M6 Metals PDF
Document Details
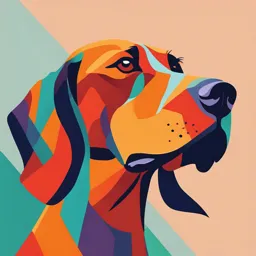
Uploaded by SpeedyNumber
Tags
Related
- Chemistry Notes for NEET - Chapter 16 - General Principles of Extraction of Metals PDF
- ASM Metals Handbook Volume 1 - Irons, Steels, & High Performance Alloys PDF
- ASM Metals Handbook Volume 01 - Properties and Selection of Irons, Steels, and High Performance Alloys PDF
- Chemistry For Engineers: Lesson 4 - Engineering Materials (Metals) PDF
- Engineering Materials Chemistry Reviewer PDF
- NAT SCI 1-CHEMISTRY FOR ENGINEERS Lesson 2: Metals and Alloys PDF
Summary
This document is about the chemistry of metals and alloys. It covers topics such as the structure of metals, models of metallic bonding, including electron sea and band theory, and different types of alloys like substitutional, interstitial, heterogeneous, and intermetallic compounds.
Full Transcript
Chemistry of Engineering Materials M6. METALS 6.1 Structure of Metals 6.2 Models of Metallic Bonding 6.2.1 Electron Sea Model Bond Theory 6.2.2 Band Theory Alloys References: Brown, et al. 2022. Chemistry: The Central Science, 15th Global E...
Chemistry of Engineering Materials M6. METALS 6.1 Structure of Metals 6.2 Models of Metallic Bonding 6.2.1 Electron Sea Model Bond Theory 6.2.2 Band Theory Alloys References: Brown, et al. 2022. Chemistry: The Central Science, 15th Global Edition in SI Units. Pearson Brown, et al. 2018. Chemistry for Engineering students. Cengage Learning Nitinol is a 1:1 nickel–titanium alloy that has „shape memory‟. This remarkable property was discovered by accident when the alloy was presented to a management meeting of the US Naval Ordnance Laboratory (from where it derived its name: Ni-Ti-N-O-L) in 1961. The folded wire was passed around the room and one of the participants used a cigarette lighter to heat the sample. To the astonishment of those present, the wire uncoiled and took on its original shape. Alloys References: Brown, et al. 2022. Chemistry: The Central Science, 15th Global Edition in SI Units. Pearson Brown, et al. 2018. Chemistry for Engineering students. Cengage Learning The wire has two crystal structures that are easily interconverted but that are stable at different temperatures. Heating the wire while holding the desired shape fixes the shape and crystal structure. On cooling, the wire may be deformed into another shape and transform to a more complicated crystal structure. Subsequent warming reforms the original crystal lattice and shape. There are several applications of nitinol in dentistry and medicine. For example, stents used to treat heart conditions can be „set‟ in their open, functioning shape before cooling and compacting for easy insertion into a blocked coronary artery. At body temperature, the nitinol reforms its open shape, allowing blood to flow more freely. Alloys References: Brown, et al. 2022. Chemistry: The Central Science, 15th Global Edition in SI Units. Pearson Brown, et al. 2018. Chemistry for Engineering students. Cengage Learning An alloy is a material that contains more than one element and has the characteristic properties of a metal. The alloying of metals is of great importance because it is one of the primary ways of modifying the properties of pure metallic elements. Nearly all the common uses of iron, for example, involve alloy compositions. Bronze is formed by alloying copper and tin, while brass is an alloy of copper and zinc. Pure gold is too soft to be used in jewelry, but alloys of gold are much harder. Some Common Alloys References: Brown, et al. 2022. Chemistry: The Central Science, 15th Global Edition in SI Units. Pearson Brown, et al. 2018. Chemistry for Engineering students. Cengage Learning Four Categories of Alloys References: Brown, et al. 2022. Chemistry: The Central Science, 15th Global Edition in SI Units. Pearson Brown, et al. 2018. Chemistry for Engineering students. Cengage Learning Alloys can be divided into four categories: substitutional alloys, interstitial alloys, heterogeneous alloys, and intermetallic compounds. Substitutional and interstitial alloys are both homogeneous mixtures in which components are dispersed randomly and uniformly. Solids that form homogeneous mixtures are called solid solutions. When atoms of the solute in a solid solution occupy positions normally occupied by a solvent atom, we have a substitutional alloy. When the solute atoms occupy interstitial positions in the “holes” between solvent atoms, we have an interstitial alloy. Four Categories of Alloys References: Brown, et al. 2022. Chemistry: The Central Science, 15th Global Edition in SI Units. Pearson Brown, et al. 2018. Chemistry for Engineering students. Cengage Learning When atoms of the solute in a solid solution occupy positions normally occupied by a solvent atom, we have a substitutional alloy. Substitutional alloys are formed when the two metallic components have similar atomic radii and chemical-bonding characteristics. Substitutional Alloys References: Brown, et al. 2022. Chemistry: The Central Science, 15th Global Edition in SI Units. Pearson Brown, et al. 2018. Chemistry for Engineering students. Cengage Learning https://wps.prenhall.com/wps/media/objects/3084/3158882/blb2306.html For example, silver and gold form such an alloy over the entire range of possible compositions. When two metals differ in radii by more than about 15%, solubility is generally more limited. Alloys References: Brown, et al. 2022. Chemistry: The Central Science, 15th Global Edition in SI Units. Pearson Brown, et al. 2018. Chemistry for Engineering students. Cengage Learning When the solute atoms occupy interstitial positions in the “holes” between solvent atoms, we have an interstitial alloy. Substitutional and interstitial alloys are both homogeneous mixtures in which components are dispersed randomly and uniformly. Solids that form homogeneous mixtures are called solid solutions. Interstitial Alloys References: Brown, et al. 2022. Chemistry: The Central Science, 15th Global Edition in SI Units. Pearson Brown, et al. 2018. Chemistry for Engineering students. Cengage Learning https://wps.prenhall.com/wps/media/objects/3084/3158882/blb2306.html For an interstitial alloy to form, the