Basic Principles of Stratigraphy PDF
Document Details
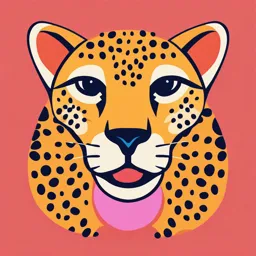
Uploaded by ManageableConcreteArt
University of Ilorin
Tags
Summary
This document provides a detailed overview of stratigraphy, a branch of geology focusing on the formation, composition, sequence, and correlation of stratified rocks. It covers basic principles, historical context, and different types of rocks, including igneous, sedimentary, and metamorphic.
Full Transcript
COURSE: GEM 105 BASIC PRINCIPLES OF STRATIGRAPHY Stratigraphy is a branch of geology that deals with formation, composition, sequence, and correlation of stratified rocks. Since the whole Earth is stratified, at least in a broad sense, bodies of all the different types of rocks - igneous, sedimenta...
COURSE: GEM 105 BASIC PRINCIPLES OF STRATIGRAPHY Stratigraphy is a branch of geology that deals with formation, composition, sequence, and correlation of stratified rocks. Since the whole Earth is stratified, at least in a broad sense, bodies of all the different types of rocks - igneous, sedimentary, metamorphic - are subject to stratigraphic study and analysis. In most cases however, stratigraphy focuses on the evaluation of sedimentary rock strata. Sedimentary rocks provide the most complete record of the earth history and accounts for about 75% of all exposed rocks. Sedimentary rocks are types of rock that are formed by the accumulation or deposition of mineral or organic particles at the Earth's surface, followed by cementation. Sedimentation is the collective name for processes that cause these particles to settle in place. The particles that form a sedimentary rock are called sediment, and may be composed of geological detritus (minerals) or biological detritus (organic matter). The study of the sequence of sedimentary rock strata is the main source for an understanding of the Earth's history, including palaeogeography, paleoclimatology and the history of life. Modern principles of stratigraphic analysis were worked out in the 18th and 19th centuries by geologists such as Niels Stensen, James Hutton, Georges Cuvier, William Smith and Charles Lyell. By 1900 all the intellectual tools needed to establish the description, sequence, and correlation of strata were in place. The study of stratification therefore involves the assessment of TIME and SPACE. Specifically, the vertical arrangement of layers represents the time dimension (oldest at the bottom, youngest at the top), while the horizontal (lateral) distribution of layers represent the space dimension (i.e. spatial variations in the stratigraphy). 1 With the vertical (time) dimension, stratigraphy is often used as a relative dating technique to assess the temporal sequence of sediment deposition according to law of Superposition. History of Stratigraphy Nicholas Steno established the theoretical basis for stratigraphy when he introduced the law of superposition, the principle of original horizontality and the principle of lateral continuity in a 1669 work on the fossilization of organic remains in layers of sediment. The first practical large-scale application of stratigraphy was by William Smith in the 1790s and early 19th century. William 'Strata' Smith (23 March 1769 – 28 August 1839) was an English geologist and surveyor who generated the first geological map of England. Known as the "Father of English geology", Smith recognized the significance of strata or rock layering and the importance of fossil markers for correlating strata. Other influential applications of stratigraphy in the early 19th century were by Georges Cuvier and Alexandre Brongniart, who studied the geology of the region around Paris. Principles of Stratigraphy Stratigraphy is the study of strata (sedimentary layers) in the Earth's crust. Geologist in the 1800s worked out 7 basic principles of stratigraphy that allowed them, and now us, to work out the relative ages of rocks. Once these age relations were worked out, another principle fell into place - the principle of fossil succession. Principle of Uniformitarianism The principle of Uniformitarianism was postulated by James Hutton (1726-1797) who examined rocks in Scotland and noted that features like mudcracks, ripple marks, graded bedding, etc. where the same features that could be seen forming in 2 modern environments. He concluded that process that are currently operating on the Earth must be the same processes that operated in the past. This principle is often stated as "the present is the key to the past". A more modern way of stating the same principle is that the laws of nature (as outlined by the laws of chemistry and physics) have operated in the same way since the beginning of time, and thus if we understand the physical and chemical principles by which nature operates, we can assume that nature operated the same way in the past. The principle of uniformitarianism is essential to understanding Earth’s history. However, prior to 1830, uniformitarianism was not the prevailing theory. Until that time, scientists subscribed to the idea of catastrophism. Catastrophism suggested the features seen on the surface of Earth, such as mountains, were formed by large, abrupt changes—or catastrophes. When discussing past climates, opponents to uniformitarianism may speak of no-analog changes. This idea suggests