Local Hormones Part 1 PDF
Document Details
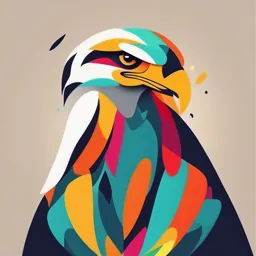
Uploaded by HelpfulHyperbola
Baghdad College of Pharmacy
Tags
Related
- UE12 Pharmacologie Moléculaire PDF
- 2024 Tyrosine Kinase Receptors x1 PDF
- Pharmacology Basic Principles AY 2023-2024 PDF
- Anti-Inflammatory, Antipyretic & Analgesic Pharmacology PDF
- Katzung Chapter 9: Adrenoceptor Agonists & Sympathomimetic Drugs PDF
- Molecular and Cellular Mechanisms of Drug Action PDF
Summary
This document provides an overview of local hormones, focusing on molecular pharmacology and the actions of histamine and prostanoids. It details various aspects of drug action and inflammatory responses. The document is suitable for undergraduate level study.
Full Transcript
Molecular Pharmacology Molecular pharmacology Deals with drug action at the molecular level rather than clinically observable action, although the latter stems from the former. When drugs bind to their receptors, they generally cause signal transduction by engagi...
Molecular Pharmacology Molecular pharmacology Deals with drug action at the molecular level rather than clinically observable action, although the latter stems from the former. When drugs bind to their receptors, they generally cause signal transduction by engaging second messengers that in turn activate kinases that affect the genome and proteins involved in cascades that carry out biological function. Binding affinity, intrinsic activity, efficacy, potency, signal transduction involving G proteins, coupling to receptors, activation of enzymes, opening of channels to allow the entry of ions, second messenger production that eventually affects biological pathways, structure‐activity relationship, drug metabolism, pharmacogenetics, pharmacokinetics, pharmacodynamics(drug mechanism of action), All are aspects of molecular pharmacology. Molecular pharmacology explores the reaction of drugs on human molecules and leads to the development of new medications LOCAL HORMONES WHAT IS A ‘MEDIATOR’? Like regular hormones, such as thyroxine or insulin , a local hormone is simply a chemical messenger that conveys information from one cell to another. That it is released from local cells in sufficient amounts to produce a biological action on the target cells within an appropriate time frame; that application of an authentic sample of the mediator reproduces the original biological effect; that interference with the synthesis, release or action (e.g. using receptor antagonists, enzyme inhibitors, ‘knock‐down’ or ‘knock‐out’ techniques) ablates or modulates the original biological response. HISTAMINE In a classic study, Dale and his colleagues demonstrated that a local anaphylactic reaction (a type I or ‘immediate hypersensitivity reaction’ such as the response to egg albumin in a previously sensitized animal; was caused by antigen–antibody reactions in sensitized tissue, and found that histamine mimicked this effect both in vitro and in vivo. Later studies confirmed that histamine is present in tissues, and released (along with other mediators) during anaphylaxis SYNTHESIS AND STORAGE OF HISTAMINE Histamine is a basic amine formed from histidine by histidine decarboxylase. It is found in most tissues but is present in high concentrations in tissues in contact with the outside world (lungs, skin and gastrointestinal tract). At the cellular level, it is found largely in mast cells (approximately 0.1–0.2 pmol/cell) and basophils (0.01 pmol/cell), but non‐mast cell histamine also occurs in ‘histaminocytes’ in the stomach and in histaminergic neurons in the brain In mast cells and basophils, histamine is complexed in intracellular granules with an acidic protein and a high molecular‐weight heparin termed macroheparin. HISTAMINE RELEASE Histamine is released from mast cells by exocytosis during inflammatory or allergic reactions. Stimuli include complement components C3a and C5a , which interact with specific surface receptors, and the combination of antigen with cell-fixed immunoglobulin (Ig)E antibodies. In common with many secretory processes , histamine release is initiated by a rise in cytosolic [Ca2+]. they cause smooth muscle contraction, vasodilation, histamine release from mast cells, and enhanced vascular permeability. They also mediate chemotaxis, inflammation, and generation of cytotoxic oxygen radicals. Various basic drugs, such as morphine and tubocurarine, release histamine, as does compound 48/80, an experimental tool often used to investigate mast cell biology. Agents that increase cAMP formation (e.g. β-adrenoceptor agonists, inhibit histamine secretion. Replenishment of secreted histamine by mast cells or basophils is a slow process, which may take days or weeks, whereas turnover of histamine in the gastric histaminocyte