Introductory Chemistry: Electrons and Periodic Table PDF
Document Details
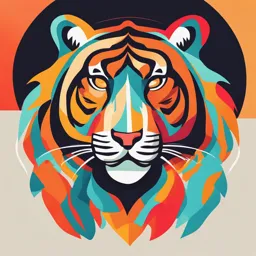
Uploaded by FirstRateMercury
Tags
Related
- Atomic Structure - Chemistry PDF Notes
- General, Organic, and Biochemistry Textbook - Chapter 2: The Structure of the Atom and the Periodic Table PDF
- HSPS Unit 3 Atomic Structure, The Periodic Table and Radioactivity PDF
- Atomic Structure PDF
- Yr 10C Atomic Structure Core Notes PDF
- Chapter 1 Lesson 3: Periodic Table of Elements PDF
Summary
This document is a chapter from a textbook on introductory chemistry. It details electrons and the periodic table, including topics like the nature of light, the Bohr atom model, atomic orbitals, electron configurations, and periodic trends. It also includes sample problem illustrations.
Full Transcript
Because learning changes everything.® Introductory Chemistry: An Atoms First Approach, 3e Chapter 2 Electrons and the Periodic Table © McGraw Hill LLC. All rights reserved. No reproduction or distr...
Because learning changes everything.® Introductory Chemistry: An Atoms First Approach, 3e Chapter 2 Electrons and the Periodic Table © McGraw Hill LLC. All rights reserved. No reproduction or distribution without the prior written consent of McGraw Hill LLC. Chapter 2—Electrons and the Periodic Table 2.1 The Nature of Light. 2.2 The Bohr Atom. 2.3 Atomic Orbitals. 2.4 Electron Configurations. 2.5 Electron Configurations and the Periodic Table. 2.6 Periodic Trends. 2.7 Ions: The Loss and Gain of Electrons. © McGraw Hill LLC 2 2.1 The Nature of Light—Wavelength and Frequency Wavelength (λ) is the distance between identical points on successive waves. Frequency (ν) is the number of waves that pass through a particular point in one second. As wavelength increases, energy decreases. As frequency increases, energy increases. Access the text alternative for slide images. © McGraw Hill LLC 3 2.1 Nature of Light—Emission Spectrum The electromagnetic spectrum arranges all types of electromagnetic radiation (light). The shortest wavelengths are gamma rays, and the longest are radio waves. Our familiar visible spectrum occupies only a very small portion of the overall electromagnetic spectrum. Access the text alternative for slide images. © McGraw Hill LLC (a) Don Farrall/Photodisc/Getty Images; (b) Ron Worobec/500px Prime/Getty Images; (c) Dorling Kindersley/Getty Images 4 2.1 The Nature of Light—The Electromagnetic Spectrum Access the text alternative for slide images. © McGraw Hill LLC (hand x-ray) itsmejust/Shutterstock; (head MRI) Don Farrall/Photodisc/Getty Images 5 2.1 The Nature of Light—Elemental Line Spectra Emission spectra that are not continuous, but contain only discrete lines, are called line spectra. Line spectra turned out to be critical to our development of models of the atom. Access the text alternative for slide images. © McGraw Hill LLC (a–d) H.S. Photos/Alamy Stock Photo 6 Hydrogen Helium Spectrum Spectrum 7 © McGraw Hill LLC 2.2 The Bohr Atom—Quantized Light Tiny, discrete “packets” of light are known as photons. Danish physicist Niels Bohr utilized this notion of photons combined with the line spectrum of hydrogen to conceive a model that we now refer to as the Bohr atom. © McGraw Hill LLC Library of Congress Prints & Photographs Division [LC-DIG-ggbain-35303] 8 2.2 The Bohr Atom—The Bohr Model He called the series of concentric, circular paths around the nucleus orbits. Each orbit was at a specific energy level, which was designated by a quantum number, n. The electron in the hydrogen atom resided at the lowest possible energy level (n = 1), called the ground state. Access the text alternative for slide images. © McGraw Hill LLC 9 © 2015 Pearson Education, Inc. Electron energy is quantized When a hydrogen atom absorbs energy, an electron is excited to a higher-energy orbit. The electron then relaxes back to a lower- energy orbit, emitting a photon of light. © McGraw Hill LLC 10 © 2015 Pearson Education, Inc. Hydrogen Emission