Lippincott's Biochemistry Chapter 19 - Amino Acids (Nitrogen Disposal) PDF
Document Details
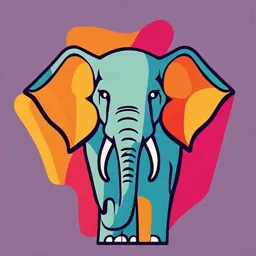
Uploaded by PrizeMeerkat
Tags
Summary
This document is Chapter 19 of Lippincott's Biochemistry, focusing on amino acids and nitrogen disposal. It explains the role of amino acids in various metabolic processes, including protein turnover and the urea cycle.
Full Transcript
Amino Acids: Nitrogen Disposal I. OVERVIEW Unlike fats and carbohydrates, amino acids are not stored by the body. That is, no protein exists whose sole function is to maintain a supply of amino acids for future use. Therefore, amino acids must be obtained from the diet, synthesized de novo, or pro...
Amino Acids: Nitrogen Disposal I. OVERVIEW Unlike fats and carbohydrates, amino acids are not stored by the body. That is, no protein exists whose sole function is to maintain a supply of amino acids for future use. Therefore, amino acids must be obtained from the diet, synthesized de novo, or produced from the degradation of body protein. Any amino acids in excess of the biosynthetic needs of the cell are rapidly degraded. The first phase of catabolism involves the removal of the a-amino groups (usually by transamination and subse- quent oxidative deamination), forming ammonia and the corresponding a-keto acids, the carbon skeletons of amino acids. A portion of the free ammonia is excreted in the urine, but most is used in the synthesis of urea (Fig. 19.1 ), which is quantitatively the most important route for dis- posing of nitrogen from the body. In the second phase of amino acid catabolism, described in Chapter 20, the carbon skeletons of the a-keto acids are converted to common intermediates of energy-producing met- abolic pathways. These compounds can be metabolized to carbon diox- ide (C02) and water (H2'>), glucose, fatty acids, or ketone bodies by the central pathways of metabolism described in Chapters 8-13 and 16..... HCC>a- II. OVERALL NITROGEN METABOLISM T Cutlamoyl phoephala A..-1* Amino acid catabolism is part of the larger process of the metabolism of nitrogen-containing molecules. Nitrogen enters the body in a variety of compounds present in food, the most important being amino acids con- r l. Otnlthlne CltNllln~ Arglnlnosucclnate ) u~tnlne tained in dietary protein. Nitrogen leaves the body as urea, ammonia, and other products derived from amino acid metabolism (such as creatinine, see p. 287). The role of body proteins in these transformations involves two important concepts: the amino acid pool and protein turnover. Figure 19.1 A. Amino acid pool Urea cycle shown as part of the essential pathways of energy Free amino acids are present throughout the body, such as in cells, metabolism. [Note: See Fig. 8.2, p. 92, blood, and the extracellular fluids. For the purpose of this discussion, for a more detalled map of metabollsm.] NHa =ammonia; 002 =carbon dioxide. 245 246 19. Amino Acids: Nitrogen Disposal envision all of these amino acids as if they belonged to a single entity, TURNOVER called the amino acid pool. This pool is supplied by three sources: 1) Protein turnover I the 1lmultaneou amino acids provided by the degradation of endogenous (body) pro- syntheale and degradation of protein teins, most of which are reutilized; 2) amino acids derived from exog- molecules. In h lthy, fed adults, the total amount of protein In the body enous (dietary) protein; and 3) nonessential amino acids synthesized remalne conetant becauae the rate from simple intermediates of metabolism (Fig. 19.2). Conversely, the of protein synth881a le Juet sufficient amino acid pool is depleted by three routes: 1) synthesis of body to replace the protein that la degraded. protein, 2) consumption of amino acids as precursors of essential nitrogen-containing small molecules, and 3) conversion of amino DleWy problln can vary acids to glucose, glycogen, fatty acids, and ketone bodies or oxidation from none (for example, to C800 g/day (comprising -90-100 