is very rapid. Histamine is metabolised by histaminase ( diamine oxidase ) and/or by the methylating enzyme imidazole N-methyltransferase HISTAMINE RECEPTORS Four types of histamine receptor have been identified, H1‐4. All are G protein–coupled receptors but their downstream signalling systems differ. H1 and H3 receptors, for example, elevate cAMP, whereas H2 and H4 receptors stimulate PLC. Splice variants of H3 and H4 receptors have been reported. All four are implicated in the inflammatory response in some capacity. Histamine H1 antagonists are the principal antihistamines used in the treatment or prevention of inflammation (notably allergic inflammation such as hay fever). The pharmacology of H4 receptors is less well developed but strongly suggests that the receptor has a significant role in the inflammatory response. Eosinophils seem to be a prominent target. ACTIONS Smooth muscle effects. Histamine, acting on H1 receptors, contracts the smooth muscle of the ileum, bronchi, bronchioles and uterus. The effect on the ileum is not as marked in humans as it is in the guinea pig (this tissue remains the de facto standard preparation for histamine bioassay). Histamine reduces air flow in the first phase of bronchial asthma, but H1 antagonists are not of much benefit in the human disease. It is possible that H4 receptors are more important in mediating these resistant histamine effects Cardiovascular effects. Histamine dilates human blood vessels and increases permeability of postcapillary venules, by an action on H1 receptors, the effect being partly endothelium‐dependent in some vascular beds. It also increases the rate and the output of the heart mainly by an action on cardiac H2 receptors. Gastric secretion. Histamine stimulates the secretion of gastric acid by action on H2 receptors. In clinical terms, this is the most important action of histamine, because it is implicated in the pathogenesis of peptic ulcer. Effects on skin. When injected intradermally, histamine causes a reddening of the skin, accompanied by a weal with a surrounding flare. This mimics the triple response to scratching of the skin, described by Sir Thomas Lewis over 80 years ago. The reddening reflects vasodilatation of the small arterioles and precapillary sphincters and the weal, the increased permeability of the postcapillary venules. These effects are mainly mediated through activation of H1 receptors. The flare is an axon reflex: stimulation of sensory nerve fibres evokes antidromic impulses through neighbouring branches of the same nerve, releasing vasodilators such as calcitonin gene-related peptide. The axon reflex is the spread of this impulse from the main axon to nearby blood vessels in the stimulated area of the skin. These impulses in the affected area release chemical agents that cause blood vessels to dilate and leak, causing the skin to sweat. Histamine causes intense itch if injected into the skin or applied to a blister base, because it stimulates sensory nerve endings through an H1-dependent mechanism. H1antagonists are used to control itch caused by allergic reactions, insect bites, etc Even though histamine release is manifestly capable of reproducing many of the inflammatory signs and symptoms, H1 antagonists do not have much clinical utility in the acute inflammatory response per se, because other mediators are more important. Histamine is, however, important in type I hypersensitivity reactions such as allergic rhinitis and urticaria. Other significant actions of histamine in inflammation include effects on B and T cells, modulating the acquired immune response It is possible that the developing field of H4 receptor pharmacology will fill in some significant gaps in our understanding of the role of histamine in inflammation in the near future EICOSANOIDS GENERAL REMARKS The term eicosanoid refers to a group of mediators that are generated from specific fatty acid precursors. They are implicated in the control of many physiological processes, are among the most important mediators and modulators of the inflammatory reaction and are a significant target for drug action. Interest in eicosanoids first arose in the 1930s after reports that semen contained a lipid substance, apparently originating from the prostate gland, which contracted uterine smooth muscle. Later, it became clear that prostaglandin (as the factor was named) was not a single substance but a whole family of compounds generated by virtually all cells from 20‐carbon unsaturated fatty acid precursors STRUCTURE AND BIOSYNTHESIS The main eicosanoid precursor is arachidonic acid (5,8,11,14‐eicosatetraenoic acid), a 20‐carbon unsaturated fatty acid containing four unsaturated double bonds (hence the prefix eicosa‐, referring to the 20 carbon atoms, and