solute atoms must have a much smaller bonding atomic radius than the solvent atoms. Typically, the interstitial element is a nonmetal that makes covalent bonds to the neighboring metal atoms. The presence of the extra bonds provided by the interstitial component causes the metal lattice to become harder, stronger, and less ductile. Types of steels References: https://www.meadmetals.com/blog/steel-grades CARBON STEELS Carbon steels only contain trace amounts of elements besides carbon and iron. This group is the most popular of the four grades of steel and it accounts for 90% of steel production. Carbon Steel has three main subgroups depending on how much carbon is in the metal: Low Carbon Steels/Mild Steels (up to 0.3% carbon), Medium Carbon Steels (0.3–0.6% carbon), and High Carbon Steels (more than 0.6% carbon). Companies often manufacture these steels in massive quantities since they‟re cheap to manufacture and they‟re strong enough to use in large-scale. Interstitial Alloys References: Brown, et al. 2022. Chemistry: The Central Science, 15th Global Edition in SI Units. Pearson Brown, et al. 2018. Chemistry for Engineering students. Cengage Learning https://www.youtube.com/watch?v=ey9rW0VbgzE For example, steel, which is much harder and stronger than pure iron, is an alloy of iron that contains up to 3% carbon. Mild steels contain less than 0.2% carbon; they are malleable and ductile and are used to make cables, nails, and chains. Types of steels References: https://www.meadmetals.com/blog/steel-grades https://www.industrialmetalsupply.com/blog/types-of-steel Mild Carbon Steel Properties Applications for Mild Carbon Steel Highly Machinable Automotive components High ductility Structural steel High weldability Wiring Cost-effective Consumer appliances Machinery Forgings Tubing Interstitial Alloys References: Brown, et al. 2022. Chemistry: The Central Science, 15th Global Edition in SI Units. Pearson Brown, et al. 2018. Chemistry for Engineering students. Cengage Learning https://www.bindingwire.org/binding/spring-wire.html Medium steels contain 0.2–0.6% carbon; they are tougher than mild steels and are used to make girders and rails. High-carbon steel, used in cutlery, tools, and springs, contains 0.6–1.5% carbon. In all three types, other elements may be added to form alloy steels. Vanadium and chromium may be added to impart strength, for instance, and to increase resistance to fatigue and corrosion. Types of steels References: https://www.meadmetals.com/blog/steel-grades https://www.industrialmetalsupply.com/blog/types-of-steel Medium Carbon Steel Properties Applications for Medium Carbon Steel Medium Carbon Steel Properties Automotive components such as Medium strength axles or gears Medium ductility Machine parts Low hardenability Heat-treatable Types of steels References: https://www.meadmetals.com/blog/steel-grades https://www.industrialmetalsupply.com/blog/types-of-steel High Carbon Steel Properties Applications for High Carbon Steel High hardness Tooling High strength Die casting Low machinability Steel bars Low ductility Railways For rust protection and corrosion Galvanizing is a process of coating iron resistance, carbon steels are typically or steel with zinc in order to provide treated with hot-dipped galvanization. greater protection against corrosion for the iron or steel base. Interstitial Alloys References: Brown, et al. 2022. Chemistry: The Central Science, 15th Global Edition in SI Units. Pearson Brown, et al. 2018. Chemistry for Engineering students. Cengage Learning https://www.bindingwire.org/binding/spring-wire.html Ultra-High Carbon Steel With a carbon content ranging from 1.51-2%, this metal can be heat treated to exceptional hardness. Specialist knives, axles, and punches are typically made from ultra-high carbon steel. There are some types of steel with more than 2.5% carbon. But these are so challenging to work that they are typically made from powdered metals. Types of steels References: https://www.meadmetals.com/blog/steel-grades https://metalpress.onlinemetals.com/understanding-steel/#types ALLOY STEELS Strictly speaking, every steel is an alloy, but not all steels are called “alloy steels.” However, the term refers to steels that have been alloyed with elements in addition to carbon. The most common elements include manganese, chromium, nickel, silicon, boron, molybdenum and vanadium. Less common alloyants include aluminum, cobalt, copper, cerium, niobium, titanium, tungsten, tin, zinc, lead, and zirconium. Adding other elements to iron and carbon can improve a range of properties. Typically the desired improvements are, strength, hardness, toughness, wear resistance, corrosion resistance, and hardenability. Alloy steels are broken down into two groups: low-alloy and high-alloy. Depending on the composition, some specialty Interstitial Alloys References: Brown, et al. 2022. Chemistry: The Central Science, 15th Global Edition in SI Units. Pearson Brown, et al. 2018. Chemistry for Engineering students. Cengage Learning https://www.unifiedalloys.com/blog/medical-stainless-steel/ One of the most important iron alloys is stainless steel, which contains about 0.4% carbon, 18% chromium, and 1% nickel. The chromium is obtained by carbon reduction of chromite (FeCr2O4) in an electric furnace. The product of the reduction is ferrochrome (FeCr2), which is added in the appropriate amount to molten iron to achieve the desired steel composition. The ratio of elements present in the steel may vary over a wide range, imparting a variety of specific physical and chemical properties to the materials. Types of steels References: https://www.meadmetals.com/blog/steel-grades Brown, et al. 2022. Chemistry: The Central Science, 15th Global Edition in SI Units. Pearson Brown, et al. 2018. Chemistry for Engineering students. Cengage Learning STAINLESS STEELS Stainless steels contain 10-20% chromium as their alloying element, as well as other elements such as nickel, silicon, manganese, and carbon. These steels have remarkably high corrosion resistance and are safe to use in outside construction because they have an increased ability to withstand rough weather. They are also widely utilized in electrical equipment. 