Cellular Respiration Overview PDF
Document Details
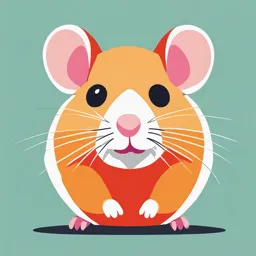
Uploaded by DazzledDalmatianJasper
Tags
Summary
This document provides an overview of cellular respiration, covering key concepts such as glycolysis, the citric acid cycle, and oxidative phosphorylation. It explains the energy transfer processes in detail and includes diagrams. The document likely serves as study material for students in a biology or biochemistry course.
Full Transcript
Aerobic respiration Possible once plants covered the Earth – filling the atmosphere with oxygen, which is a product of photosynthesis Occurs in mitochondria (eukaryotic cell organelle) (endosymbiotic theory proposes that mitochondria were once separate organisms incorporated into eukaryot...
Aerobic respiration Possible once plants covered the Earth – filling the atmosphere with oxygen, which is a product of photosynthesis Occurs in mitochondria (eukaryotic cell organelle) (endosymbiotic theory proposes that mitochondria were once separate organisms incorporated into eukaryotes specifically for their respiratory abilities) Key principles Pyruvate is the metabolite that links two central catabolic pathways, glycolysis and the citric acid cycle The reactions of the citric acid cycle follow a chemical logic The TCA cycle is a hub of metabolism, with catabolic pathways leading in and anabolic pathways leading out The central role of the citric acid cycle in metabolism requires that it be regulated in coordination with many other pathways Enzymes have evolved to form complexes to efficiently achieve a series of chemical transformations without realeasing the intermediates into the bulk solvent Pyruvate oxidation to Acetyl CoA In the presence of O2, pyruvate enters the mitochondrion (in eukaryotic cells) where the oxidation of glucose is completed Before the citric acid cycle can begin, pyruvate must be converted to acetyl Coenzyme A (acetyl CoA), which links glycolysis to the citric acid cycle This step is carried out by a multienzyme complex that catalyses three reactions: 1) Decarboxylation 2) Oxidation by NAD+ 3) Attachment to CoA Coenzyme A Has a reactive thiol (-SH) group that is critical to its role as an acyl carrier (R−C=O) Pyruvate is oxidized to Acetyl-CoA and CO2 Mitochondrial pyruvate carrier (MPC) – an H+ coupled pyruvate- specific symporter in the inner mitochondrial membrane (carries 2 items at the same time) Pyruvate dehydrogenase (PDH) complex – highly ordered cluster of enzymes and cofactors that oxidizes pyruvate in the m. matrix to Acetyl CoA and CO2 Three enzymes (E1-3) Five coenzymes (TPP - thyamine pyrophosphate – B1 derivative), lipoate, CoA, FAD, NAD) PDH complex The Citric Acid Cycle (Krebs cycle, Tricarboxylic acid cycle) The citric acid cycle, also called the Krebs cycle, completes the break down of pyruvate to CO2 extracts the max potential energy The cycle oxidizes organic fuel derived from pyruvate, generating 1 ATP, 3 NADH, and 1 FADH2 per turn Krebs cycle The citric acid cycle has eight steps, each catalyzed by a specific enzyme The acetyl group of acetyl CoA joins the cycle by combining with oxaloacetate, forming citrate The next seven steps decompose the citrate back to oxaloacetate, making the process a cycle The NADH and FADH2 produced by the cycle relay electrons extracted from food to the electron transport chain Citrate synthase Malate dehydrogenase Aconitase Fumarase Isocitrate dehydrogenase Succinate dehydrogenase Ketoglutarate dehydrogenase Succinyl-CoA synthase Overall yield of energy – Krebs cycle The TCA cycle directly generates only one ATP per turn The large flow of electrons into the respiratory chain via NADH and FADH2 leads to formation of almost 10x more ATP during oxidative phosporylation For every Acetyl-CoA , there is: 3 NADH, FADH2 and ATP Per glucose molecule it is x 2 Each NADH drives formation of ~ 2.5 ATP Each FADH2 about ~ 1.5 ATP ATP formation in the aerobic oxidation of glucose TCA cycle accepts carbon skeletons The cycle accepts 3-, 4-, 5- carbon skeletons The breakdown of amino acids yields carbon skeletons: Deaminated aspartate yields oxaloacetate Deaminated glutamate yields alpha-ketoglutareate (more