that certain communities or conditions that existed in the past may not be found on Earth today. The idea of catastrophism was eventually challenged based on the observations and studies of two men—James Hutton and Charles Lyell. Hutton (1726–1797) was a Scottish farmer and naturalist. In his observations of the world around him, he became convinced natural processes, such as mountain building and erosion, occurred slowly over time through geologic forces that have been at work since Earth first formed. He eventually turned his observations and ideas into what became known as the Principle of Uniformitarianism. Among the scientists who agreed with Hutton was Charles Lyell. Lyell (1797–1875) was a Scottish geologist. In 1830, he published a book, Principles of Geology, that challenged the idea of catastrophism, which was still the dominant theory despite Hutton’s work. Lyell believed Hutton was correct about the gradually changing processes shaping Earth’s surface. He found his own examples of these processes in his examination of rocks and sediments. 3 Principle of Superposition The principle of superposition was first proposed in 1669 by the Danish scientist Nicolas Steno. Between the years of 1785 and 1800, James Hutton and William Smith advanced the concept of geologic time and strengthened the belief in an ancient world. Hutton, a Scottish geologist, first proposed formally the fundamental principle used to classify rocks according to their relative ages. He concluded, after studying rocks at many outcrops, that each layer represented a specific interval of geologic time. Further, he proposed that wherever uncontorted layers were exposed, the bottom layer was deposited first and was, therefore, the oldest layer exposed; each succeeding layer, up to the topmost one, was progressively younger. Because of Earth's gravity, deposition of sediment will occur depositing older layers first followed by successively younger layers. Thus, in a sequence of layers that have not been overturned by a later deformational event, the oldest layer will be on the bottom and the youngest layer on top. Principle of Original Horizontality Sedimentary strata are deposited in layers that are horizontal or nearly horizontal, parallel to or nearly parallel to the Earth's surface. Sediment deposited on steep slopes will be washed away before it is buried and lithified to become sedimentary rock, but sediment deposited in nearly horizontal layers can be buried and lithified. Thus rocks that we now see inclined or folded have been disturbed since their original deposition. Principle of Original Continuity If layers are deposited horizontally over the sea floor, then they would be expected to be laterally continuous over some distance. Thus, if the strata are later uplifted and then cut by a canyon, we know that the same strata would be expected to occur on both sides of the canyon. 4 Look at the many photographs of the Grand Canyon in your textbook. Note that you can follow the layers all along the walls of the canyon, and you can find the same layers on both sides of the canyon. The Grand Canyon is particularly good for this because different sedimentary rocks have different colors. Principle of Cross-cutting Relations Younger features truncate (cut across) older features. Faults, dikes, erosion, etc., must be younger than the material that is faulted, intruded, or eroded. Figure 1: Schematic diagram showing an example of cross cutting relation. For example, the mudstone, sandstone and shale are cut by the basalt dike, so we know that the mudstone, sandstone, and shale had to be present before the intrusion of the basalt dike. Thus, we know that the dike is younger than the mudstone, sandstone, and shale. Similarly, the rhyolite dike cuts only the mudstone and the sandstone, but does not cut across the shale. Thus, we can deduce that the mudstone and sandstone are older than the rhyolite dike. But, since the rhyolite dike does not cut across the shale, we know the shale is younger than the rhyolite dike. In the diagram below, the fault cuts the limestone and the sandstone, but does not cut the basalt. Thus we know that the fault is younger than the limestone and shale, but older than the basalt above. 5 Figure 2: Schematic diagram showing an example of cross cutting relation with the presence of a fault Principle of Inclusions If we find a rock fragment enclosed within another rock, we say the fragment is an inclusion. If the enclosing rock is an igneous rock, the inclusions are called xenoliths. In either case, the inclusions had to be present before they could be included in the younger rock, therefore, the inclusions represent fragments of an older rock. Figure 3: Schematic diagram showing the principle of inclusion. In the example here, as the basalt flowed out on the surface it picked up inclusions of the underlying sandstone. So we know the sandstone is older than the basalt flow. Similarly, the overlying rhyolite flow contains inclusions of the basalt, so we know that the basalt is older than the rhyolite. This principle is often useful for distinguishing between a lava flow and a sill. (Recall that a sill is intruded between 6 existing layers). In the case shown below, we know that the basalt is a sill because it contains inclusions of both the underlying rhyolite