Mechanics of Breathing (Part II) PDF
Document Details
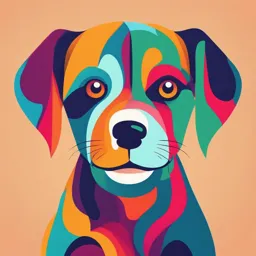
Uploaded by FruitfulIntegral
Wayne State University School of Medicine
Tags
Summary
These notes cover respiratory physiology, focusing on the mechanics of breathing. They detail chest wall compliance, lung and chest wall compliance, and dynamic lung compliance, including how airway resistance and geometry affect these processes. The content is suitable for undergraduate study.
Full Transcript
WSUSOM Medical Physiology – Respiratory Physiology Page | 13 of 20 Mechanics of Breathing Chest Wall Compliance The figure above shows the change in thoracic wall volume that occurs for changes in intrapleural pressure when the lungs are not present. ...
WSUSOM Medical Physiology – Respiratory Physiology Page | 13 of 20 Mechanics of Breathing Chest Wall Compliance The figure above shows the change in thoracic wall volume that occurs for changes in intrapleural pressure when the lungs are not present. When intrapleural pressure is zero, the relaxed volume is V1. The curve flattens at low volumes as the limits of wall collapse are reached. The slope of the curve at any point is the thoracic wall compliance. Factors that determine thoracic wall compliance include, elasticity of the chest wall tissue, respiratory muscle integrity, respiratory muscle innervation and obesity. WSUSOM Medical Physiology – Respiratory Physiology Page | 14 of 20 Mechanics of Breathing Lung and Chest Wall Compliance The diagram shows the compliance curves for the lung, chest wall, and lung + chest wall. Note several important points. (A) The chest wall determines the compliance limits of the respiratory system (lung + chest wall) at low lung volumes. (B) Functional residual capacity occurs when the expansion force of the chest wall opposes the recoil force of the lung. Thus, the opposing forces of the chest wall and lung are balanced and an equilibrium is reached at FRC. (C) When the limits imposed by the lung are removed the resting position of the chest wall is approximately 80 % of TLC. This means that under “normal” conditions the outward expansion force generated by the chest wall aids in inspiration. (D) When the system is intact, the minimum amount of gas in the lungs is the RV. When the limits imposed by the chest wall are removed, the lung may deflate further to minimal volume (E) The lung determines the compliance of the respiratory system at high volumes that are below the resting position of the chest wall (F) The lung and chest wall limit compliance of the system when volumes are above the resting position of the chest wall. If the subject increases lung volume above this point airway pressure increases. This is due to the greater inward recoil force of the lung. The chest wall, on the other hand, is closer to where it wants to be and thus contributes less outward elastic recoil force then at FRC. WSUSOM Medical Physiology – Respiratory Physiology Page | 15 of 20 Mechanics of Breathing Dynamic Lung Compliance and Airway Resistance In the previous discussion, we considered the static lung and chest wall compliance (i.e. when air is not moving). The factors principally responsible for these measures are the elastic properties of the lung and chest wall, and surface tension. However, we must consider compliance of the lung and chest wall when air is moving (i.e. dynamic compliance). The additional factors that influence the dynamic compliance measures are airway resistance, tissue resistance and inertia. Of these three, airway resistance is quantitatively the most important. The above diagram shows simultaneous changes in lung volume, intrapleural pressure, gas flow, and alveolar pressure during normal inspiration and expiration. The graph of intrapleural pressure shows the actual pleural pressure (AB`C), and the pleural pressure required to overcome only the elastic characteristics of the lung (ABC). The difference in pressures is due to the additional work expended to overcome resistance to air flow. Airway resistance can be calculated using Poiseuille’s Law. This law describes the pressure-flow characteristics for laminar flow in a tube: V = Pπr4 8ηl where P is the pressure, r is the tube radius, η is fluid viscosity and l is tube length. Since resistance is driving pressure divided by flow then we can arrange this equation to calculate resistance: R = 8ηl πr4 As the equation shows Poiseulle’s law predicts that resistance to laminar flow (of air or a Newtonian fluid) is directly proportional to viscosity and tube length and inversely proportional to tube radius. Therefore, small changes in tube radius can have large effects on resistance. There are a number of factors that influence airway resistance and these are discussed on the following pages. WSUSOM Medical Physiology – Respiratory Physiology Page | 16 of 20 Mechanics of Breathing Factors that determine airway resistance - Airway geometry The conducting zone consists of right and left primary bronchi that enter each lung and diverge into secondary bronchi that serve each lobe of the lungs (Fig. on the right). Secondary bronchi branch into several orders of tertiary bronchi, which ultimately branch into bronchioles. As the conducting airways become smaller, the supportive cartilage changes in character until it is no longer present in the bronchioles. The initial 16 generations strictly conduct air while the final 7 generations (respiratory zone) are involved in gas exchange. The chief site of airway resistance is located in the intermediate-sized bronchi. Because of the increase in cross-sectional area the small airways contribute relatively little to airway resistance. WSUSOM Medical Physiology – Respiratory Physiology Page | 17 of 20 Mechanics of Breathing Factors that determine airway resistance - Lung Volume The graph on the left show the relationship between airway resistance and lung volume. As lung volume increases airway resistance decreases and conductance (i.e. the reciprocal of resistance) increases. The reduction in resistance