g of amino acids) in comparison with the amount (high-protein dim). but 100 g/day le typical of the of protein in the body {-12 kg in a 70-kg man), it is conceptually at the U.S. diet. center of whole-body nitrogen metabolism. Synlheels of noneuentlal In healthy, well-fed individuals, the input to 1he amino acid amlnoaclda pool Is balanced by 1he output. That Is, the amount of amino acids contained In the pool Is constant. The amino acid pool is said to be in a steady state, and the individual is said to be in nitrogen balance (see p. 367). B. Protein turnover Most proteins in 1he body are constantly being synthesized and then degraded (turned over), permitting the removal of abnormal or unneeded proteins. For many proteins, regulation of synthesis deter- Body Synllleela of: protein mines the concentration of protein in the cell, with protein degrada- Porphyrtna -400gfday Creidlne tion assuming a minor role. For other proteins, the rate of synthesis Neurotnmemlttere is constitutive (that is, essentially constant}, and cellular levels of the Purtnea protein are controlled by selective degradation. Pyrimidines 01tl9r n!tJogen- contelnlng 1. Rate: In healthy adults, the total amount of protein in the body compounda Varlee remains constant because the rate of protein synthesis is just sufficient to replace the protein that is degraded. This process, called protein turnover, leads to the hydrolysis and resynthesis of 3D0-400 g of body protein each day. The rate of protein turn- GluCOM, Ketone bodlas, glycogen fatty acfds over varies widely for individual proteins. Short-lived proteins {for example, many regulatory proteins and misfolded proteins) are rapidly degraded, having half-lives measured in minutes or hours. Long-lived proteins, with hatf-lives of days to weeks, constitute the majority of proteins in the cell. Structural proteins, such as col- lagen, are metabolically stable and have half-lives measured in months or years. lhe amino acid not UHd In blosynthetlc reactions are 2. Protein degradation: There are two major enzyme systems burned ae a fUel. responsible for degrading proteins: the ATP-dependent ubiquitin (Ub)-proteasome system of the cytosol and the ATP-independent degradative enzyme system of the lysosomes. Proteasomes Figure 19.2 selectively degrade damaged or short-lived proteins. Lysosomes Sources and fates of amino acids. use acid hydrolases (see p. 162) to nonselectively degrade intra- [Note: Nitrogen from amino acid degradation is released as ammonia, cellular proteins (autophagy) and extracellular proteins (het- which is converted to urea and erophagy), such as plasma proteins, that are taken into the cell excreted.]~= carton dioxide. by endocytosis. Ill. Dietary Protein Digestion 247 a. Ublqultln-proteasome system: Proteins selected for degra- dation by the cytosolic ubiquitin--proteasome system are first modified by the covalent attachment of Ub, a small, globular, 0 Protein ealaclDd for degradation Is tagaed wHh molecules of ublqullln (an AlP-dependent procau). nonenzymic protein that is highly conserved across eukaryotic species. Ubiquitination of the target substrate occurs through E'I Ublqultlnated proteins isopeptide linkage of the a-carboxyl group of the C-terminal gly- U are recognized by the CYtosollC proteuome, cine of Ub to thee-amino group of a lysine in the protein substrate Which unfolds,~ ublqulllnatM, and by a three-step, enzyme-catalyzed, ATP-dependent process. tnaneports tt:19proleln [Note: Enzyme 1 (E1, an activating enzyme) activates Ub, which to Its p~lytfc core (aleo an AlP· is then transferred to E2 (a conjugating enzyme). E3 (a ligase) dependent proc:aa). identifies the protein to be degraded and interacts with Eft.Ub. There are many more E3 proteins than there are E1 or E2.] The consecutive addition of four or more Ub molecules to the target protein generates a polyubiquitin chain. Proteins tagged with Ub chains are recognized by a large, barrel-shaped, macromolec- ular, proteolytic