and the overlying sandstone. This also tells us that the sill is younger than the both the rhyolite and the sandstone. Figure 4: Schematic diagram showing the principle of inclusion. Fossil Succession Once geologists had worked the relative ages of rocks throughout the world, it became clear that fossils that were contained in the rock could also be used to determine relative age. It was soon recognized that some fossils of once living organisms only occurred in very old rocks and others only occurred in younger rocks. Furthermore, some fossils were only found within a limited range of strata and these fossils, because they were so characteristic of relative age were termed index fossils. With this new information, in combination with the other principles of stratigraphy, geologists were able to recognize how life had changed or evolved throughout Earth history. This recognition led them to the principle of fossil succession, which basically says that there is a succession of fossils that relate to the age of the rock. Stratigraphic classification Stratigraphic classification encompasses all rocks of the crust of the Earth. Rocks have many tangible and measurable properties and may be classified according to any of them. Rocks may also be classified by their time of origin or interpreted attributes, such as environment or genesis. 7 The stratigraphic position of change for any property or attribute does not necessarily coincide with that for any other. Consequently, units based on one property commonly do not coincide with those based on a different property. Therefore, it is not possible to express the distributions in the rocks of all of the different properties with a single set of stratigraphic units. Different sets of units are needed. However, all the different classifications are closely related because they express different aspects of the same rock bodies and they are used to achieve the same goals of stratigraphy: to improve our knowledge and understanding of the Earth’s rock bodies and their history. Categories of Stratigraphic Classification Rock bodies may be classified according to many different inherent properties. Each classification needs its own distinctive nomenclature. The following kinds of formal units are best known and most widely used: Lithostratigraphic units: units based on the lithologic properties of the rock bodies. Unconformity-bounded units: bodies of rock bounded above and below by significant discontinuities in the stratigraphic succession. Biostratigraphic units: units based on the fossil content of the rock bodies. Magnetostratigraphic polarity units: units based on changes in the orientation of the remnant magnetization of the rock bodies. Chronostratigraphic units: units based on the time of formation of the rock bodies. Many other properties and attributes may be used to classify rock bodies and the way is open to use any that show promise. Whenever this is the case, the unit-terms being used should be defined. Though each kind of stratigraphic unit may be particularly useful in stratigraphic classification under certain conditions or in certain areas or for certain purposes, chronostratigraphic units offer the greatest promise for formally-named units of worldwide application because they are based on their time of formation. 8 Lithostratigraphic, biostratigraphic, and unconformity-bounded units are all of limited areal extent, and thus unsatisfactory for global synthesis. Magnetostratigraphic polarity units, though potentially worldwide in extent, require extrinsic data from the other units for their recognition, and dating. For these reasons, chronostratigraphic units have been chosen for international communication among stratigraphers with respect to position in the stratigraphic column. Lithostratigraphic Unit Sequences of sedimentary and volcanic rocks are subdivided on the basis of their shared or associated lithology. Formally identified lithostratigraphic units are structured in a hierarchy of lithostratigraphic rank, higher rank units generally comprising two or more units of lower rank. Going from smaller to larger in rank, the main lithostratigraphic ranks are Bed, Member, Formation, Group and Supergroup. Formal names of lithostratigraphic units are assigned by geological surveys. Units of formation or higher rank are usually named for the unit's type location, and the formal name usually also states the unit's rank or lithology. A lithostratigraphic unit may have a change in rank over some distance; a group may thin to a formation in another region and a formation may reduce in rank for member or bed as it "pinches out". Bed A bed is a lithologically distinct layer within a member or formation and is the smallest recognisable stratigraphic unit. These are not normally named, but may be in the case of a marker horizon. Beds are the layers of sedimentary rocks that are distinctly different from overlying and underlying subsequent beds of different sedimentary rocks. Layers of beds are called strata. They are formed from sedimentary rocks being deposited on the Earth's solid surface over long periods of time. The strata are layered in the same order that they were deposited, permitting discrimination as to which beds are younger and which ones are older (the law of 9 superposition). The structure of