LT.02 Normal Bone Marrow PDF
Document Details
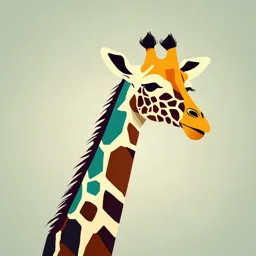
Uploaded by StableTheory
University of Cape Town
Tags
Summary
This document provides background information about the function of bone marrow, focusing on differentiation and maturation processes involved in blood cell production. It uses an analogy of education and career progression to explain these concepts.
Full Transcript
LT.02. Normal Bone Marrow Aim To provide background knowledge of how the bone marrow functions so that further discussion of haematological disease can refer to these underlying principles. Delivery objectives 1. Define and explain the concepts of differentiation and maturation with regard to haemo...
LT.02. Normal Bone Marrow Aim To provide background knowledge of how the bone marrow functions so that further discussion of haematological disease can refer to these underlying principles. Delivery objectives 1. Define and explain the concepts of differentiation and maturation with regard to haemopoiesis. 2. Discuss the components of bone marrow required to produce mature blood cells. 3. Describe the maturation stages of haemopoietic cells. Content If you consider that under normal conditions the adult bone marrow produces approximately 1011 of each of the three major blood cells (red cells, neutrophils and platelets) PER DAY, the scale of blood production is difficult to comprehend. In addition, the blood and bone marrow form a very dynamic organ system which must be able to respond rapidly to a variety of stresses, from infection to trauma and blood loss. This capacity has to be maintained for a whole lifetime – sometimes a hundred years or more – without failing. How is this achieved? Essentially the bone marrow consists of immature blood cells situated in a specific microenvironment and whose proliferation is controlled by means of growth factors. A. Haemopoietic cells The immature cells in the marrow are present in different stages of differentiation and maturation, forming a hierarchy of cells from the most primitive (stem cells) to the most mature (red cells, granulocytes such as neutrophils, and platelets) The terms “differentiation” and “maturation” have specific meanings. To illustrate these, consider the analogy of a person’s education and career. Before you went to school you were full of potential, you could be anything you wanted to be and nothing was limiting your choice - at this stage you were undifferentiated and analogous to a stem cell. At the end of primary school and beginning of high school you made some choices in subjects that started to limit your future direction e.g. would you take French, science or accountancy? By grade 10 your choices were becoming very limited. If you did not take maths for matric your chances of getting into medical school or becoming an engineer would be very small. This narrowing of options with each new choice can be likened to “differentiation” – the process of becoming more different or specialised. You decided to become a doctor and entered medical school, further reducing your options. Every decision you take will tend to steer you in a certain direction – even your choice of internship and community service will lead you towards a particular final outcome. At each stage it becomes less likely that you will go back to become something else, an architect for example. One day your training will be complete and you will be a fully trained medical practitioner. At this point you will make your final commitment to your chosen field and begin gaining experience and practical skills as a registrar. This stage is analogous to the final maturation of the cell e.g. from blast to neutrophil. “Maturity” is the process of becoming better equipped to do what you have chosen to do. The diagram shows the progressively narrowing options of haemopoietic cells from the stem cell, which can give rise to any of the haemopoietic cell types, to cells committed to a particular lineage e.g. megakaryocytic or monocytic. Some of the terms used, like “CFU”, come from experimental work in which primitive marrow cells were grown on agar plates much like bacteria are cultured in the laboratory. “Colony Forming Unit” refers to a cell that divided to form a cluster, or colony, of similar cells. The haemopoietic hierarchy Pluripotent Stem Cell Haemopoietic Lymphoid Stem Cell Stem Cell CFU- GEMM CFU- E CFU-GM CFU-Meg CFU-Eo CFU-Baso Proliferation – transit amplification Maturation Erythroid Neutrophil Monocyte Megakaryocyte Eosinophil Basophil 7 One difference from this analogy is that the cells have the ability to proliferate until they are fully differentiated and have started to mature along their ultimate choice of lineage. Division of a cell early in the differentiation pathway has the effect of increasing potential cell numbers enormously, as can be seen in the diagram. What then is a stem cell? As most cells divide they give rise to daughter cells that are slightly different from