caused by “Wilbrand’s Knee” – a loop of contralateral fibers in optic nerve. Now though to be just close proximity of contralateral fibers to central fibers of retina Ischemic lesion of proximal optic nerve can therefore cause Junctional Scotoma – central scotoma of ipsilateral eye, and superior temporal crescent loss in contralateral eye Most common cause is meningiomas Optic Tracts Stimuli from the nasal retinas cross in the optic chiasm to opposite optic tract Stimuli from the temporal retinas do not cross in optic chiasm and continue in the optic tract on the same side Each optic tract contains uncrossed temporal retinal axons and crossed nasal retinal axons End result – each optic tract contains all visual stimuli from the opposite visual field Left retinal field Right retinal field Subcortical Visual Structures Targets of optic tracts Controls pupillary light reflex, communicates with contralateral pretectum across posterior commissure Receives fibers from rod-rich peripheral visual areas. Sends fibers to Pulvinar and then extrastriate visual cortex – tectopulvinaar path. Tectospinal tract – reorient to visual and auditory stimuli