a bed is determined by its bedding plane, the surface that separates two layers. Beds can be differentiated in various ways, such as by particle size or rock or mineral type. The term is generally applied to sedimentary strata, but may also be used for volcanic flows or ash layers. Types of beds include cross-beds and graded beds. Cross-beds, or "sets," are not layered horizontally and are formed by a combination of local deposition on the inclined surfaces of ripples or dunes, and local erosion. Graded beds show a gradual change in grain or clast sizes from one side of the bed to the other. A normal grading occurs where there are larger grain sizes on the older side, while an inverse grading occurs where there are smaller grain sizes on the older side. By determining the type of beds, geologists are able to determine the relative ages of the rocks Member A member is a named lithologically distinct part of a formation. Not all formations are subdivided in this way and even where they are recognized, they may only form part of the formation. A member need not be mappable at the same scale as a formation. Formation Formations are the primary units used in the subdivision of a sequence and may vary in scale from tens of centimetres to kilometres. They should be distinct lithologically from other formations, although the boundaries do not need to be sharp. To be formally recognised, a formation must have sufficient extent to be useful in mapping an area. A geological formation, or formation, is a body of rock having a consistent set of physical characteristics (lithology) that distinguish it from adjacent bodies of rock, and which occupies a particular position in the layers of rock exposed in a geographical region (the stratigraphic column). It is the fundamental unit of lithostratigraphy, the study of strata or rock layers. 10 A formation must be large enough that it can be mapped at the surface or traced in the subsurface. Formations are otherwise not defined by the thickness of their rock strata, which can vary widely. They are usually, but not universally, tabular in form. They may consist of a single lithology (rock type), or of alternating beds of two or more lithologies, or even a heterogeneous mixture of lithologies, so long as this distinguishes them from adjacent bodies of rock. Group A group is a set of two or more formations that share certain lithological characteristics. A group may be made up of different formations in different geographical areas and individual formations may appear in more than one group. A group is a lithostratigraphic unit, a part of the geologic record preserved in rock strata. Groups are generally divided into individual formations. Groups may sometimes be combined into supergroups. Groups are useful for showing relationships between formations, and they are also useful for small-scale mapping or for studying the stratigraphy of large regions. Geologists exploring a new area have sometimes defined groups when they believe the strata within the groups can be divided into formations during subsequent investigations of the area. It is possible for only some of the strata making up a group to be divided into formations. Supergroup A supergroup is a set of two or more associated groups and/or formations that share certain lithological characteristics. A supergroup may be made up of different groups in different geographical areas. Biostratigraphic units A sequence of fossil-bearing sedimentary rocks can be subdivided on the basis of the occurrence of particular fossil taxa. A unit defined in this way is known as a 11 biostratigraphic unit, generally shortened to biozone. The five commonly used types of biozone are assemblage, range, abundance, interval and lineage zones. An assemblage zone is a stratigraphic interval characterised by an assemblage of three or more coexisting fossil taxa that distinguish it from surrounding strata. A range zone is a stratigraphic interval that represents the occurrence range of a specific fossil taxon, based on the localities where it has been recognised. An abundance zone is a stratigraphic interval in which the abundance of a particular taxon (or group of taxa) is significantly greater than seen in neighboring parts of the succession. An interval zone is a stratigraphic interval whose top and base are defined by horizons that mark the first or last occurrence of two different taxa. A lineage zone is a stratigraphic interval that contains fossils that represent parts of the evolutionary lineage of a particular fossil group. This is a special case of a range zone. Facies Concept. Facies is a body of rock characterized by a particular combination of lithology, physical and biological structures that bestow an aspect that are different from the bodies of rock above, below and laterally adjacent. The facies concept refers to the sum of characteristics of a sedimentary unit, commonly at a fairly small (cm-m) scale including: Lithology Grain size Sedimentary structures Color Composition Biogenic content 12 If the facies description is confined to the physical and chemical characteristics of a rock this is referred to as the lithofacies. In cases where the observations concentrate on the fauna and flora present, this is termed as biofacies. A study that focuses on the trace fossils in the rock