complex called a proteasome (Fig. 19.3). The proteasome unfolds, deubiquitinates, and cuts the target pro- tein into fragments that are then further degraded by cytosolic prot9BSes to amino acids, which enter the amino acid pool. The Ub is recycled. It is noteworthy that the selective degradation of proteins by the ubiquitin--proteosome complex (unlike simple hydrolysis by proteolytic enzymes) requires ATP hydrolysis. b. Degradation slgnals: Because proteins have different half- lives, it is clear that protein degradation cannot be random but, rather, is influenced by some structural aspect of the protein that serves as a degradation signal, which is recognized and bound 0 00 by an £3. The half-life of a protein is also influenced bythe amino 0 Ublqultln (N}-terminal residue, the so-.--/ ;f NHa Succ1~ CoA ,: chondrial matrix and the cytosol.] ;( leoleuclne, Velln Amlnoaclde 1. Carbamoyl phosphate formation: Formation of carbamoyl --- - phosphate by carbamoy/ phosphate synthetase I (CPS I) is driven by cleavage of two molecules of ATP. Ammonia incorporated into cart>amoyt phosphate is provided primarily by the oxidative deamination of glutamate by mitochondrial GOH (see Fig. 19.11 ). Figure 19.13 Uttimately, the nitrogen atom derived from this ammonia becomes Transport of ammonia (NH3) from one of the nitrogens of urea. CPS I requires N-acetylglutamate = muscle to the liver. ADP adenosine (NAG) as a positive allosteric activator (see Fig. 19.14). [Note: diphosphate; Pi =inorganic Carbamoy/ phosphate synthetase II participates in the biosynthesis phosphate; CoA =coenzyme A. 254 19. Amino Acids: Nitrogen Disposal n Tluuee other titan liver ll8e enzymee U of thla pathway to make arginine. H, ,coo- CYTOSOL C l;fH2 II c C=NHt , ' I Fumsrase-ooc H NH I H~ Malate - - - - Fumuete 9H2 yH2 n Ornlthlne.. regenerated ~and transported Into the CH2 mltochondrton. HCNH3 + I C()(J MITOCHONDRIAL MATRIX L-Arglnlne ~Ha+ ~Ha+ N H+ coo- , 3 ' C =N - CH 9~ 9~ ' I c~ c:::::=:::;::;:::==~ c;:~ ~H yH2 c~ c~ yH2 coo- HCNH3+ HCNH3+ yH2 6oo- coo- CH2 L-OmHhlne L-OmHhlne HCNH ' 3 + coo- A11Jlnlnoeucclnale F.11 Cltrulllne la ayntheelzed Iii.I and transported out of -o P=O 1he mltocltondrlon. o- C.rbamovl phoephafe f;IH2 c;»=o t;JH ~Hi! c~ yH2 6~ CH2 H9NH3+ HCNH3+ 2ADP coo- coo- ccxr L·CltJ'ulllne L-Cttndllne · HaN -CH I r.3 The amino group of yH2 1:.1 aepartate pi'ovld one of coo- the nHrogen atoma of urea. HCOa- L Allpartate + MD 0 camon atom Bicarbonate provldela 1118 of urea. N H3 + NADH+H+ 2ATP T Oxaloaceta:te n U Free ammonia provld one of the nitrogen atoms of urea. n The enzyme has an absolute IE:J requirement for N-e.c:etyl· l'3 Fumande la hydrated to glutamate, which llct9 ~ melate, which Is oxidized as an alloeterlc activator. to oxaloacetate that la tranumlnated 10 aapartate. Figure 19.14 Reactions of the urea cycle. [Note: An antiporter transports citrulline and ornithine across the inner mitochondrial membrane.] ADP"' adenoeine diphosphate: AMP= adenosine monophosphate; PP;"' pyrophosphate; Pi= inorganic phosphate; NAD(H) =nicotinamide adenine dinucleotide; MD= mslate dehydrogensse. V. Urea Cycle 255 of pyrimidines (seep. 302). It does not require NAG, uses glutamine as 1he nitrogen source, and occurs in the cytosol.] 2. Cltrulllne formation: The carbamoyl portion of carbamoyl phos- phate is transferred to ornithine by ornithine transcarbamylase (OTC) as the phosphate is released as inorganic phosphate. The reaction product, citrulline, is transported to the cytosol. [Note: Ornithine and citrulline move across the inner mitochondrial membrane via an antiporter. These basic amino acids are not Transamlnatlon Oxidative incorporated into cellular proteins because there are no codons deamlnatlon for them (see p. 447).) Ornithine is regenerated with each turn of the urea cycle, much in the same way that oxaloacetate is regenerated by the reactions of the tricarboxylic acid (TCA) cycle (seep. 109). 