themselves, usually being more differentiated. A stem cell however has the property of self-renewal – at least one of the daughter cells is identical to it and replaces the original stem cell exactly. A stem cell must also be able to give rise to a daughter cell which is more differentiated than itself otherwise the tissue would never be able to reach its final form. In the marrow stem cells are extremely rare and cannot be recognised by what they look like. Proof of their existence is based on experimental evidence e.g. by the ability of a few primitive cells to reconstitute the entire bone marrow when the original marrow has been ablated. B. Growth factors These substances, when bound to their specific receptors on the cells, lead to an increase in a cell population. Two factors contribute to this effect: 1. They enhance cell survival. Low concentrations are required to keep cells viable and prevent them from automatically undergoing apoptosis. Obviously the more viable cells there are present, the more cells are available to respond to proliferative signals. 2. They stimulate proliferation by allowing the cell cycle to proceed from G0 to G1 or to continue through G1. In addition, some growth factors have effects on mature cells which prime them for action and enhance their efficiency. At least 20 haemopoietic growth factors have been characterised and cloned. They act in conjunction with each other with more than 1 factor being required for the control of each lineage and most factors in turn being involved in more than 1 lineage. Specific haematological growth factors (Notes in italic are for interest only) 1. Erythropoietin This is the major growth factor involved in the control of red cell production (erythropoiesis). It is synthesized mainly in the kidney but small amounts also come from the liver. The main proliferative action occurs at the level of the CFU-E (see previous diagram) and to a lesser extent on the more primitive Burst Forming Unit (BFU-E). More of these cells are produced than are required during steady state conditions and many apoptose, but a surge of Erythropoietin in response to hypoxia allows them to survive and proliferate. It is also required for the survival of early normoblasts. 2. Interleukin-3 (IL-3) or Multi-Colony Stimulating Factor (CSF) This is produced by activated T lymphocytes and, as its name suggests, is involved in the control of proliferation of the early stages of many cell lineages. It acts together with other growth factors as follows:- IL-3 + GM-CSF and G-CSF produces neutrophils IL-3 + M-CSF produces monocytes IL-3 + Erythropoietin produces red cells IL-3 + IL-5 produces eosinophils IL-3 + thrombopoietin produces megakaryocytes 3. GM-CSF This is synthesised in T lymphocytes, endothelial cells, fibroblasts and macrophages. In addition to its effect on neutrophil and monocyte precursors, it stimulates production of eosinophils, megakaryocytes and red cells. It also primes mature neutrophils by increasing their chemotactic ability and upregulating adhesion molecules. 4. G-CSF This is synthesised by monocytes and fibroblasts. It has a far narrower range of activities than GM-CSF, acting only on cells committed to the neutrophil lineage. It also primes mature neutrophils and upgrades their killing abilities. Because it is more specific it has become very useful clinically to stimulate neutrophil production in neutropenic patients without producing adverse side effects. 5. Thrombopoietin This is made in the liver by hepatic macrophages. It acts at the level of CFU-Meg to produce megakaryocytes and hence platelets. C. The Marrow Microenvironment Normal haemopoiesis occurs only in the bone marrow in postnatal life. If a transfusion of primitive haemopoietic precursors is given intravenously as part of a bone marrow transplant, the cells obviously circulate to all parts of the body but it is only in the bone marrow that the cells develop functional, ongoing haemopoiesis. Why? Control of the haemopoietic cells requires a very specific microenvironment which supports the cells physically and nutritionally, delivers the growth factors appropriately, and releases the cells into the blood only when they are sufficiently mature. A number of cells in the marrow play important parts in this microenvironment. 1. Fibroblasts, stromal and “adventitial cells” These form the physical scaffold of the marrow but also produce some of the growth factors. They secrete adhesion molecules such as fibronectin which “glue” the haemopoietic cells into the marrow until they are mature. 2. Macrophages These are produced in the marrow from monocytes, themselves a product of haemopoiesis. They produce growth factors and regulate iron which is essential for red cell development. 3. Vascular endothelium Haemopoietic cells enter the circulation by means of special vascular sinuses which run between cords of developing haemopoietic cells. The endothelium of these sinuses allows the passage of mature cells through endothelial cells, not between them. The endothelium also produces some growth factors. 