Skeletal Muscle Physiology Mechanisms PDF
Document Details
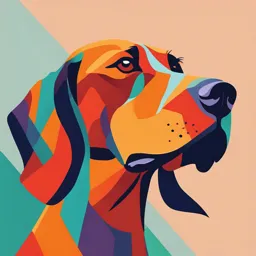
Uploaded by EffectiveMesa
University of Iowa
Vitor A. Lira
Tags
Summary
Skeletal muscle physiology lecture notes cover the mechanisms of muscle atrophy and recovery. This document details the major pathways involved in muscle atrophy, including protein degradation via ubiquitin-proteasome and autophagy-lysosomal systems. This document also details acute muscle atrophy due to decreased use, and signaling mechanisms, including fiber type shift, and fasting/starvation.
Full Transcript
HHP 4130/6130 Skeletal Muscle Physiology Mechanisms involved in muscle atrophy and recovery from atrophy Office Hours: Wednesdays 3:00-5:00pm, via Zoom meeting (see ICON). Vitor A. Lira Associate Professor Dept. Health & Human Physiology F.O.E. Diabetes Research Center Pappajohn Biomedical Institut...
HHP 4130/6130 Skeletal Muscle Physiology Mechanisms involved in muscle atrophy and recovery from atrophy Office Hours: Wednesdays 3:00-5:00pm, via Zoom meeting (see ICON). Vitor A. Lira Associate Professor Dept. Health & Human Physiology F.O.E. Diabetes Research Center Pappajohn Biomedical Institute Email: [email protected] I. Major pathways involved in atrophy and recovery from atrophy Muscle protein degradation Major proteolytic systems Ubiquitin-proteasome system Autophagy-lysosomal system Basal protein degradation across fiber types Acute muscle atrophy & signaling mechanisms Atrophy from decreased use models Fiber type shift Fasting/starvation Major signals that regulate muscle atrophy Mechanisms of recovery from atrophy Satellite cells…required or not? I. Major pathways involved in atrophy and recovery from atrophy Muscle protein degradation Major proteolytic systems Ubiquitin-proteasome system Autophagy-lysosomal system Basal protein degradation across fiber types Acute muscle atrophy & signaling mechanisms Atrophy from decreased use models Fiber type shift Fasting/starvation Major signals that regulate muscle atrophy Mechanisms of recovery from atrophy Satellite cells…required or not? Major intracellular proteolytic systems Ubiquitin-proteasome System Autophagy-lysosome System Calpain System Proteins (long-lived, aggregates) Organelles (mitochondrion, ER) Lipids and glycogen Caspases Poly-ubiquitinated proteins proteins (generally short-lived) The ubiquitin (Ub)-proteasome pathway (UPP) of protein degradation. Ex: E3 Ubiquitin- ligases specific to skeletal and heart muscles: - Atrogin1 - Murf1 Lecker S H et al. JASN 2006;17:1807-1819 ©2006 by American Society of Nephrology The ubiquitin (Ub)-proteasome pathway (UPP) of protein degradation. C2C12 myotube lysates Ebert et al, JBC 2012. MG132 – an inhibitor of the proteasome Autophagy The autophagosome Autophagosome - Macroautophagy. Conserved from yeast to mammals.. Requires the formation of a specialized vesicle…the autophagosome.. Degrades long-lived/misfolded proteins and organelles (e.g.,mitochondria). Mizushima et al, Nat Cell Biol 2010. Autophagy Atg7 ULKs Autophagy disruption leads to ubiquitinated protein accumulation in skeletal muscle Atg7 plays an essential role in the initiation of autophagy. Atg7 deletion causes a major disruption in the process. Ubiquitinated proteins accumulate upon autophagy deficiency in skeletal muscle. Yan…Lira, Sci Reports 2017. Skeletal Muscle Fibers Protein synthesis & degradation features Slow-twitch Fast -twitch (Type 2) Rodents MHC Type 1 2a 2x 2b Humans Protein synthesis +++ +++ ++ + Protein degradation +++ +++ ++ + Important: These are based on SO FOG FG “steady-state” levels of protein Plantaris (PL) synthesis and degradation (i.e., Soleus (SO) healthy, non-atrophying or White Vastus hypertrophying skeletal Lateralis (WV) muscle). Exercise Training Disuse/Detraining Mitochondrial content correlates with basal autophagy in muscles Mitochondrial biogenesis/content markers Lira et al, FASEB J, 2013 Protein synthesis assessment Heavy AA or “Heavy Water” Puromycin