3. Arglnlnosucclnate formation: Argininosuccinate synthetase combines citrulline with aspartate to form argininosuccinate. The a-amino group of aspartate provides 1he second nitrogen that is g,_ ultimately incorporated into urea. The formation of argininosucci- ~ HCO,- nate is driven by the cleavage of ATP to adenosine monophos- Fumarate Arginine \{ phate and pyrophosphate. This is the 1hird and final molecule of ATP consumed in the formation of urea. l\.1 UREA Omfthlne. _\{ CYCLE ) Atgininoauccinate v-;::: 4. Arglnlnosucclna:te cleavage: Argininosuccinate is cleaved by lt Clllbllmoyl ~ Cltrulllne phoepl'lete argininosuccinate lyase to yield arginine and fumarate. The argi- nine serves as the immediate precursor of urea. The fumarate is hydrated to malate, providing a link with several metabolic path- ways. Malate can be oxidized by ma/ate dehydrogenaseto oxaloac- etate, which can be transaminated to aspartate (see Fig. 19.8) and enter the urea cycle (see Fig. 19.14). Alternatively, malate can be Figure 19.15 transported into mitochondria via the malate-aspartate shuttle Flaw of nitrogen from amino acids (see p. 80), reenter the TCA cycle, and get oxidized to oxaloace- to urea. Amino groups for urea tate, which can be used for gluconeogenesis (see p. 120). [Note: synthesis are collected in the form Malate oxidation generates NADH for oxidative phosphorylation of ammonia (NH3) and aspartate. (see p. 77}, thereby reducing the energy cost of the urea cycle.] NAD{H) =nicotinamide adenine dlnucleotlde; HCOa- =bicarbonate. 5. Arginine cleavage to omlthlne and urea: Arginase-1 hydrolyzes arginine to ornithine and urea and is virtually exclusive to the liver. Therefore, only the liver can cleave arginine, thereby synthesizing urea, whereas other tissues, such as the kidney, can synthesize arginine from citrulline. [Note: Arginase-11 in kidneys controls argi- nine availability for nitric oxide synthesis (see p. 150).] 6. Fate ot urea: Urea diffuses from the liver and is transported in the blood to 1he kidneys, where it is filtered and excreted in the urine {see Fig. 19.19). A portion of the urea diffuses from the blood into the intestine and is cleaved to C02 and ammonia by bacterial urease. The ammonia is partly lost in the feces and is partly reab- sorbed into the blood. In patients with kidney failure, plasma urea levels are elevated, promoting a greater transfer of urea from blood into the gut. The intestinal action ot urease on this urea becomes Figure 19.16 a clinically important source of ammonia, contributing to the hyper- Formation and degradation of ammonemia often seen in 1hese patients. Oral administration of N-acetylglutamate, an allosterlc antibiotics reduces the number of intestinal bacteria responsible activator of csrbamoyt phosphate for this ammonia production. = synthetase I. CoA coenzyme A. 256 19. Amino Acids: Nitrogen Disposal B. Overall stoichiometry qo - NH2 CH2 Aspartate+NHa +Hco3- +3ATP+H..z0~ urea + fumarate + 2 ADP + AMP + 2 P. + PP. I 9H2 HCNH3+ I Because four high-energy phosphate bonds are consumed in the syn- coo- 1hesis of each molecule of urea, the synthesis of urea is irreversible, Glutamlne with a large, negative AG (see p. 70). One nitrogen of the urea mole- cule is supplied by free ammonia and the other nitrogen by aspartate. Glutamate is the immediate precursor of both ammonia (through oxi- dative deamination by GDH) and aspartate nitrogen (through trans- amination of oxaloacetate by AS7). In effect, both nitrogen atoms of coo- l urea arise from glutamate, which, in turn, gathers nitrogen from other 9H2 amino acids (Fig. 19.15). 