304 Stainless Steel, for example, is highly sought after for its ability to withstand the elements while keeping electrical material out of harm's way. Types of steels References: https://www.industrialmetalsupply.com/blog/types-of-steel While different grades of stainless steel Properties of stainless steel include at like 304 Stainless Steel have a place in least 10.5% chromium, which helps construction, a majority of industries use prevent corrosion and staining. While stainless steel for their sanitary carbon steel is generally prone to rust, properties. These steels are stainless steel has a chromium film to commonplace in medical equipment, block oxidization. piping, cutting tools, and food processing Stainless Steel Properties equipment. One of the most important iron alloys is the stainless steel which High tensile strength contains about 0.4% carbon, 18% Easily formed chromium, and 1% nickel. Corrosion resistant Eco-friendly Types of steels References: https://www.meadmetals.com/blog/steel-grades https://www.industrialmetalsupply.com/blog/types-of-steel Stainless Steel Types Stainless Steel Applications Austenitic Surgical equipment Martensitic Structural parts Ferritic Food industry applications Duplex Aerospace components Precipitation hardened Automotive valves and fittings Types of steels References: https://www.meadmetals.com/blog/steel-grades https://www.industrialmetalsupply.com/blog/types-of-steel TOOL STEELS Tool steels, as you can probably guess by the name, excel in cutting and drilling equipment. The secret is the tungsten, molybdenum, cobalt, and vanadium that increases their heat resistance and overall durability. With chemical properties like hardness and wear resistance, tooling steel can keep a sharp edge even in the hottest environments. It is ideal for crafting tools or applications that Heterogeneous Alloys References: Brown, et al. 2022. Chemistry: The Central Science, 15th Global Edition in SI Units. Pearson Brown, et al. 2018. Chemistry for Engineering students. Cengage Learning In a heterogeneous alloy the components are not dispersed uniformly. For example, the heterogeneous alloy pearlite contains two phases. One phase is essentially pure body-centered cubic iron, and the other is the compound Fe3C, known as cementite. In general, the properties of heterogeneous alloys depend on both the composition and the manner in which the solid is formed from the molten mixture. The properties of a heterogeneous alloy formed by rapid cooling of a molten mixture, for example, are distinctly different from the properties of an alloy formed by slow cooling of the same mixture. Intermetallic compounds References: Brown, et al. 2022. Chemistry: The Central Science, 15th Global Edition in SI Units. Pearson Brown, et al. 2018. Chemistry for Engineering students. Cengage Learning Intermetallic compounds are compounds rather than mixtures. Because they are compounds, they have definite properties and their composition cannot be varied. Unlike the atoms in substitutional and interstitial alloys, the different types of atoms in an intermetallic compound are ordered rather than randomly distributed. The ordering of atoms in an intermetallic compound generally leads to better structural stability and higher melting points than what is observed in the constituent metals. These features can be attractive for high-temperature applications. On the negative side, intermetallic compounds are often more brittle than substitutional alloys. Intermetallic compounds References: Brown, et al. 2022. Chemistry: The Central Science, 15th Global Edition in SI Units. Pearson Brown, et al. 2018. Chemistry for Engineering students. Cengage Learning Intermetallic compounds References: Brown, et al. 2022. Chemistry: The Central Science, 15th Global Edition in SI Units. Pearson Brown, et al. 2018. Chemistry for Engineering students. Cengage Learning https://www.rtings.com/headphones/learn/dynamic-vs-planar-magnetic The intermetallic compound Ni3Al is a major component of jet aircraft engines because of its strength at high temperature and its low density. Razor blades are often coated with Cr3Pt, which adds hardness, allowing the blade to stay sharp longer. The hexagonal intermetallic compound SmCo5, is used to make the permanent magnets found in lightweight headsets and high-fidelity speakers. A related compound with the same structure, LaNi5, is used as the anode in nickel- metal hydride batteries. Intermetallic compounds References: Brown, et al. 2022. Chemistry: The Central Science, 15th Global Edition in SI Units. Pearson Brown, et al. 2018. Chemistry for Engineering students. Cengage Learning https://spinoff.nasa.gov/Spinoff2012/hm_6.html The compound Nb3Sn, is a superconductor, a substance that, when cooled below a critical temperature, conducts electricity with no resistance. In the case of Nb3Sn superconductivity is observed only when the temperature falls below 18 K. Superconductors are used in the magnets in MRI scanners widely employed for medical imaging. The need to keep the magnets cooled to such a low temperature is part of the reason why MRI devices are expensive to operate. Alloys of Gold References: Brown, et al. 2022. Chemistry: The Central Science, 15th Global Edition in SI Units. Pearson Brown, et al. 2018. Chemistry for Engineering students. Cengage Learning In the jewelry trade pure gold is termed 24 karat. The karat number decreases as the mass percent of gold decreases. The most common alloys used in jewelry are 14 karat, which (14/24) 100 = 58 % gold, and 18 karat, which (18/24) 100 = 75 % gold, Alloys of Gold References: Brown, et al. 2022. Chemistry: The Central Science, 15th Global Edition in SI Units. Pearson Brown, et al. 2018. Chemistry for Engineering students. Cengage Learning Alloying changes a metal‟s properties. An alloy that is 75% Au, 12.5% Ag, and 12.5% Cu (marked with the blue dot has a tensile strength that is more than 10 times higher than that of pure gold and is more than 7 times harder than pure gold. Alloys of Gold References: Brown, et al. 2022. Chemistry: The Central Science, 15th Global Edition in SI Units. Pearson Brown, et al. 2018. Chemistry for Engineering students. Cengage