labeling Type IIa and Type I fibers have higher rates of protein synthesis than Type IIx and Iib fibers puromycin labeling puromycin labeling puromycin labeling Goodman et al. Novel insights into the regulation of skeletal muscle protein synthesis as revealed by a new nonradioactive in vivo technique. FASEB J 25, 1028-1039, 2011. Basal autophagy and proteasome activity are increased in postural/oxidative muscles - Oxidative fibers (Type I and IIa) have higher levels of baseline autophagy and proteasomal activity than glycolytic type IIx and IIb fibers. Proteasome - Higher levels of basal autophagy are required for Autophagy appropriate turnover of a larger mitochondrial content. Metabolic rate Lira et al., FASEB J 2013 Fernando et al., Exp Gerontol 2019 Balance between protein synthesis and degradation ultimately determines muscle mass Synthesis Net muscle mass Degradation Basal Atrophy Hypertrophy (steady-state) - Myofibrillar proteins (contractile filaments) account for 70-80% of total muscle protein. - Proteins are constantly being synthesized and degraded to maintain a functional protein pool (i.e., protein degradation and synthesis are constantly occurring). - Unbalances between protein synthesis and protein degradation will result either in muscle atrophy or hypertrophy. I. Major pathways involved in atrophy and recovery from atrophy Muscle protein degradation Major proteolytic systems Ubiquitin-proteasome system Autophagy-lysosomal system Basal protein degradation across fiber types Acurte muscle atrophy & signaling mechanisms Atrophy from decreased use models Fiber type shift Fasting/starvation Major signals that regulate muscle atrophy Mechanisms of recovery from atrophy Satellite cells…required or not? Models of muscle decreased use that lead to atrophy Denervation Immobilization Low gravity and hindlimb unloading (suspension) Muscle decreased use – slow twitch (postural) muscles atrophy the most - 8 weeks of hindlimb unloading causes a fast initial loss in muscle mass. Later muscle mass stabilizes. - This is because a new “steady- state” of muscle mass is reached and will only change if muscle use is brought back up. - The postural slow twitch soleus experiences a higher degree of atrophy than the mixed fiber plantaris muscle (both are plantar- flexors). Adapted from Thomason et al, 1987 Muscle decreased use – slow-to-fast fiber type switch - 1 year following spinal cord injury fast ATPase activity increases in fibers of a rat soleus muscle. - Dark fibers represent higher (fast) ATPase activity. - Overall atrophy of fibers is also evident. Lieber et al, 1986 Muscle decreased use – fibers become more glycolytic - 4 weeks of hindlimb unloading causes a significant decrease in succinate dehydrogenase activity (SDH activity), a marker of oxidative capacity of the muscle. - In the same context, glyceraldehyde phosphate dehydrogenase activity (GPD activity – a marker of glycolytic capacity, a.k.a. GAPDH) is either not affected or increased. Roy et al, 1987 Increased protein degradation and decreased protein synthesis lead to muscle atrophy in decreased use - Electromyogram (EMG) activity seems to drive the phenotype. - Initial robust decrease in EMG is followed by a robust increase in protein degradation and decrease in synthesis. - After ~8 days of hindlimb unloading, EMG activity is restored to normal and than increased by ~50%. - This makes protein degradation rates match protein synthesis levels. - Finally, EMG activity tends to go back to pre-suspension again and a new “steady-state” in net muscle protein content is reached. Adapted from Thomason et al, 1987 Lira Office Hours (week of 10/28) Via Zoom (link on announcement sent to all) Tues (10/29) – 2:15-3:15 pm Thurs (10/31) – 4-5 pm Fasting/starvation-induced muscle atrophy Acute & severe model of atrophy. Muscle provides AA for gluconeogenesis in the liver. Protein synthesis is dramatically reduced. Protein degradation is increased. Glucose‐Alanine Cycle ‐ skeletal muscle