Lines © McGraw Hill LLC 11 Described the electron transitions responsible for the line spectrum of hydrogen. Failed to the line spectra of any other elements. When an atom has more than one electron, the model has to be more complex. © McGraw Hill LLC 12 2.3 Atomic Orbitals—Quantum Mechanical Model The failure of the Bohr model was followed by the quantum mechanical (QM) model. The QM model does not specify the location of an electron. The QM model defines a region of space called an orbital. Electrons are most likely to be found in the QM orbital. Unlike Bohr’s orbits, which were all circular and varied only in size, QM orbitals vary in shape. © McGraw Hill LLC 13 2.3 Atomic Orbitals—Electron Density Map QM orbitals are the region an electron is most likely to be found. A sparkler is a good visual for this. We can estimate ~90% of the sparks are contained within a spherical region with a radius of five centimeters. Unlike the sparks, we cannot know or map the coordinates of electrons in an atom. The use of QM enables us to calculate the probability of finding an electron. It allows us to construct a probability density map of possible locations for an electron. Such a probability map of electron density within an atom is called an atomic orbital. Access the text alternative for slide images. © McGraw Hill LLC (a–c) Imagemore/Getty Images 14 2.3 Atomic Orbitals—Shells, Subshells, and Orbitals Principal energy levels (shells: n = 1, 2, 3 ) contain one or dot dot dot more sublevel. Energy sublevels (referred to as subshells: s, p, d, and f), each contains one or more orbitals. The number of energy sublevels and the number of orbitals depend on the value of the principal quantum number (n), which is an integer. The number of energy sublevels in each principal energy level is equal to the principal quantum number, n. © McGraw Hill LLC 15 2.3 Atomic Orbitals—Orbital Designations the energy and the number of When n is sublevel letter orbitals in the designation can be energy sublevel is 1 s 1 2 s 1 p 3 3 s 1 p 3 d 5 4 s 1 p 3 d 5 f 7 © McGraw Hill LLC 16 2.3 Atomic Orbitals—s Orbitals The s-orbital is spherical. The probability of finding an electron (the electron density) is highest near the nucleus. Electron density decreases as we move away from the nucleus. Access the text alternative for slide images. © McGraw Hill LLC 17 2.3 Atomic Orbitals—s Orbital Model The 2s orbital looks very much like the 1s orbital, just bigger. The same is true for the 3s and 4s orbitals. Access the text alternative for slide images. © McGraw Hill LLC 18 2.3 Atomic Orbitals—p Orbitals Beginning with the second principal energy level (n = 2), in addition to the s sublevel, there is also a p sublevel. A p sublevel contains three p orbitals that are shaped like dumbbells. Each of the p orbitals lies along one of the axes, making them all perpendicular to one another. Access the text alternative for slide images. © McGraw Hill LLC 19 2.3 Atomic Orbitals—d and f Orbitals Beginning with the third principal energy level (n = 3), there is also a d sublevel containing five d orbitals. Beginning with the fourth principal energy level (n = 4), there is an f sublevel, which contains seven f orbitals. The shapes of the f orbitals are highly complex. Access the text alternative for slide images. © McGraw Hill LLC 20 2.3 Atomic Orbitals—Hydrogen Orbital Energy Levels Access the text alternative for slide images. © McGraw Hill LLC 21 2.3 Atomic Orbitals—Multi-electron Orbital Energy Levels Access the text alternative for slide images. © McGraw Hill LLC 22 Sample Problem 2.2 Consider each of the following sublevel designations. Determine if each is a legitimate designation and if not, explain why: (a) 1p, (b) 2s, (c) 3f, (d) 4p © McGraw Hill LLC 23 2.4 Electron Configurations— Electron Configuration Overview The behavior of an atom depends on its electron configuration. This is the specific arrangement of electrons in the atom’s orbitals. The electron configuration is a list