9H2 HCNH I 3 + C. Regulation coo- Glutamate NAG is an essentiaJ activator for CPS I, the rate-limiting step in the urea cycle. It increases the affinity of CPS I for ATP. NAG is synthe- sized from acetyl CoA and glutamate by N-acety/gfutamate synthase Figure 19.17 (NAGb), as shown in Figure 19.16, in a reaction for which arginine Hydrolysis of glutamine to form is an activator. The cycle is also regulated by substrate availability ammonia {NHs). (short-term regulation) and enzyme induction (long term). VI. AMMONIA METABOLISM Ammonia is produced by all tissues during the metabolism of a vari- ety of compounds, and it is disposed of primarily by formation of urea in the liver. However, the blood ammonia level must be kept very low, because even slightly elevated concentrations (hyperammonemia) are toxic to the central nervous system (CNS). Therefore, a mechanism is required tor the transport of nitrogen from the peripheral tissues to the liver for ultimate disposal as urea while keeping circulating levels of free coo- l ammonia low. yH2 yH2 A. Sources HCNH I 1,+ coo- Amino acids are quantitatively the most important source of ammonia Glutamate because most Western diets are high in protein and provide excess ATP+ NHa amino acids, which travel to the liver and undergo transdeamination Glut.amine {that is, the linking of the aminotransferase and GDH reactions), pro- S)'l'llhel'llN ducing ammonia. [Note: The liver catabolizes straight-chain amino ADP+P1 acids, primarily.] However, substantial amounts of ammonia can be CO - NHa obtained from other sources. I yH2 1. Glula.mlne: An important source of plasma glutamine is from the yH2 ca.tabolism of BCAA in skeletal muscle. This glutamine is taken HCNH3+ I up by cells of the intestine, the liver, and the kidneys. The liver coo- and kidneys generate ammonia from glutamine by the actions of Glutamlne glut.a.minase (Fig. 19.17) and GDH. In the kidneys, most of this ammonia is excreted into the urine as NH4+, which provides an Figure 19.18 important mechanism for maintaining the body's acid-base bal- Synthesis of gtutamlne. ADP = ance through the excretion of protons. In the liver, the ammonia is adenosine diphosphate; P1 = detoxified to urea and excreted. [Note: a-Ketoglutarate, the second inorganic phosphate; NHa = product of GDH, is a glucogenic precursor in the liver and kidneys.] ammonia. VI. Ammonia Metabolism 257 Ammonia is also generated by intestinal glutaminase. Enterocytes obtain glutamine either from the blood or from digestion of dietary METABOLISM protein. [Note: Intestinal glutamine metabolism also produces ala- nine, which is used by the liver for gluconeogenesis, and citrulline, which is used by the kidneys to synthesize arginine.] if 2. Intestinal bacterta: Ammonia is formed from urea by the action of bacterial urease in the lumen of the intestine. This ammonia is Glutamale absorbed from the intestine by way of the portal vein, and virtually deb~ all is removed by the liver via conversion to urea. 3. Amines: Amines obtained from the diet and monoamines that serve as hormones or neurotransmitters give rise to ammonia by the action of monoamine oxidase (see p. 286). -- 11' A ~ ca-Ketoglutarate NAD(P)H 4. Purines and pyrimidines: In the catabolism of purines and DIET 11 pyrimidines, amino groups attached to the ring atoms are released BODY PROTEIN as ammonia (see Fig. 22.15 on p. 300). B. Transport In the clrculatlon Glutamate+ ATP ~ mine AHhough ammonia is constantly produced in the tissues, it is present at very low levels in blood. This is due both to the rapid removal of synthBtase blood ammonia by the liver and to the fact that several tissues, par- ADP+P1 ticularly muscle, release amino acid nitrogen in the form of glutamine and alanine, rather than as free ammonia (see Fig. 19.13). Glutamlne 1. Urea: Formation of urea in the liver is quantitatively the most important disposal route for ammonia. Urea travels in the blood from the liver to the kidneys, where it passes into the glomerular filtrate. HP Glutamal9 2. Glutamlne: This amide of glutamate provides a nontoxic storage and transport form of ammonia (Fig. 19.18). The ATP-requiring for- v mation of glutamine from glutamate and ammonia by glutamine Amide nitrogen donated in synthetase occurs primarily in skeletal muscle and the liver but is biosynthetic also important in the CNS, where it is the major mechanism for the reactions + NH4 removal of