would be a description of the ichnofacies. The facies concept is not just a convenient means of describing rocks and grouping sedimentary rocks seen in the field, it also forms the basis for facies analysis. By interpreting the sediment in terms of the physical, chemical and ecological conditions at the time of deposition it becomes possible to reconstruct palaeoenvironments, i.e. environments of the past. So, from the presence of symmetrical ripple structures in a fine sandstone it can be deduced that the bed was formed under shallow water with wind over the surface of the water creating waves that stirred the sand to form symmetrical wave ripples. The ‘shallow water’ interpretation is made because wave ripples do not form in deep water but the presence of ripples alone does not indicate whether the water was in a lake, lagoon or shallow-marine shelf environment. The facies should therefore be referred to as ‘symmetrically rippled sandstone’ or perhaps ‘wave rippled sandstone’, but not ‘lacustrine sandstone’ because further information is required before that interpretation can be made. Presence of cross bedded sandstone can form during deposition in deserts, rivers, deltas, lakes, beaches and shallow marine In contrast, present of hermatypic corals indicate that the sediments were deposited in shallow clear and warm seawater. 13 Figure 5: Depositional environment of clastic and non-clastic sedimentary rocks Unconformities - Breaks in the Stratigraphic Record Because the Earth's crust is continually changing, i.e. due to uplift, subsidence, and deformation, erosion is acting in some places and deposition of sediment is occurring in other places. When sediment is not being deposited, or when erosion is removing previously deposited sediment, there will not be a continuous record of sedimentation preserved in the rocks. We call such a break in the stratigraphic record a hiatus. When we find evidence of a hiatus in the stratigraphic record we call it an unconformity. An unconformity is a surface of erosion or non-deposition. Three types of unconformities are recognized. 14 Angular Unconformity Figure 6: Schematic diagram showing the development of an angular unconformity. Because of the Principles of Stratigraphy, if we see a cross section like this in a road cut or canyon wall where we can recognize an angular unconformity, then we know the geologic sequence of events that must have occurred in the area to produce the angular unconformity. Angular unconformities are easy to recognize in the field because of the angular relationship of layers that were originally deposited horizontally. 15 Nonconformity Figure 7: Schematic diagram showing the development of a nonconformity. Nonconformities occur where rocks that formed deep in the Earth, such as intrusive igneous rocks or metamorphic rocks, are overlain by sedimentary rocks formed at the Earth's surface. The nonconformity can only occur if all of the rocks overlying the metamorphic or intrusive igneous rocks have been removed by erosion. Disconformity Figure 8: Schematic diagram showing the development of a disconformity. Disconformities are much harder to recognize in the field, because often there is no angular relationship between sets of layers. Disconformity are usually recognized by 16 correlating from one area to another and finding that some strata is missing in one of the areas. Disconformities can also be recognized if features that indicate a pause in deposition, like paleosols (ancient soil horizons), or erosion, like stream channels are present. Variation in Unconformities The nature of an unconformity can change with distance. Notice how if we are only examining a small area in the figure above, we would determine a different type of unconformity at each location, yet the unconformity itself was caused by the same erosional event. Correlation Correlation is the process of establishing which sedimentary strata are of the same age but geographically separated. Correlation can be determined by using magnetic polarity reversals, rock types, unique rock sequences, or index fossils. There are four main types of correlation: stratigraphic, lithostratigraphic, chronostratigraphic, and biostratigraphic. Stratigraphic Correlation Stratigraphic correlation is the process of establishing which sedimentary strata are the same age at distant geographical areas by means of their stratigraphic relationship. Geologists construct geologic histories of areas by mapping and making stratigraphic columns-a detailed description of the strata from bottom to top. An example of stratigraphic relationships and correlation between Canyonlands National Park and Zion National Park in Utah. At Canyonlands, the Navajo Sandstone overlies the Kayenta Formation which overlies the cliff-forming Wingate Formation. In Zion, the Navajo Sandstone overlies the Kayenta formation which overlies the cliff-forming Moenave Formation. Based on the stratigraphic relationship, the Wingate and Moenave Formations correlate. These two formations 17 have unique names because their composition and outcrop pattern is slightly different. Lithostratigraphic correlation establishes a similar age of strata based on lithology, which is the composition and physical properties of that strata. Lithos is Greek for stone and -logy comes from the Greek