of numbers and letters indicating which energy sublevels are occupied. The number of electrons in the sublevel is indicated with a superscript. © McGraw Hill LLC 24 2.4 Electron Configurations—Orbital Diagrams Overview An orbital diagram is similar to the electron configuration. Each orbital is represented with a square box. Each orbital is labeled with the appropriate n value and letter designation. Each electron is represented with an arrow. © McGraw Hill LLC 25 2.4 Electron Configurations—Spin Imagine an electron spinning on its vertical axis like a top. There are two possible directions for it to spin. Two electrons that are spinning in opposite directions are said to have paired spins. When we represent two electrons in the same orbital diagram, we orient the arrows in opposite directions. The first arrow typically is pointed up, so the second arrow must point down. Access the text alternative for slide images. © McGraw Hill LLC 26 2.4 Electron Configurations— General Rules 1. Electrons will occupy the lowest energy orbital available. 2. An orbital can accommodate a maximum of two electrons. 3. Any two electrons occupying the same orbital must have electrons spinning in opposite directions. (This is known as the Pauli exclusion principle.) 4. Orbitals of equal energy (those in the same energy sublevel) will each get one electron before any of them gets a second electron. (This is known as Hund’s rule.) © McGraw Hill LLC 27 2.4 Electron Configurations—He and Li Examples Access the text alternative for slide images. © McGraw Hill LLC 28 2.4 Electron Configurations—C and O Examples Access the text alternative for slide images. © McGraw Hill LLC 29 2.4 Electron Configurations— Shorthand Notation We can abbreviate part of an electron configuration. The noble gas core can be used for a more compact electron configuration. For example, consider a sodium atom. We can write the electron configuration as 1s 2 2 s 2 2 p 6 3s1. Since 1s 2 2 s 2 2 p 6 is actually the configuration of neon, we could abbreviate sodium’s configuration as [ Ne ] 3s1. Access the text alternative for slide images. © McGraw Hill LLC 30 Sample Problem 2.3—Problem and Strategy Determine both the full and condensed ground-state electron configurations and orbital diagram for each of the following elements. (a) Mg, (b) Al, and (c) S. © McGraw Hill LLC 31 2.5 Electron Configurations and the Periodic Table—Model Access the text alternative for slide images. © McGraw Hill LLC 32 Sample Problem 2.4—Problem and Strategy Write the ground-state electron configuration, using the noble gas core abbreviation, for each of the following elements: (a) Ca, (b) Te, and (c) Br. © McGraw Hill LLC 33 2.5 Electron Configurations and the Periodic Table—Valence Electrons The outermost electrons in an atom, those with the highest principal quantum number, are called the valence electrons. The inner electrons in an atom are called the core electrons. Valence electrons give elements their “personalities.” Within a group, the valence electron configurations are the same. Access the text alternative for slide images. © McGraw Hill LLC 34 Sample Problem 2.5—Problem and Strategy Identify the valence electrons in the electron configurations that you wrote for each element in Sample Problem 2.4. © McGraw Hill LLC 35 2.5 Electron Configurations and the Periodic Table—Lewis Dot Symbols In Lewis dot symbols, valence electrons are shown as dots around the element’s symbol. The maximum number of dots around an element’s symbol is eight (total of two electrons in the s orbital and six electrons in the three p orbitals). When we write Lewis dot symbols, we don’t put a second dot in any position (left, right, top, or bottom) until there are single dots in all the positions. © McGraw Hill LLC 36 2.5 Electron Configurations and the Periodic Table—Lewis Dot Symbol Examples Access the text alternative for slide images. © McGraw Hill LLC 37 2.6 Periodic Trends—Atomic Size Access the text alternative for slide