tetra‐enoic, referring to the four double bond. The principal groups of eicosanoids are prostaglandins, thromboxanes, leukotrienes, lipoxins and resolvins. The common term prostanoids refers to prostaglandins and thromboxanes only In most cell types, arachidonic acid is a component of phospholipids and the intracellular concentration of the free acid is low. Eicosanoids are not stored in cells (like histamine, for example) but are synthesized and released immediately. The initial and rate‐limiting step in eicosanoid synthesis is therefore the liberation of arachidonate. Usually this is a one‐step process catalysed by the enzyme phospholipase A2 , but an alternative multi‐step process involving phospholipases C or D in conjunction with diacylglycerol lipase is sometimes utilised. Several types of PLA2 exist, but the most important is probably the highly‐regulated cytosolic PLA2 (cPLA2). This enzyme not only generates arachidonic acid (and thus eicosanoids) but also lysoglyceryl‐phosphorylcholine (lyso‐PAF), the precursor of platelet‐activating factor (PAF), another inflammatory mediator Cytosolic PLA2 is activated by phosphorylation and this may be triggered by signal transduction systems activated by many stimuli, such as thrombin action on platelets, C5a on neutrophils, bradykinin on fibroblasts antigen– antibody reactions on mast cells. General cell damage also triggers cPLA2 activation. The free arachidonic acid (which normally exists as arachidonate in solution) is metabolised separately (or sometimes jointly) by several pathways, including the following Fatty acid cyclo‐oxygenase (COX). Two main isoforms exist, COX‐1 and COX‐2. These are highly homologous enzymes but are regulated in different and tissue‐specific ways. They enzymatically combine arachidonic (and some other unsaturated fatty acid) substrates with molecular oxygen to form unstable intermediates, cyclic endoperoxides, which can subsequently be transformed by other enzymes to different prostanoids. Lipoxygenases. There are several subtypes, which often work sequentially, to synthesise leukotrienes, lipoxins and other compounds PROSTANOIDS COX‐1 is present in most cells as a constitutive enzyme. It produces prostanoids that act mainly as homeostatic regulators (e.g. modulating vascular responses, regulating gastric acid secretion). COX‐2 is not normally present (at least in most tissues – CNS and renal tissue are important exceptions) but it is strongly induced by inflammatory stimuli and therefore believed to be more relevant as a target for anti‐inflammatory drugs. Both enzymes catalyse the incorporation of two molecules of oxygen into two of the unsaturated double bonds in each arachidonate molecule. forming the highly unstable endoperoxides prostaglandin (PG)G2 and PGH2. The suffix ‘2’ indicates that the product contains only two double bonds. PGG2 and PGH2 are rapidly transformed in a tissue‐specific manner by endoperoxide isomerase or synthase enzymes to PGE2, PGI2 (prostacyclin), PGD2, PGF2α and thromboxane (TX)A2, which are the principal bioactive end products of this reaction. The mix of eicosanoids thus produced varies between cell types, depending on the particular endoperoxide isomerases or synthases present. In platelets, for example, TXA2 predominates, whereas in vascular endothelium PGI2 is the main product. Macrophages, neutrophils and mast cells synthesise a mixture of products If eicosatrienoic acid (three double bonds) rather than arachidonic acid is the substrate for these enzymes, the resulting prostanoids have only a single double bond, for example PGE1, while eicosapentaenoic acid, which contains five double bonds, yields PGE3. Eicosapentaenoic acid is abundant in diets rich in oily fish and may, if present in sufficient amounts, represent a significant fraction of cellular fatty acids and thus constitute the major source of precursors for the COX enzyme. When this occurs, the production of the pro‐inflammatory PGE2 is diminished and, more significantly, the generation of TXA2 as well. This may partly underlie the beneficial anti‐inflammatory and cardiovascular actions that are ascribed to diets rich in this type of marine product. CATABOLISM OF THE PROSTANOIDS This is a multi‐step process. After carrier‐mediated uptake, most prostaglandins are rapidly inactivated by prostaglandin dehydrogenase and reductase enzymes. These enzymes oxidise the 15‐hydroxyl group , and the 13‐14 double bond, both of which are important for biological activity. The inactive products are further degraded by general fatty acid‐oxidising enzymes and excreted in the urine. These dehydrogenase enzymes are present in high concentration in the lung, and 95% of infused PGE2, PGE1 or PGF2α is inactivated after a single passage through the lungs, meaning that