word for doctrine or science. Lithostratigraphic correlation can be used to correlate whole formations long distances or can be used to correlate smaller strata within formations to trace their extent and regional depositional environments. For example, the Navajo Sandstone, which makes up the prominent walls of Zion National Park, is the same Navajo Sandstone in Canyonlands because the lithology of the two are identical even though they are hundreds of miles apart. Extensions of the same Navajo Sandstone formation are found miles away in other parts of southern Utah, including Capitol Reef and Arches National Parks. Chronostratigraphic Correlation Chronostratigraphic correlation matches rocks of the same age, even though they are made of different lithologies. Different lithologies of sedimentary rocks can form at the same time at different geographic locations because depositional environments vary geographically. For example, at any one time in a marine setting, there could be this sequence of depositional environments from the beach to deep marine: beach, nearshore area, shallow marine lagoon, reef, slope, and deep marine. Each depositional environment will have a unique sedimentary rock formation. Biostratigraphic correlation Biostratigraphic correlation uses index fossils to determine strata ages. Index fossils represent assemblages or groups of organisms that were uniquely present during specific intervals of geologic time. Assemblages refer to a group of fossils. Fossils allow geologists to assign a formation to an absolute date range, such as the Jurassic Period (199 to 145 million years ago), rather than a relative time scale. In fact, most 18 of the geologic time ranges are mapped to fossil assemblages. The most useful index fossils come from lifeforms that were geographically widespread and had a species lifespan that was limited to a narrow time interval. In other words, index fossils can be found in many places around the world, but only during a narrow time frame. Some of the best fossils for biostratigraphic correlation are microfossils, most of which came from single-celled organisms. As with microscopic organisms today, they were widely distributed across many environments throughout the world. Some of these microscopic organisms had hard parts, such as exoskeletons or outer shells, making them better candidates for preservation. Foraminifera, single-celled organisms with calcareous shells, are an example of an especially useful index fossil for the Cretaceous Period and Cenozoic Era. Conodonts are another example of microfossils useful for biostratigraphic correlation of the Cambrian through Triassic Periods. Conodonts are tooth-like phosphatic structures of an eel-like multi-celled organism that had no other preservable hard parts. The conodont-bearing creatures lived in shallow marine environments all over the world. Upon death, the phosphatic hard parts were scattered into the rest of the marine sediments. These distinctive tooth-like structures are easily collected and separated from limestone in the laboratory. 19 Figure 9: Microscopic image of conodont fossil. Geologic time scale. The geologic time scale and basic outline of Earth’s history were worked out long before we had any scientific means of assigning numerical age units, like years, to events of Earth history. Working out Earth’s history depended on realizing some key principles of relative time. Nicolas Steno (1638-1686) introduced basic principles of stratigraphy, the study of layered rocks, in 1669. William Smith (1769-1839), working with the strata of English coal mines, noticed that strata and their sequence were consistent throughout the region. Eventually he produced the first national geologic map of Britain, becoming known as “the Father of English Geology.” Nineteenth-century scientists developed a relative time scale using Steno’s principles, with names derived from the characteristics of the rocks in those areas. The figure of this geologic time scale shows the names of the units and subunits. Using this time scale, geologists can place all events of Earth history in order without ever knowing their numerical ages. 20 Fossils and the geological time scale The need for detailed geological mapping of the rocks and minerals in the Earth and the matching-up or correlation of rock layers, are vital to the search and discovery of economically important minerals. To do this, rock units have to be described and the same sort of layers recognized worldwide. Geologists created the geologic time scale to organize Earth’s history into eons, eras, periods, and epochs. While a human life spans decades, geologic time spans all of Earth’s history 4,600 million years. Fossils are often used to help match up rock layers all over the world. Sedimentary rock units are frequently recognized by their unique or distinctive fossils. These important layers of sedimentary rocks are used to make up the Geological Time Scale. The Geological Time Scale divides the millions of years of Earth history into units or periods. Most of the boundaries on the geological time scale correspond to the origination or extinction of particular kinds of fossils. Knowing when major groups of fossils first appeared or went extinct is therefore incredibly useful for determining the ages of rocks in the