images. © McGraw Hill LLC 38 Sample Problem 2.6—Problem and Strategy For each pair of elements, indicate which one you would expect to be the largest: (a) Al or P, (b) Se or O. © McGraw Hill LLC 39 2.6 Periodic Trends—Metallic Character Access the text alternative for slide images. © McGraw Hill LLC 40 2.6 Periodic Trends—Ionization Energy The energy required to remove an electron from an atom is called the ionization energy. As we move down a group, less and less energy is required. As we move from left to right across a period, the amount of energy required to remove an electron generally increases. As we move down a group, atomic size increases and the valence electrons are farther and farther away from the nucleus—making the attractive force between them weaker, and making an electron easier to remove. As we move from left to right, not only does the magnitude of charge on the nucleus increase, but also the distance between it and the electron to be removed (because atomic size gets smaller) decreases. © McGraw Hill LLC 41 2.6 Periodic Trends—Ionization Energy by Element Access the text alternative for slide images. © McGraw Hill LLC 42 Sample Problem 2.7—Problem and Strategy For each pair of elements, indicate which one you would expect to have the least metallic character: (a) Na or Cl, (b) I or F, (c) Li or Rb. © McGraw Hill LLC 43 Sample Problem 2.8—Problem and Strategy For each pair of elements, indicate which one you would expect to be more difficult from which to remove an electron. (a) Rb or Li, (b) Ca or Br. © McGraw Hill LLC 44 2.6 Periodic Trends—Electron Affinity The ability of an atom to gain an electron is its electron affinity. In general, an atom that loses an electron easily will not gain an electron easily. An atom that does not lose an electron easily will readily gain an electron. This leads to essentially opposite trends for the two processes from left to right within a period. Atoms at the far right of the periodic table (not including the noble gases) gain electrons most easily. Access the text alternative for slide images. © McGraw Hill LLC 45 2.7 Ions: The Loss and Gain of Electrons—Cations and Anions When an atom loses or gains one or more electrons, it becomes an ion, specifically, an atomic ion, which is simply an atom in which the number of electrons is not equal to the number of protons. Because an electron has a negative charge, the loss or gain of electrons causes an atom to become charged. An atom that loses one or more electrons becomes positively charged, and we refer to it as a cation. An atom that gains one or more electrons becomes negatively charged, and we refer to it as an anion. © McGraw Hill LLC 46 2.7 Ions: The Loss and Gain of Electrons—Isoelectronic Atoms of the main-group elements will lose or gain electrons to achieve a noble gas electron configuration. This is referred to as isoelectronic with a noble gas. This phenomenon enables us to predict the number of electrons that will be lost or gained in each case—allowing the prediction of the ion charge. Ca [ Ar ] 4 s 2 Becomes Ca 2+ [ Ar ] © McGraw Hill LLC 47 2.7 Ions: The Loss and Gain of Electrons—Common Ions Access the text alternative for slide images. © McGraw Hill LLC 48 Sample Problem 2.10—Problem and Strategy Predict the charge on the common ion formed from each element listed and write the electron configuration for the ion. (a) Na (b) Ca (c) O © McGraw Hill LLC 49 2.7 Ions: The Loss and Gain of Electrons—Lewis Dot Symbols of Ions We can represent ions of main-group elements with Lewis dot symbols. To do this, we start with the Lewis dot symbol of an atom and remove a dot for each electron it loses—or add a dot for each electron it gains. © McGraw Hill LLC 50 Sample Problem 2.11—Problem and Strategy Write the Lewis dot symbols for the atom and the ion commonly formed by each element. (a) P (b) Se (c) Sr © McGraw Hill LLC 51 Sample Problem 2.11—Solution Solution Access the text alternative for slide images. © McGraw Hill LLC 52