ammonia in the brain. Glutamine is found in plasma at concentrations higher than other amino acids, a finding consistent with its transport function. [Note: The liver keeps blood ammonia URINE It'> Carbamoyl phosphate levels low through glutaminase, GDH, and the urea cycle in peri- portal (close to inflow of blood) hepatocytes and through glutamine {j syntfJetase I UNecycle synthetase as an ammonia scavenger in the perivenous hepato- Urea- - ' cytes.] Ammonia metabolism is summarized in Figure 19.19. (BLOOD) c. Hyperammonamla The capacity of the hepatic urea cycle exceeds the normal rates of Figure 19.19 ammonia generation, and the levels of blood ammonia are normally Ammonia (NH3) metabolism. Urea low (5-35 µmoVI). However, when liver function is compromised, due content in 1he urine is reported either to genetic defects of the urea cycle or liver disease, blood as urinary urea nitrogen, or UUN. Urea in blood is reported as BUN levels can be >1,000 µmolll. Such hyperammonemia is a medical (blood urea nitrogen). (Note: The emergency, because ammonia has a direct neurotoxic effect on enzymes glutamate dehydrogenass, the CNS. For example, elevated concentrations of ammonia in the gfutamine synthetass, and carbamoyl blood cause the symptoms of ammonia intoxication, which include phosphate synthetase I fix NHs into tremors, slurring of speech, somnolence (drowsiness), vomiting, organic molecules.] cerebral edema, and blurring of vision. At high concentrations, 258 19. Amino Acids: Nitrogen Disposal ammonia can cause coma and death. There are two major types of Phenylbutyrate I prodrug thm I hyperammonemia. rapldly converted to phenylaeetate, which combines wltl! glutamlne to form phenylacetylglutamlne. The 1. Acquired: Liver disease is a common cause of acquired hyper- phenylacetyglutamlne, containing two ammonemia in adults and may be due, for example, to viral hepa- atoma ot nitrogen, I excr81ed In Ille titis or to hepatotoxins such as alcohol. Cirrhosis of the liver may urine, thereby uel llng In clearance ot nltrogenou wute. result in formation of collateral circulation around the liver. As a result, portal blood is shunted directly into the systemic circulation and does not have access to the liver. Therefore, the conversion of I URIN I ammonia to urea is severely impaired, leading to elevated levels II of ammonia. 2. Congenital: Genetic deficiencies of each of the five enzymes of the urea cycle (and of NAGS, have been described, with an t ::- overall incidence of -1 :25,000 live births. X-linked OTC deficiency is lhe most common of these disorders, predominantly affecting ~ k: : = males, although female carriers may become symptomatic. All :t.! I{ le h Amino llCldll of the other urea cycle disorders follow an autosomal-recessive Qlut miM ~ inheritance pattern. In each case, the failure to synthesize urea Qlutamine ~ leads to hyperammonemia during the first weeks following birth. Qlutamlne ~Glutamate [Note: The hyperammonemia seen with arginase deficiency is less severe because arginine contains two waste nitrogens and Qlutamlne ····' NH3 NHtu.NHa can be excreted in the urine.] Historically, urea cycle defects had NHa a high morbidity (neurologic manifestations) and mortality. Treatment included restriction of dietary protein in the presence of sufficient calories to prevent protein catabolism. Administration of com- Figure 19.20 pounds that bind covalently to nonessential amino acids, produc- Treatment of patients with urea ing nitrogen~ontaining molecules that are excreted in the urine, cycle defects by administration of has improved survival. For example, phenylbutyrate given orally is phenylbutyrate to aid in excretion of ammonia (NH3). converted to phenylacetate. This condenses with glutamine to form phenylacetylglutamine, which is excreted {Fig. 19.20). VII. CHAPTER SUMMARY Nitrogen enters the body in a variety of compounds present in food, the most important being amino acids contained in dietary protein. Nitrogen leaves the