field. For example, if you find a rock with a trilobite fossil upon it, you will immediately know that the rock is Paleozoic in age (541 Ma to 252 Ma) and not older or younger; knowing the species of trilobite allows even greater precision. The principle of faunal succession was developed by an English surveyor named William "Strata" Smith (1769-1839). As he studied layers of rocks to determine where to build canals, he noticed that he found the same ordering of fossil species from place to place; Fossil A was always found below Fossil B, which in turn was always found below Fossil C, and so on. By documenting these sequences of fossils, Smith was able to temporally correlate rock layers (or, strata) from place to place (in other words, to establish that rock layers in two different places are equivalent in age based upon the fact that they include the same types of fossils). Temporal correlation allowed Smith to construct the first geological map of an entire country. 21 Temporal correlation has made many people extremely rich by allowing them to predict the locations of valuable geological resources such as fossil fuels. More generally, it has allowed us to reconstruct the geological history of Earth by comparing rocks and fossils from place to place. This is critically important because no single place on Earth preserves a complete geological history, or even a small fraction of it. Because of its usefulness for communicating about events in Earth's history, it is important that all students of geology, geophysics, civil engineering, paleontology, and evolutionary biology commit the geological time scale to memory. This is most easily done by first breaking the time scale into its component parts: eons, eras, periods, and epochs. Eons The eon is the broadest category of geological time. Earth's history is characterized by four eons; in order from oldest to youngest, these are the Hadeon, Archean, Proterozoic, and Phanerozoic. Collectively, the Hadean, Archean, and Proterozoic are sometimes informally referred to as the "Precambrian." (The Cambrian period defines the beginning of the Phanerozoic eon; so, all rocks older than the Cambrian are Precambrian in age. Eras Eons of geological time are subdivided into eras, which are the second-longest units of geological time. The Phanerozoic eon is divided into three eras: the Paleozoic, Mesozoic, and Cenozoic. Most of our knowledge of the fossil record comes from the three eras of the Phanerozoic eon. The Paleozoic ("old life") era is characterized by trilobites, the first four-limbed vertebrates, and the origin of land plants. During the Paleozoic Era (541 to 252 million years ago) Fish diversified and marine organisms were very abundant during the Paleozoic. Common Paleozoic fossils include 22 trilobites and cephalopods such as squid, as well as insects and ferns. The greatest mass extinction in Earth's history ended this era. The Mesozoic Era (252 to 66 million years ago) was the "Age of Reptiles."Dinosaurs, crocodiles, and pterosaurs ruled the land and air, also it is noteworthy for the first appearances of mammals and flowering plants. As climate changed, sea levels rose world-wide and seas expanded across the center of North America. Large marine reptiles such as plesiosaurs, along with the coiled-shell ammonites, flourished in these seas. Common Mesozoic fossils include dinosaur bones and teeth, and diverse plant fossils. Finally, the Cenozoic ("new life") era is sometimes called the "age of mammals" 66 million years ago and is the era during which we live today. Birds and mammals rose in prominence after the extinction of giant reptiles. Common Cenozoic fossils include cat-like carnivores and early horses, as well as ice age fossils like wooly mammoths. Caves can preserve the remains of ice-age animals that died in them or were transported there after death. Periods Just as eons are subdivided into eras, eras are subdivided into units of time called periods. The most famous of all geological periods is the Jurassic period of the Mesozoic era (the movie Jurassic Park, of course, has something to do with that). The Paleozoic era is divided into six periods. From oldest to youngest, these are the Cambrian, Ordovician, Silurian, Devonian, Carboniferous, and Permian. Note that in the United States, the Carboniferous is divided into two separate periods: the Mississippian and the Pennsylvanian. The Mesozoic era is divided into the Triassic, Jurassic, and Cretaceous periods. Finally, the Cenozoic era is divided into three periods: the Paleogene, Neogene, and Quaternary. 23 Epochs and Ages Periods of geological time are subdivided into epochs. In turn, epochs are divided into even narrower units of time called ages. For the sake of simplicity, only the epochs of the Paleogene, Neogene, and Quaternary periods are shown on the time scale at the top of this page. It is important to note, however, that all of the periods of the Phanerozoic era are subdivided into the epochs and ages. The Paleogene period is divided into--from oldest to youngest--the Paleocene, Eocene, and Oligocene epochs. The Neogene is divided into the Miocene and Pliocene epochs. Finally, the Quaternary is divided into the Pleistocene and Holocene epochs. 24 Figure 10: Geologic time scale and the major events that occurred in each era 25