occurs primarily because increasing lung volume provides radial traction to the surrounding lung tissue (see figure on the right), which decreases the possibility that the airway would collapse leading to an increase in airway resistance. WSUSOM Medical Physiology – Respiratory Physiology Page | 18 of 20 Mechanics of Breathing Factors that determine airway resistance – Dynamic compression of the airway Another important modifier of airway diameter and consequently resistance is dynamic compression of airways. Dynamic compression results in flow limitation during expiration. Panel C shows various pressures within the tracheobronchial tree during a forced vital capacity maneuver (this maneuver is completed by filling the lungs to total lung capacity and subsequently expiring forcefully to residual volume). During expiration, lung volume is changing from total lung capacity to residual volume. Panel C shows the pressures recorded as the lung is passing through functional residual capacity. At this point in time, the alveolar pressure is + 15 cmH2O and the intrapleural pressure is + 10 cmH2O. Thus, at the level of the alveoli the pressure across the alveoli is 15 – 10 cmH2O = 5 cmH2O. However, note that as we move from the level of the alveoli to the upper regions of the tracheobronchial tree the pressure within the airway is decreasing (15 to 12 to 8 to 4). These pressure changes occur for two primary reasons. First, drops in pressure occur in response to increases in resistance which occurs as we move from the bottom to the top of the tracheobronchial tree in healthy individuals. Second, the Bernoulli principle may also contribute to the progressive decrease in airway pressure. For an ideal fluid with no viscosity, as the linear velocity of the fluid flow increases, the pressure exerted by the fluid on the walls of the vessel (the “lateral pressure”) decreases. Therefore, as the velocity of airflow in the small compressible airways increases during a forced expiration, the pressure inside the vessel decreases. Independent of the mechanism the end result is that at some point a collapsing pressure may exist across the airway. In the example shown in panel C, the collapsing pressure occurs when the airway pressure has decreased to 8 cmH2O (i.e. 8 – 10 cmH2O = - 2 cmH2O). As a result of the dynamic compression, airway resistance increases significantly. Dynamic compression typically will only occur at lower lung volumes in healthy individuals. However, in individuals with chronic obstructive pulmonary disease, dynamic compression of the small airways may occur at higher lung volumes during normal breathing. The reason is that lung elasticity is severely compromised so that radial traction of the small airways is mitigated. This lack of radial traction coupled with hyperactive smooth muscle leads to elevated resistance in the small airway. Consequently, significant drops in pressure in the small airway leads to dynamic compression even at high lung volumes. To counteract this mechanism individuals with chronic obstructive pulmonary disease will often breath with pursed lips (Panel D). This maneuver is completed in an attempt to ensure that the greatest point of resistance is closer to the top of the tracheobronchial tree rather than at the level of the small airway. WSUSOM Medical Physiology – Respiratory Physiology Page | 19 of 20 Mechanics of Breathing Factors that determine airway resistance - Airway Smooth Muscle Tone Airway resistance is altered by a variety of endogenous mediators. Airway smooth muscle tone is affected by noxious stimuli that activate receptors located beneath the epithelium of smooth muscle. Axons from these receptors travel to the medulla via the vagus nerve. Consequently, efferent fibers within the vagus nerve will be stimulated and cause bronchial smooth muscle constriction. The parasympathetic nervous system mediates the reflex modulated by the release of acetylcholine at muscarinic receptors on the muscle cell. Parasympatholytic drugs (i.e. atropine or glycopyrrolate) may block the efferent response to stimulation of this reflex arc. The sympathetic system (shown to the far right in the above diagram) plays a role in modulating bronchial smooth muscle tone, and some direct innervation is present as shown in the diagram. However, the presence of circulating catecholamines is probably more important to airway resistance as direct innervation is not extensive in most species. Agonists at the beta-2 receptor are used therapeutically to treat bronchoconstriction (i.e. albuterol, clenbuterol). The nonadrenergic noncholinergic system may also play a role in modulating smooth muscle tone, and vasoactive intestinal peptide (VIP) has been shown to be a bronchodilator. Nitric oxide may also play a role in modulation of bronchial smooth muscle tone. A variety of inflammatory mediators can alter airway tone. Histamine and serotonin are two mediators that can be released from mast cells in response to IgE-mediated degranulation of mast cells. Lipid mediators {leukotrienes (LTB4), sulfidopeptide leukotrienes (LTC4, LTD4, LTE4)} and prostaglandins are also released. WSUSOM Medical Physiology – Respiratory Physiology Page | 20 of 20 Mechanics of Breathing Factors that Determine Airway Resistance – Airflow Patterns Airflow changes from laminar to turbulent flow when airflow velocity is increased. During normal tidal breathing airflow is turbulent in the upper airways while flow is typically laminar in the small airways (< 2 mm internal diameter). Transitional flow may also be present, with turbulent flow evident at the points of bifurcation. During turbulent flow, particles move in irregular and constantly varying paths forming eddies. Resistance to airflow increases in response to turbulent flow. Flow is turbulent when Reynold’s number is greater than 2000. Reynolds number = 2rvd where r is radius, v is average air flow velocity, d is density and η = viscosity. Turbulent airflow generates breath sounds while laminar flow is essentially noiseless.