and liver - Mostly in fasting, but also in prolonged exercise, muscle tissue (a very large reservoir of AAs) is broken down into AAs and the carbon skeletons are used as fuel (in the form of glucose) – either (ALT) by muscle or other organs like the brain. - Because muscle cannot perform the urea cycle, glutamine is converted to glutamate, NH4+ is released, and the amino group left in glutamate is transferred to pyruvate forming alanine. - This reaction is catalyzed by alanine (ALT) aminotransferase (ALT). - Liver ALT performs the inverse reaction. Urea is formed and gluconeogenesis occur from pyruvate. Finally, glucose is released in the blood stream for muscle and other tissues to use. Fasting/starvation-induced muscle atrophy Protein synthesis drops in several tissues. Fasting/starvation-induced muscle atrophy - In muscle, the drop in protein synthesis and atrophy occurs primarily in fast-twitch fibers. - Contrary to disuse (decreased use)-induced muscle atrophy, fast twitch fibers atrophy the most during fasting. Goodman et al., Plos One 2012. Fasting/starvation-induced muscle atrophy AMPK p62 LC3-I LC3-II C F fed fasted fed fasted Plantaris muscle (overnight fast) Fasting model shown here: Several genes associated with the UPS and autophagy are also upregulated. Autophagy flux is increased (representative blots shown). Bongers et al, Am J Physiol Endocrinol Metab, 2013; Masiero et al, Cell Metabol 2009. Muscle atrophy caused by reduced use Markers of protein degradation are induced in all models of acute muscle atrophy. * * * * * * * * Denervation model shown here: Several genes associated with the UPS and autophagy are upregulated. Autophagy flux is increased (data not shown). Bongers et al, Am J Physiol Endocrinol Metab, 2013; O’Leary et al, Am J Physiol Cell Physiol 2012 Summary - The biochemical profile of atrophy is similar across different models of acute decreases in muscle use (e.g., immobilization, denervation and low gravity or unloading). - Protein synthesis rates decrease, and protein degradation increases. - Decreased muscle use causes atrophy of all muscle fiber types. However, slow-twitch muscles (postural muscles) atrophy the most. - Decreased use also causes a slow-to-fast fiber type switch. - Prolonged fasting or starvation, however, causes atrophy primarily of fast twitch fibers. In these conditions, atrophy in the muscle is required for survival. It provides alanine for liver gluconeogenesis. - No fiber type switching is observed with fasting/starvation. - Both the ubiquitin-proteasome system and autophagy appear to contribute to increased protein degradation in conditions of acute muscle atrophy due to decreased use and fasting. Signals for muscle atrophy - Muscle atrophy is driven primarily by increased muscle degradation rates and decreased protein synthesis rates. - Increased muscle degradation occurs due to increased transcription of genes involved in protein degradation. - Examples of atrophy genes are ubiquitin-ligases (required for UPS and Autophagy) – proteins that attach multiple ubiquitin peptides to proteins (e.g., atrogin-1, Murf1). - Ubiquitinated proteins are targeted to degradation. - Decreased protein degradation rates occur primarily due to decreased protein translation Signals for muscle atrophy Muscle activation (EMG) - Both ATF4 and FOXO are transcription factors that bind to the promoter region of genes and ATF4 FOXO stimulate the expression of that gene. - The expression of atrogenes (genes that encode proteins that are involved in protein degradation and atrophy) are stimulated by both ATF4 and FOXO. ATF4 FOXO Example: Autophagy genes, Atrogin-1 & Promoter Gene Promoter Gene MURF-1. ↑ Protein degradation Muscle atrophy Loss of ATF4 delays fasting- and immobilization-induced atrophy - Fasting for 24h and 48h (Panels A, B, C). - Immobilization for 3 and 7 days (Panels D, E, F). Ebert et al., J Biol Chem 2012. Loss of FOXO protects against fasting- and immobilization-induced atrophy - Immobilization for 7 days. - FOXO is also required for fasting-induced atrophy (data not shown). Senf et al., Am J Physiol Cell Physiol 2009. Loss of the atrogenes Atrogin-1 or Murf1 blunts muscle atrophy Atrogin1 +/+ Atrogin1 -/- - Experiment involving denervation of the gastrocnemius muscle in mice. - A significant part of the muscle atrophy process (40-60%) is prevented by knocking out each of these proteins in skeletal muscle. Note: Comparatively, much less is known about the degree to which autophagy (and different autophagy genes) contributes to atrophy in reduced use models. Adapted from Bodine et al, 2001 Disuse: muscle becomes less sensitive to stimuli of protein sysnthesis Growth Mechanical Factors Signals - mTORC1 – mammalian target of AA rapamycin complex 1 is a complex of proteins. Sarcolemma - mTOR acts by phosphorylating its downstream targets. AKT - Muscle fibers become anabolic resistant – resistant to growth stimuli (nutrients and hormones). mTORC1 ↑ Protein synthesis Fasting: muscle becomes more sensitive to stimuli of protein sysnthesis Growth Mechanical Factors Signals AA - mTORC1 – mammalian target of rapamycin complex 1 is a complex of Sarcolemma proteins. - mTOR acts by phosphorylating its downstream targets. AKT - Muscle fibers become more anabolic sensitive rapidly increasing protein synthesis when growth stimuli is mTORC1 present (nutrients and hormones). ↑ Protein synthesis I. Major pathways involved in atrophy and recovery from atrophy Muscle protein degradation Major proteolytic systems Ubiquitin-proteasome system Autophagy-lysosomal system Basal protein degradation across fiber types Acute muscle atrophy & signaling mechanisms Atrophy from decreased use Models Fiber type shift Fasting/starvation Major signals that regulate muscle atrophy Mechanisms of recovery from atrophy Satellite cells…required or not? Role of satellite cells in muscle regrowth - Muscle nuclear (myonuclear) domain is the ratio of cytosolic area-to-nucleus number in muscle fibers. - Myonuclear domain tends to decrease with atrophy. Examining the role of satellite cells in recovery from atrophy - 2 weeks of hindlimb unloading followed by 2 weeks of reloading - Soleus muscle was examined. Why? Jackson et al. Satellite cell depletion does not inhibit adult skeletal muscle regrowth Adapted from Thomason et al, 1987 following unloading-induced atrophy. Am J Physiol Cell Physiol 303, C854-C861, 2012. Role of satellite cells in muscle regrowth Mice expressing Pax7-cre (satellite cell specific) that activates the DTA transgene (diphteria toxin A chain) – once activated the toxin kills the satellite cells. Jackson et al. Satellite cell depletion does not inhibit adult skeletal muscle regrowth following unloading- induced atrophy. Am J Physiol Cell Physiol 303, C854-C861, 2012. Role of satellite cells in muscle regrowth Measurements of force performed in single fiber preparations. Jackson et al. Satellite cell depletion does not inhibit adult skeletal muscle regrowth following unloading- induced atrophy. Am J Physiol Cell Physiol 303, C854-C861, 2012. Signals for muscle atrophy and role of satellite cells in muscle regrowth - Summary Increased protein degradation is driven primarily by increased transcription of genes involved in both the ubiquitin-proteasome system and autophagy. Two major regulators of the increased gene transcription of atrophy-related genes are ATF4 and FOXO proteins. Satellite cells are required for normal increase in fiber type cross-sectional area in a chronic overload model in mice (hypertrophy over basal/normal muscle mass) but are not required for muscle mass recovery from atrophy caused by disuse (i.e., reduced use). Myonuclear domain tends to decrease with unloading, and the number of nuclei already present in the fiber seems to be sufficient to sustain the increased protein synthesis required for normal regrowth. For next class: - Read 2 papers posted on ICON: -Topic – Biological sex-dependent responses in muscle atrophy