during protein metabolism) TCA and metabolic pathways Regulation of TCA cycle Regulation balances the supply of key intermediates with the demands of energy production and biosynthetic processes Regulation occurs at several points: PDH complex On – energy demand.. Off – lots of fatty acids and acetyl-CoA as fuel Lost of ATP and NADH Citrate synthase Isocitrate dehydrogenase complex Alpha-ketoglutarete dehydrogenase complex Oxidative phosphorylation Following glycolysis and the citric acid cycle, NADH and FADH2 account for most of the energy extracted from food These two electron carriers donate electrons to the electron transport chain, which powers ATP synthesis via oxidative phosphorylation During oxidative phosphorylation, chemiosmosis couples electron transport to ATP synthesis https://www.youtube.com/watch?v=LQm TKxI4Wn4 Electrons are transferred from NADH or FADH2 to the electron transport chain Electrons are passed through a number of proteins including cytochromes (each with an iron atom) to O2 The electron transport chain generates no ATP directly The flow of electrons can do biological work Electromotive force (emf) – force proportional to the difference in electron affinity between chemical species drives electron flow spontaneously through a circuit Biological circuit – electrons from glucose flow through a series of electron-carrier intermediates to another chemical species, such as O2 Chemiosmosis: The Energy-Coupling Mechanism Electron transfer in the electron transport chain causes proteins to pump H+ from the mitochondrial matrix to the intermembrane space H+ then moves back across the membrane, passing through ATP synthase ATP synthase uses the flow of H+ to drive phosphorylation of ATP This is an example of chemiosmosis, the use of energy in a H+ gradient to drive cellular work The energy stored in a H+ gradient across a membrane couples the redox reactions of the electron transport chain to ATP synthesis The H+ gradient is referred to as a proton-motive force, emphasizing its capacity to do work https://www.youtube.com/watch?v=kXpzp4RDGJI An Accounting of ATP Production by Cellular Respiration During cellular respiration, most energy flows in this sequence: glucose NADH electron transport chain proton-motive force ATP About 34% of the energy in a glucose molecule is transferred to ATP during cellular respiration, making about 32 ATP There are several reasons why the number of ATP is not known exactly In the last stage of respiration, some protons leak through the inner mitochondrial membrane back inside the matrix, so proton motive force which spins the headpiece of ATP synthase to produce ATP is decreased, hence less ATP produced Summary Glycolysis and the citric acid cycle connect to many other metabolic pathways Gluconeogenesis A process by which the body maintains euglycemia by producing glucose from NON-CARBOHYDRATE sources which?? Occurs mainly in the liver and kidney in both the cytosol and mitochondria Occurs during fasting Note: skeletal muscle cannot do it due to the absence of Glucose-6- phosphatase Gluconeogenesis Glycogenolysis and Glycogenesis Glycogenolysis – the breakdown of cellular glycogen to glucose –1 phosphate Glycogen phosphorylase Phoshoglucomutase – conversion to glu 6-p In muscle enters glycolysis In liver back to glucose – blood glucose (Glucose-6-phosphatase) Glycogenesis – the synthesis of glycogen Begins with glu 6-p from glucose or lactate (lactate in the liver is converted to glucose 6-phosphate by gluconeogenesis Phosphoglucomutase – conversion to glu 1 p Endogenous Glycogen and Starch are Degraded by Phosphorolysis Glycogen phosphorylase – mobilizes glycogen stored in animal tissues by a phosphorylation reaction to yield glucose 1-phosphate (vs. starch phosphorylase) Phosphoglycomutase – catalyzes the reversible reaction glucose-1 phosphate to glucose-6-phosphate (can continue through glycolysis) Mutase – enzyme that catalyzes the transfer of a functional group from one position to another in the same molecule Other carbs are hydrolysed by digestive enzymes into their monosaccharide units and then transported from the small intestine to the blood and then to liver and other organs