Learning The color of gold varies depending on the metals it is alloyed with. Gold is typically alloyed with silver and/or copper. All three elements crystallize with a face-centered cubic structure. The fact that all three elements have similar radii (Au and Ag are nearly the same size, Cu is roughly 11% smaller) and crystallize with the same type of structure make it possible to form substitutional alloys with nearly any composition. Alloys of Gold References: Brown, et al. 2022. Chemistry: The Central Science, 15th Global Edition in SI Units. Pearson Brown, et al. 2018. Chemistry for Engineering students. Cengage Learning Gold alloyed with equal parts silver and copper takes on the golden yellow color we associate with gold jewelry. Red or rose gold is a copper- rich alloy. Silver-rich alloys take on a greenish hue, eventually giving way to silvery-white colors as silver becomes the majority constituent. Alloys of Gold References: Brown, et al. 2022. Chemistry: The Central Science, 15th Global Edition in SI Units. Pearson Brown, et al. 2018. Chemistry for Engineering students. Cengage Learning Gold can also be alloyed with many other metals. White gold is generally obtained by alloying either nickel or palladium with gold. Although Ni-Au alloys are cheaper, they are generally considered inferior because they are harder to work and because many people suffer an allergic reaction to nickel. All of these alloys are substitutional alloys, but gold also forms some interesting intermetallic compounds. Alloys of Gold References: Brown, et al. 2022. Chemistry: The Central Science, 15th Global Edition in SI Units. Pearson Brown, et al. 2018. Chemistry for Engineering students. Cengage Learning Reacting gold and aluminum in a 1:2 mole ratio produces the intermetallic compound AuAl2, which is known as purple gold for its unusual color. If the aluminum is replaced with indium to form AuIn2 the color changes to blue. Because they are intermetallic compounds, purple and blue gold are brittle, which makes it very difficult to form them into rings and other jewelry pieces. They can be used as gemstones or inlays, however, as in the ring shown. Metals References: Brown, et al. 2022. Chemistry: The Central Science, 15th Global Edition in SI Units. Pearson Brown, et al. 2018. Chemistry for Engineering students. Cengage Learning Metallic solids are held together by a delocalized “sea” of collectively shared valence electrons. This form of bonding allows metals to conduct electricity and also responsible for the fact that most metals are relatively strong without being brittle. Metallic solids, also simply called metals, consist entirely of metal atoms. Metallic Solids References: Brown, et al. 2022. Chemistry: The Central Science, 15th Global Edition in SI Units. Pearson Brown, et al. 2018. Chemistry for Engineering students. Cengage Learning https://www.tutorialspoint.com/chemistry_part1/chemistry_metals_and_non_metals_2.htm The bonding in metals is too strong to be due to dispersion forces, and yet there are not enough valence electrons to form covalent bonds between atoms. The bonding, called metallic bonding, results from the fact that the valence electrons are delocalized throughout the entire solid. That is, the valence electrons are not associated with specific atoms or bonds but are spread throughout the solid. In fact, we can visualize a metal as an array of positive ions immersed in a “sea” of delocalized valence electrons. Metallic Properties References: Brown, et al. 2022. Chemistry: The Central Science, 15th Global Edition in SI Units. Pearson Brown, et al. 2018. Chemistry for Engineering students. Cengage Learning https://www.tekportal.net/metal/ A clean metal surface has a characteristic luster. In addition, metals that we can handle with bare hands have a characteristic cold feeling related to their high thermal conductivity. Metals also have high electrical conductivity, which means that electrically charged particles flow easily through them. The thermal conductivity of a metal usually parallels its electrical conductivity. Silver and copper, for example, which possess the highest electrical conductivities among the elements, also possess the highest thermal conductivities. Metallic Properties References: Serway and Jewett. 2019. Physics for Scientists and Engineers. Cengage Young and Freedman. 2020. University Physics with Modern Physics. Person Metallic Properties References: Brown, et al. 2022. Chemistry: The Central Science, 15th Global Edition in SI Units. Pearson Brown, et al. 2018. Chemistry for Engineering students. Cengage Learning Most metals are malleable, which means that they can be hammered into thin sheets, and ductile, which means that they can be drawn into wires. These properties indicate that the atoms are capable of slipping past one another. Models of Metallic Bonding References: Brown, et al. 2022. Chemistry: The Central Science, 15th Global Edition in SI Units. Pearson Brown, et al. 2018. Chemistry for Engineering students. Cengage Learning The first and simplest explanation of metallic bonding is often referred to as the sea of electrons model. The essential feature of this model is that the valence electrons of metal atoms are delocalized and move freely throughout the solid rather than being tied to any specific atom. The blue area represents the delocalized (and negatively charged) valence electrons spread throughout a piece of metal. The circles depict the positively charged cores of the metal atoms. The “electron sea” model of metallic bonding is somewhat crude but does account for the malleability and ductility of metals, as well as their electrical conductivity. Metallic Properties References: Brown, et al. 2022. Chemistry: The Central Science, 15th Global Edition in SI Units. Pearson Brown, et al. 2018. Chemistry for Engineering students. Cengage Learning The “electron sea” model of metallic bonding is somewhat crude but does account for the malleability and ductility of metals, as well as their electrical conductivity. The blue area represents the delocalized (and negatively charged) valence electrons spread throughout a piece of metal. The circles depict the positively charged