little normally reaches the arterial circulation and for this reason the half‐life of most prostaglandins in the circulation is less than 1 minute. TXA2 and PGI2 are slightly different. Both are inherently unstable and decay rapidly and spontaneously (within 30 s and 5 min, respectively) in biological fluids into inactive TXB2 and 6‐keto‐PGF1α, respectively. Further metabolism then occurs, but it is not really relevant to us here. PROSTANOID RECEPTORS There are five main classes of prostanoid receptor , all of which are G protein–coupled receptors. They are termed DP, FP, IP, EP and TP receptors, respectively, depending on whether their ligands are PGD, PGF, PGI, PGE or TXA species. Some have further subtypes; for example, there are four EP receptors. ACTIONS OF THE PROSTANOIDS The prostanoids can affect most tissues and exert a bewildering variety of effects. PGD2 causes vasodilatation in many vascular beds, inhibition of platelet aggregation, relaxation of gastrointestinal and uterine muscle, and modification of release of hypothalamic/pituitary hormones. It has a bronchoconstrictor effect through a secondary action on TP receptors. It may also activate chemoattractant receptors on some leukocytes. PGF2α causes uterine contraction in humans , luteolysis in some species (e.g. cattle) and bronchoconstriction in others (e.g. cats and dogs). PGI2 causes vasodilatation, inhibition of platelet aggregation , renin release and natriuresis through effects on tubular reabsorption of Na+. TXA2 causes vasoconstriction, platelet aggregation and bronchoconstriction (more marked in guinea pig than in humans). PGE2, the predominant ‘inflammatory’ prostanoid has the following actions: at EP1 receptors, it causes contraction of bronchial and gastrointestinal smooth muscle; at EP2 receptors, it causes bronchodilatation, vasodilatation, stimulation of intestinal fluid secretion and relaxation of gastrointestinal smooth muscle; at EP3 receptors, it causes contraction of intestinal smooth muscle, inhibition of gastric acid with increased mucus secretion, inhibition of lipolysis, inhibition of autonomic neurotransmitter release and contraction of the pregnant human uterus. at EP4 receptors, it causes similar effects to those of EP2 stimulation (these were originally thought to be a single receptor). Vascular relaxation is one consequence of receptor activation, as is cervical ‘ripening’. Some inhibitory effects of PGE2 on leukocyte activation are probably mediated through this receptor. Several clinically useful drugs act at prostanoid receptors. These include misoprostol, an EP2/EP3 agonist that suppresses gastric acid secretion , the FP agonists bimatoprost, latanoprost, tafluprost and travoprost which are used for the treatment of glaucoma and iloprost and epoprostanol which are IP agonists used for the treatment of pulmonary hypertension THE ROLE OF PROSTANOIDS IN INFLAMMATION The inflammatory response is inevitably accompanied by the release of prostanoids. PGE2 predominates, although PGI2 is also important. In areas of acute inflammation, PGE2 and PGI2 are generated by the local tissues and blood vessels, while mast cells release mainly PGD2. In chronic inflammation, cells of the monocyte/macrophage series also release PGE2 and TXA2. Together, the prostanoids exert a sort of yin–yang effect in inflammation, stimulating some responses and decreasing others. The most striking effects are as follows PGE2, PGI2 and PGD2 are themselves powerful vasodilators but they also synergise with other inflammatory vasodilators, such as histamine and bradykinin. It is this combined dilator action on precapillary arterioles that contributes to the redness and increased blood flow in areas of acute inflammation. Prostanoids do not directly increase the permeability of the postcapillary venules, but potentiate the effects on vascular leakage caused by histamine and bradykinin. Similarly, they do not themselves produce pain, but sensitise afferent C fibres to the effects of bradykinin and other noxious stimuli. The anti‐inflammatory and analgesic effects of aspirin‐like drugs (NSAIDs, stem largely from their ability to block these actions. Prostaglandins of the E series are also pyrogenic (i.e. they induce fever). High concentrations are found in cerebrospinal fluid during infection, and the increase in temperature (attributed to cytokines) is actually mediated by the release of PGE2. NSAIDs exert antipyretic actions by inhibiting PGE2 synthesis in the hypothalamus. Some prostaglandins have anti‐inflammatory effects which are important during the resolution phase of inflammation. For example, PGE2 decreases lysosomal enzyme release and the generation of toxic oxygen metabolites from neutrophils, as well as the release of histamine from mast cells.