body as urea, ammonia, and other products derived from amino acid metabolism (Fig. 19.21}. Free amino acids in the body are produced by hydrolysis of dietary protein by proteases activated from their zymogen form in the stomach and intestine, degradation of tissue proteins, and di now synthesis. This amino acid pool is consumed in the synthesis of body protein, metabolized for energy, or its members used as precursors for other nitrogen-containing compounds. Free amino acids from digestion are taken up by intestinal enterocytea via sodium-dependent secondary active transport. Small peptides are taken up via proton-llnked tran1port. Note that body protein is simultaneously degraded and resynthesized, a process known as protein tumovar. The concentration of a cellular protein may be determined by regulation of its synthesis or degradation. The ATP-dependent, cytosolic, selective ublquHln-proteasome and ATP-independent, relatively nonselective lysosomal acid hydtolllBflll are the two major enzyme systems that are responsible for degrading proteins. Nitrogen cannot be stored, and amino acids in excess of the blosynthetlc needs of the cell are quickly degraded. The first phase of catabollsm Involves the transfer of the a-amino groups through tranaamlnatlon by pyrldoxal phosphal&-dependent amlnotransfenlllBB (transamlnans), followed by oxidative deamlnallon of glutamate by glutamate dehydrogenue, forming ammonia and the corresponding u-kelo acids. A portion of the free ammonia is excreted in the urine. Some ammonia is used in converting glutamate to glutamlne for safe transport, but most is used in the hepatic synthesis of urea, which is quantitatively the most important route for disposing of nib'ogen from the body. Alanine also earlies nitrogen to the liver for disposal as urea. The two major causes of hyperammonemia (with its neurologic effects) are acquired liver disease and congenital deficiencies of urea cycle enzymes such as X-linked omithine transcarbamylaee. VII. Chapter Summary 259.______A_m_ln_o_a_c_ld_p_oo_1_ _ _ __,)l~ _____R_e_m_ova __l_of_n_lt_ro_ge_n_f_ro_m_a_m_l_n_o_a_c_ld_ _ _ _____, I I is defined as occurs bscause All of the free amino acids in 1----.. cells and extraoelluar fluids Amino acids cannot dlreclly putlelpate In energy metabollsm _____are_~produced---..- _ _by~-~tutJ contJumed by I until Degradation Synlhael of Degradation [ Amino groufs are removed J of body nones&11ntlal of dietary mediBtfJd by prol81n amino acid protein [ Two -.ue;ua1 reactions a-Keto acids and nitrogen ~---- souroe Proteolytic enzymes o1 the el tract and ~ncreas first t ] =~S::, >- [ Llverdamage t I ] due to Amino graupe transferred Hepatitis is~tsdby CllThoala to a-keto acldll forming: Heptotoxic drugs Ubiquitin Aapartate Cllublma:te Defects in enzymes N-Terminal of !tie urea cycle +. amino acids fo/Jowed by PEST sequences EJ dehydtogenw) results In Proteasome Lysosoine Cytoaol via Glutamate oxidatively nonspecific daamlna:llld to: proteases Simultaneous Clill1 release ammonia synltleele and leads to ----.{ Prol81n turnover] enters degradation { - - ----1[ Urea cycle ] 1 ~ I may have '9SU/ts tn t Nitrogen of asparate, ~ and NHa Incorporated Into Amlnoaclda Metabollam used In of amino Urea blosynth8818 acld8 ~ted eh:=FJ ---- is by involves i ~---~ ~ cha~by I "9atedby 0..- - 20 - TranecrlpUon and translation factors Blosynthetlc pathways t Hyperammonemia Mori>ldlty and Drug therapy ReducUon of 21 32 mortality protein intake 'M'7 - ;·.. ~-----------~~ y= Iii_ - Figure 19.21 Key concept map for nitrogen metabolism. GI"' gastrointestinal; PEST"' praline, glutamate, serine, threonine; NHa "' ammonia; 002 "' carbon dioxide. 