cores of the metal atoms. Electron-Sea Model References: Brown, et al. 2022. Chemistry: The Central Science, 15th Global Edition in SI Units. Pearson Brown, et al. 2018. Chemistry for Engineering students. Cengage Learning To understand malleability, consider what would happen at the atomic level if a metal were struck with a hammer. At least some of the atoms would respond to this force by moving relative to others. If the electrons are moving freely, however, as postulated in the sea of electrons model, they can adjust to the new position of the atoms and bonding is relatively unaffected. Electron-Sea Model References: Brown, et al. 2022. Chemistry: The Central Science, 15th Global Edition in SI Units. Pearson Brown, et al. 2018. Chemistry for Engineering students. Cengage Learning In the left-hand picture, the atoms are arranged in a regular array. A force is applied as indicated by the large arrows—perhaps by striking the metal with a hammer. The cores of the metal atoms move in response to this force, and the sample is deformed as shown in the right-hand picture. But the free flow of electrons maintains the bonding throughout this process. Electron-Sea Model References: Brown, et al. 2022. Chemistry: The Central Science, 15th Global Edition in SI Units. Pearson Brown, et al. 2018. Chemistry for Engineering students. Cengage Learning https://www.bartleby.com/subject/science/physics/concepts/mechanical-properties-of-solids Similarly, pulling a metal into a wire changes the positions of the atoms, but delocalized electrons can adjust and maintain metallic bonding. Finally, these delocalized electrons can move in response to any applied electrical field, so the conductivity of metals can also be understood from the model. Molecular-Orbital Model References: Brown, et al. 2022. Chemistry: The Central Science, 15th Global Edition in SI Units. Pearson Brown, et al. 2018. Chemistry for Engineering students. Cengage Learning Although the electron-sea model works surprisingly well given its simplicity, it does not adequately explain many properties of metals. According to the model, for example, the strength of bonding between metal atoms should steadily increase as the number of valence electrons increases, resulting in a corresponding increase in the melting points. However, elements near the middle of the transition metal series, rather than those at the end, have the highest melting points in their respective. This trend implies that the strength of metallic bonding first increases with increasing number of electrons and then decreases. Similar trends are seen in other physical properties of the metals, such as the boiling point, heat of Molecular-Orbital Model References: Brown, et al. 2022. Chemistry: The Central Science, 15th Global Edition in SI Units. Pearson Brown, et al. 2018. Chemistry for Engineering students. Cengage Learning To obtain a more accurate picture of the bonding in metals, we must turn to molecular- orbital theory (band theory). Here are some of the rules of molecular-orbital theory: 1. Atomic orbitals combine to make molecular orbitals that can extend over the entire molecule. 2. A molecular orbital can contain zero, one, or two electrons. 3. The number of molecular orbitals in a molecule equals the number of atomic orbitals that combine to form molecular orbitals. Molecular-Orbital Model References: Brown, et al. 2022. Chemistry: The Central Science, 15th Global Edition in SI Units. Pearson Brown, et al. 2018. Chemistry for Engineering students. Cengage Learning The sea of electrons model provides qualitative understanding, but quantitative models for metallic bonding also exist. The most important such model is band theory, and we can use our example of putting metal atoms into a carbon nanotube to explain the origins of this theory, which was based on molecular-orbital theory. If you could put lithium atoms inside a suitably sized nanotube, the metal atoms could be made to line up into a “wire” just one atom wide. Molecular-Orbital Model References: Brown, et al. 2022. Chemistry: The Central Science, 15th Global Edition in SI Units. Pearson Brown, et al. 2018. Chemistry for Engineering students. Cengage Learning Because the valence electron configuration of lithium is 2s1, any bonding interactions between the metal atoms will have to be based on the 2s orbitals. Those orbitals, of course, are waves, and so we can use the concept of wave interference to think about how they will interact with one another. We‟ll start with the simplest case: two lithium atoms placed close enough together for their 2s orbitals to overlap one another and interact. Now recall that waves can interfere either constructively or destructively. Molecular-Orbital Model References: Brown, et al. 2022. Chemistry: The Central Science, 15th Global Edition in SI Units. Pearson Brown, et al. 2018. Chemistry for Engineering students. Cengage Learning If the pair of 2s orbitals interacts in phase with one another, the interference will be constructive. The resulting wave, which will have increased amplitude (or electron density) between the nuclei, is referred to as a bonding molecular orbital. On the other hand, if the pair of 2s orbitals interacts out of phase with one another, the resulting wave will have a node between the nuclei. This is known as an antibonding molecular orbital. Molecular-Orbital Model References: Brown, et al. 2022. Chemistry: The Central Science, 15th Global Edition in SI Units. Pearson Brown, et al. 2018. Chemistry for Engineering students. Cengage Learning The molecular-orbital diagram for Li2 has one filled bonding molecular orbital and one empty antibonding molecular orbital with a nodal plane between the atoms. For Li4, there are four molecular orbitals, ranging from the lowest- energy orbital, where the orbital interactions are completely bonding (0 nodal planes), to the highest-energy orbital, where all interactions are antibonding (3 nodal planes). Molecular-Orbital Model References: Brown, et al. 2022. Chemistry: The Central Science, 15th Global Edition in SI Units. Pearson Brown, et al. 2018. Chemistry for Engineering students. Cengage Learning As the length of the chain increases, the number of