4. Adipose cells These cells shrink or expand as required to maintain the marrow volume as the haemopoietic cells increase or decrease in response to requirements. They seem to be more important than just space fillers as they form an essential component of long term bone marrow cultures which undergo active haemopoiesis, and they contain a specific form of fat which does not metabolise in the same way as normal adipose tissue fat does. These cells and the haemopoietic cells are organised into cords separated by venous sinuses. Numerous adhesion molecules occur in association with the cells and form an extracellular matrix. The developing cells have receptors for these molecules which they then lose with maturity – until then molecules such as fibronectin, laminin, collagen, vitronectin and von Willebrand’s factor “lock” the immature cells into the marrow. How do haemopoietic cells mature? Immature but fully differentiated haemopoietic cells are very different from the mature forms which circulate in the blood. In order to understand this, it is strongly advised that you make use of the online haematology atlases and Google images to illustrate the following notes. 1. Neutrophil maturation The first recognisable neutrophil precursor is the blast, a cell with a round nucleus and very little cytoplasm, while the mature neutrophil has a 3-lobed nucleus and a large amount of cytoplasm. Obviously considerable change occurs during maturation. While this change is a fairly continuous process, it is convenient to identify and name certain stages as the properties of the cell differ with the degree of maturation. The blast has a large nucleus in which the chromatin looks “primitive” – it still is using numerous genes, must be able to replicate the DNA and the chromatin is not yet condensed. Blasts are actively dividing cells. The next stage is referred to as the promyelocyte. It is still an actively dividing cell but has started to make more cytoplasm and the nucleus is eccentric in position. There are some primary granules in the cytoplasm – little packets of enzymes required for the microbial killing function of the neutrophil. The myelocyte is the last stage of maturation capable of division. There is even more cytoplasm which now contains secondary granules in addition to the primary granules. Secondary granules are specific to the granulocyte type i.e. there are neutrophilic, eosinophilic and basophilic granules each occurring in a specific cell. Secondary granules also contain enzymes required for antimicrobial functions. Metamyelocytes start to condense their chromatin and have a bean-shaped nucleus. Band cells have a condensed, horseshoe-shaped nucleus. The cell is functional and can be released into the blood if there is an exceptional need for neutrophils. The mature neutrophil has a nucleus which looks like a string of beads with lobes separated by thin strands of chromatin. The function of the neutrophil is to migrate through the tissues to find and kill pathogens – this shape of the nucleus makes it flexible and able to squeeze through small gaps without disrupting the tissues. 2. Red cell maturation The first recognisable red cell precursor is again the blast, which has a large nucleus and very little cytoplasm, while the mature red cell has no nucleus and consists entirely of haemoglobin-rich cytoplasm. The maturation process therefore consists of the production of large quantities of haemoglobin and the extrusion of the nucleus from the cell. The developing red cell is known as a normoblast and the stages of development are not as clearly divided as they are in the granulocytes, rather they are known as early or late normoblasts. The cytoplasm starts off as scanty and is deeply basophilic because of all the ribosomes and RNA required for haemoglobin (protein) synthesis. As the haemoglobin accumulates it dilutes the blue of the cytoplasm which becomes grey and then pink as the ribosomes and RNA are no longer needed. The nucleus becomes progressively darker and smaller until it is actively ejected from the cell before it leaves the marrow. Macrophages ingest the extruded nucleus and recycle its constituents. 3. Megakaryocyte maturation This also begins with a blast cell virtually indistinguishable from the blasts of the other cell line in appearances, but results in a very large cell with copious cytoplasm and a multilobed nucleus. The ploidy of the cell varies from 8 to 32N as the chromatin replicates without the nucleus dividing. The cytoplasm develops an extensive network of membranes within it which partition the cytoplasm into what will eventually become individual platelets. The platelets are released by fragmentation of the cytoplasm and the platelets, sometimes still joined together in chains, pass into the venous sinus. Large chunks of cytoplasm may be broken down further into platelets in the pulmonary capillaries.