260 19. Amino Acids: Nitrogen Disposal Study Questions Choose the ONE bast answer. Correct answer"' B. Transamination reactions always have 19.1 In this transamination reaction Oxaloacetate x an amino acid and an a-keto acid as substrates. The prod- ucts of the reaction are also an amino acid (corresponding (right), which of the following ~ to the o-keto substrate} end an a-kelo acid (corresponding to are the products X andY? ~ the amino acid substrate}. Three amino acid a-keto acid pairs commonly encountered in metabolism are alaninafpyruvate, A. Alanine, a-ketoglutarate Glutamate y aspertata/oxaloacetata, and glutamate/u-ketoglutarate. In this e. Aspartate, a-ketoglutarate question, glutamate is deaminated to form CJ-ketcglutarate, C. Glutamate, alanine and oxaloacelal8 is aminaled to form aspartate. D. Pyruvate, aspartate 19.2 Which one of the following statements about amino Correct answer= D. Glutamine, produced by the catabolism acids and their metabolism is correct? of branched-chain amino acids in muscle, is deaminated A. Free amino acids are taken into the enterocytes by by glutaminase to ammonia + glutamate. The glutamate is a single proton-linked transport system. deaminated by glutamate dehydrogenase to ammonia + B. In healthy, well-fed individuals, the input to the amino 11-ketoglutarate, which can be used for gluconeogenesis. acid pool exceeds the output. Free amino acids are taken into enterocytes by several dif- ferent sodium-linked transport systems. HeaHhy, wall-fed C. lhe liver uses ammonia to buffer protons. individuals are in nitrogen balance, in which nitrogen input D. Muscle-derived glutamine is metabolized in liver equals output. The liver converts ammonia to urea, and the and kidney tissue to ammonia+ a gluconeogenic kidneys use ammonia to buffer protons. Amino acid catabo· precursor. lism begins with transamination thet generates glutamate. E. The first step in the catabolism of most amino acids The glutamate undergoes oxidative deamination. Toxic is their oxidative deamination. ammonia is transported as glutamine and alanine. Arginine is synthesized and hydrolyzed in the hepatic urea cycle. F. The toxic ammonia generated from the amide nitrogen of amino acids is transported through blood as arginine. For Questions 19.3-19.5, use 1he following scenario. A female neonate appeared healthy until age -24 hours, when she became lethargic. A sepsis workup proved negative. At 56 hours, she started showing focal seizure Correct answer = C. Genetic deficiencies of each of lhe activity. The plasma ammonia level was found to be five enzymes of the urea cycle, as well as deficiencies in N-acetylglutamate synthase, have been described. The 887 µmol/I (normal 5-35 µmol/I). Quantitative plasma accumulation of citrulline (but not argininosuccinate) in lhe amino acid levels revealed a marked elevation of citrulline plasma of this patient means that lhe enzyme required for but not argininosuccinate. the conversion of citrulline to argininosuccinate (arginino- succinate synthetase} is defective, whereas the enzyme 19.3 Which one of the following enzymic activities is most that cleaves argininosuccinate (argininosuccinate lyase) is likely to be deficient in this patient? functional. A. Arglnase B. Argininosuccinate lyase Correct answer = B. Deficiencies of the enzymes of the c. Argininosuccinate synthetase urea cycle resuH in the failure to synthesize urea and D. Carbamoyl phosphate synthetase I lead to hyperammonemia in the first few weeks after birth. E. Omithine transcarbamylase Glutamine will also be elevated because it acts as a nontoxic storage and transpon form of ammonia. Therefore, elevated 19.4 Which one of the following would also be elevated in the glutamlne accompanies hyperammonemla. Asparaglne blood of this patient? and lysine do not serve this sequestering role. Urea would A. Asparaglne be decreased because of Impaired activity ol lhe urea cycle. [Note: Alanine would also be elevated In this patient.] B. Glutamine c. Lysine D. Urea The arginine will be cleaved by arginese to urea and omi- 19.5 Why might supplementation with arginine be of benefit thine. Omilhine will be combined with carbemoyl phosphate by omithine transcarbamylase to form citrulline. Citrulline, to this patient? containing one waste nitrogen, will be excreted.