molecular orbitals increases. Regardless of chain length, the lowest- energy orbitals are always the most bonding and the highest-energy orbitals always the most antibonding. Furthermore, because each lithium atom has only one valence shell atomic orbital, the number of molecular orbitals is equal to the number of lithium atoms in the chain. Molecular-Orbital Model References: Brown, et al. 2022. Chemistry: The Central Science, 15th Global Edition in SI Units. Pearson Brown, et al. 2018. Chemistry for Engineering students. Cengage Learning Because each lithium atom has one valence electron, half of the molecular orbitals are fully occupied and the other half are empty, regardless of chain length. If the chain becomes very long, there are so many molecular orbitals that the energy separation between them becomes vanishingly small. Molecular-Orbital Model References: Brown, et al. 2022. Chemistry: The Central Science, 15th Global Edition in SI Units. Pearson Brown, et al. 2018. Chemistry for Engineering students. Cengage Learning As the chain length goes to infinity, the allowed energy states become a continuous band. For a crystal large enough to see with the eye (or even an optical microscope), the number of atoms is extremely large. Consequently, the electronic structure of the crystal is like that of the infinite chain, consisting of bands, as shown on the right-hand side of the figure.. Molecular-Orbital Model References: Brown, et al. 2022. Chemistry: The Central Science, 15th Global Edition in SI Units. Pearson Brown, et al. 2018. Chemistry for Engineering students. Cengage Learning The electronic structures of most metals are more complicated than shown in the figure because we have to consider more than one type of atomic orbital on each atom. Because each type of orbital can give rise to its own band, the electronic structure of a solid usually consists of a series of bands. The electronic structure of a bulk solid is referred to as a band structure. Molecular-Orbital Model References: Brown, et al. 2022. Chemistry: The Central Science, 15th Global Edition in SI Units. Pearson Brown, et al. 2018. Chemistry for Engineering students. Cengage Learning The band structure for a typical metal is shown schematically in the figure shown. The electron filling depicted corresponds to nickel metal, but the basic features of other metals are similar. The electron configuration of a nickel atom is [Ar]3d84s2, as shown on the left side of the figure. The energy bands that form from each of these orbitals are shown on the right side. Molecular-Orbital Model References: Brown, et al. 2022. Chemistry: The Central Science, 15th Global Edition in SI Units. Pearson Brown, et al. 2018. Chemistry for Engineering students. Cengage Learning The 4s, 4p, and 3d orbitals are treated independently, each giving rise to a band of molecular orbitals. In practice, these overlapping bands are not completely independent of each other, but for our purposes this simplification is reasonable. The 4s, 4p, and 3d bands differ from one another in the energy range they span (represented by the heights of the rectangles on the right side of the figure shown) and in the number of electrons they can hold (represented by the area of the rectangles). Molecular-Orbital Model References: Brown, et al. 2022. Chemistry: The Central Science, 15th Global Edition in SI Units. Pearson Brown, et al. 2018. Chemistry for Engineering students. Cengage Learning The 4s, 4p, and 3d bands can hold 2, 6, and 10 electrons per atom, respectively, two per orbital, as dictated by the Pauli exclusion principle. The energy range spanned by the 3d band is smaller than the range spanned by the 4s and 4p bands because the 3d orbitals are smaller and, therefore, overlap with orbitals on neighboring atoms less effectively. Molecular-Orbital Model References: Brown, et al. 2022. Chemistry: The Central Science, 15th Global Edition in SI Units. Pearson Brown, et al. 2018. Chemistry for Engineering students. Cengage Learning This reduces the strength of the bonding interactions, which dominate the bottom of the band, as well as the antibonding interactions, which dominate the top of the band. As a result, the 3d band spans a narrower range of energy than the 4s and 4p bands. Many properties of metals can be understood from the figure shown. We can think of the energy band as a partially filled container for electrons. The incomplete filling of the energy band gives rise to characteristic metallic properties. Molecular-Orbital Model References: Brown, et al. 2022. Chemistry: The Central Science, 15th Global Edition in SI Units. Pearson Brown, et al. 2018. Chemistry for Engineering students. Cengage Learning The electrons in orbitals near the top of the occupied levels require very little energy input to be “promoted” to higher- energy orbitals that are unoccupied. Under the influence of any source of excitation, such as an applied electrical potential or an input of thermal energy, electrons move into previously vacant levels and are thus freed to move through the lattice, giving rise to electrical and thermal conductivity. Molecular-Orbital Model References: Brown, et al. 2022. Chemistry: The Central Science, 15th Global Edition in SI Units. Pearson Brown, et al. 2018. Chemistry for Engineering students. Cengage Learning Without the overlap of energy bands, the periodic properties of metals could not be explained. In the absence of the d- and p- bands, we would expect the s-band to be half- filled for the alkali metals (group 1A) and completely filled for the alkaline- earth metals (group 2A). If that were true, metals like magnesium, calcium, and strontium would not be good electrical and thermal conductors, in disagreement with experimental observations. Molecular-Orbital Model References: Brown, et al. 2022. Chemistry: The Central Science, 15th Global Edition in SI Units. Pearson Brown, et al. 2018. Chemistry for Engineering students. Cengage Learning While the conductivity of metals can be qualitatively understood using either the electron-sea model or the molecular-orbital model, many physical properties of transition metals, such as the melting points plotted in the figure shown, can be explained only with the latter model. The molecular-orbital model predicts that bonding first becomes stronger as the number of valence electrons increases and the bonding orbitals are populated. Upon moving past the middle elements of the transition metal series, the bonds grow weaker as we fill the antibonding orbitals. Strong bonds between atoms lead to metals with higher melting and boiling points, higher heats of fusion, higher Band Theory and Conductivity References: Brown, et al. 2022. Chemistry: The Central Science, 15th Global Edition in SI Units. Pearson Brown, et al. 2018. Chemistry for Engineering students. Cengage Learning Aufbau principle dictates that electrons occupy the lowest energy orbitals, likewise electrons in bulk materials fill the bands starting at the lowest energy. The highest energy with electron occupation and the energy gap between this energy level and the next available band provide a means for understanding the electrical conductivity of a material.Electrons carry a current by moving through a material, and this motion can be thought of in terms of electrons moving from one orbital to Band Theory and Conductivity References: Brown, et al. 2022. Chemistry: The Central Science, 15th Global Edition in SI Units. Pearson Brown, et al. 2018. Chemistry for Engineering students. Cengage Learning But electrons within a filled band cannot move readily to conduct electricity. To have mobile electrons, we will need to have an unfilled band or portion of a band that is close in energy to a filled band or portion of a band. In the band diagram for lithium shown on the right- hand side of the figure, the band populated by valence electrons is referred to as the valence band, and the unoccupied band above it is known as the conduction band. Band Theory and Conductivity References: Brown, et al. 2022. Chemistry: The Central Science, 15th Global Edition in SI Units. Pearson Brown, et al. 2018. Chemistry for Engineering students. Cengage Learning The conduction band lies directly above the valence band in energy, and the highest occupied energy level sits at the boundary between these two bands. So an electron sitting at the top of the valence band needs to gain only a very small amount of energy to move into the conduction band. This means that lithium should be a good electrical conductor, which it is. Metals, Insulators, and Semiconductors References: Brown, et al. 2022. Chemistry: The Central Science, 15th Global Edition in SI Units. Pearson Brown, et al. 2018. Chemistry for Engineering students. Cengage Learning For nonmetal, on the other hand, has a large energy gap between the filled valence band and the empty conduction band. So it takes a significant amount of energy to reach that empty band, where electron movement would be allowed. Under ordinary circumstances, electrons will not have enough energy to overcome this large band gap, so nonmetals do not conduct and are referred to as insulators. The diamond form of carbon is an excellent example of a nonconductive material. Metals, Insulators, and Semiconductors References: Brown, et al. 2022. Chemistry: The Central Science, 15th Global Edition in SI Units. Pearson Brown, et al. 2018. Chemistry for Engineering students. Cengage Learning The intermediate case is a semiconductor, shown in the center panel of the figure. The highest filled energy level is at the top of the valence band, but the next band is only modestly higher in energy. In semiconductors, some electrons have enough thermal energy to reach the upper band even at room temperature, and therefore they have at least limited electrical conductivity. Metals, Insulators, and Semiconductors References: Brown, et al. 2022. Chemistry: The Central Science, 15th Global Edition in SI Units. Pearson Brown, et al. 2018. Chemistry for Engineering students. Cengage Learning If the temperature is increased, more electrons should occupy the upper band, and the conductivity of semiconductors increases at higher temperatures. Silicon, from the same group as carbon, is the prototypical example of a pure element that behaves as a semiconductor. Semiconductors References: Brown, et al. 2022. Chemistry: The Central Science, 15th Global Edition in SI Units. Pearson Brown, et al. 2018. Chemistry for Engineering students. Cengage Learning https://brightensolarco.com/silicon-leads-cheaper-thermal-energy-storage-systems-solar-energy/ Silicon and germanium lie immediately below carbon in the periodic table. Like carbon, each of these elements has four valence electrons, just the right number to satisfy the octet rule by forming single covalent bonds with four neighbors. Hence, silicon and germanium, as well as the gray form of tin, crystallize with the same infinite network of covalent bonds as diamond. Semiconductors can be divided into two classes, elemental semiconductors, which contain only one type of atom, and compound semiconductors, which contain two or more elements. Elemental Semiconductors References: Brown, et al. 2022. Chemistry: The Central Science, 15th Global Edition in SI Units. Pearson Brown, et al. 2018. Chemistry for Engineering students. Cengage Learning https://www.aculon.com/hydrophobic-germanium/ The elemental semiconductors all come from group 4A. As we move down the periodic table, bond distances increase, which decreases orbital overlap. This decrease in overlap reduces the difference between the bonding molecular orbitals of the valence band and the antibonding molecular orbitals of the conduction band. As a result, the band gap decreases on going from diamond (5.5 eV) to silicon (1.11 eV) to germanium (0.67 eV) to gray tin (0.08 eV). Compound Semiconductor References: Brown, et al. 2022. Chemistry: The Central Science, 15th Global Edition in SI Units. Pearson Brown, et al. 2018. Chemistry for Engineering students. Cengage Learning Compound semiconductors maintain the same average valence electron count as elemental semiconductors—four per atom. For example, when gallium (group 3A) and arsenic (group 5A) combine to form gallium arsenide, GaAs, each Ga atom contributes three electrons and each As atom contributes five, which averages out to four per atom—the same number as in silicon or germanium. Hence, GaAs is a semiconductor. Other examples are InP, where indium contributes three valence electrons and phosphorus contributes five, and CdTe, where cadmium provides two valence electrons and tellurium contributes six. In both cases, the average is again four valence electrons per atom. GaAs, InP, and CdTe all crystallize with a zinc blende structure. Semiconductor Doping References: Brown, et al. 2022. Chemistry: The Central Science, 15th Global Edition in SI Units. Pearson Brown, et al. 2018. Chemistry for Engineering students. Cengage Learning In a semiconductor, the valence band is filled with electrons and the conduction band is empty. These two bands are separated by an energy band gap, Eg. The band that forms from bonding molecular orbitals is called the valence band, and the band that forms from antibonding orbitals is called the conduction band. References: Brown, et al. 2022. Chemistry: The Central Science, 15th Global Edition in SI Units. Pearson Brown, et al. 2018. Chemistry for Engineering students. Cengage Learning Semiconductor Doping References: Brown, et al. 2022. Chemistry: The Central Science, 15th Global Edition in SI Units. Pearson Brown, et al. 2018. Chemistry for Engineering students. Cengage Learning Electrical engineers manipulate both the orbital overlap and the bond polarity to control the band gaps of compound semiconductors for use in a wide range of electrical and optical devices. The electrical conductivity of a semiconductor is influenced by the presence of small numbers of impurity atoms. The process of adding controlled amounts of impurity atoms to a material is known as doping. Semiconductor Doping References: Brown, et al. 2022. Chemistry: The Central Science, 15th Global Edition in SI Units. Pearson Brown, et al. 2018. Chemistry for Engineering students. Cengage Learning Consider what happens when a few phosphorus atoms (known as dopants) replace silicon atoms in a silicon crystal. In pure Si all of the valence-band molecular orbitals are filled and all of the conduction-band molecular orbitals are empty. Because phosphorus has five valence electrons but silicon has only four, the “extra” electrons that come with the dopant phosphorus atoms are forced to occupy the conduction band. The doped material is called an n-type semiconductor, n signifying that the number of negatively charged electrons in the conduction band has increased. These extra electrons can move very easily in the conduction band. Thus, just a few parts per million (ppm) of phosphorus in silicon can increase silicon‟s intrinsic conductivity by a factor of a million! Semiconductor Doping References: Brown, et al. 2022. Chemistry: The Central Science, 15th Global Edition in SI Units. Pearson Brown, et al. 2018. Chemistry for Engineering students. Cengage Learning Semiconductor Doping References: Brown, et al. 2022. Chemistry: The Central Science, 15th Global Edition in SI Units. Pearson Brown, et al. 2018. Chemistry for Engineering students. Cengage Learning It is also possible to dope semiconductors with atoms that have fewer valence electrons than the host material. Consider what happens when a few aluminum atoms replace silicon atoms in a silicon crystal. Aluminum has only three valence electrons compared to silicon‟s four. Thus, there are electron vacancies, known as holes, in the valence band when silicon is doped with aluminum. Since the negatively charged electron is not there, the hole can be thought of as having a positive charge. Any adjacent electron that jumps into the hole leaves behind a new hole. Thus, the positive hole moves about in the lattice like a particle. A material like this is called a p-type semiconductor, p signifying that the number of positive holes in the material has increased. Semiconductor Doping References: Brown, et al. 2022. Chemistry: The Central Science, 15th Global Edition in SI Units. Pearson Brown, et al. 2018. Chemistry for Engineering students. Cengage Learning Semiconductor Doping References: Brown, et al. 2022. Chemistry: The Central Science, 15th Global Edition in SI Units. Pearson Brown, et al. 2018. Chemistry for Engineering students. Cengage Learning The junction of an n-type semiconductor with a p-type semiconductor forms the basis for diodes, transistors, solar cells, and other devices. Reference: Brown, et al. 2022. Chemistry: The Central Science, 15th Global Edition in SI Units. Pearson Artificial lighting is so widespread we take it for granted. Major savings in energy would be realized if incandescent lights could be replaced by light-emitting diodes (LEDs). Because LEDs are made from semiconductors, this is an appropriate place to take a closer look at the operation of an LED. Reference: Brown, et al. 2022. Chemistry: The Central Science, 15th Global Edition in SI Units. Pearson The heart of an LED is a p–n diode, which is formed by bringing an n-type semiconductor into contact with a p-type semiconductor. In the junction where they meet, there are very few electrons or holes to carry the charge across the interface between them, and the conductivity decreases. Reference: Brown, et al. 2022. Chemistry: The Central Science, 15th Global Edition in SI Units. Pearson When an appropriate voltage is applied, electrons are driven from the conduction band of the n- doped side into the junction, where they meet holes that have been driven from the valence band of the p-doped side. The electrons fall into the empty holes, and their energy is converted into light whose photons have energy equal to the band gap (Figure 12.32). In this way electrical energy is converted into optical LEDs Reference: Brown, et al. 2022. Chemistry: The Central Science, 15th Global Edition in SI Units. Pearson Because the wavelength of light that is emitted depends on the band gap of the semiconductor, the color of light produced by the LED can be controlled by appropriate choice of semiconductor. Most red LEDs are made of a mixture of GaP and GaAs. Because the light emission results from semiconductor structures that can be made extremely small and because they emit little heat, LEDs are replacing standard incandescent and fluorescence light bulbs in many applications.