Lectures 4-5 PDF
Document Details
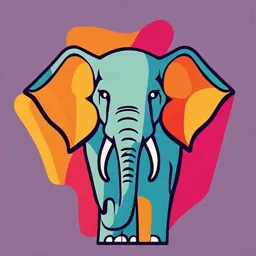
Uploaded by AltruisticSilicon
Tags
Summary
These lecture notes provide an overview of saccades, rapid eye movements. They discuss different types of saccades, factors affecting latency, and the processes involved in saccade adaptation. The notes also cover the neuroanatomy and control of saccades.
Full Transcript
Saccades are fast conjugate movements that move both eyes quickly in the same direction Not only toward visual objects, but also toward auditory and tactile stimuli, as well as toward memorized objects Saccades are among the fastest movements that the body can make* We make more th...
Saccades are fast conjugate movements that move both eyes quickly in the same direction Not only toward visual objects, but also toward auditory and tactile stimuli, as well as toward memorized objects Saccades are among the fastest movements that the body can make* We make more than 100,000 saccades per day Types of saccades Volitional ○ Visually guided Saccades to a visual target ○ Memory guided Saccade to a target that is no longer visible Requires keeping the location of the target in working memory ○ Express saccades Saccades of ultra-short latency (~80-100 msec) Bypasses cortical processing ○ Antisaccade Subject must make saccade in opposite direction of visual target Used as a probe for frontal lobe function ○ Saccade sequences Subject must encode several saccades together Involuntary ○ Reflexive saccades Saccades made in response to sudden appearance of a novel stimulus (visual, auditory or tactile) ○ Quick phases of nystagmus Function: to reset eye position following the slow phase of nystagmus Generation: uses same neural circuitry as other saccades ○ Microsaccades During fixation, eyes are not still but often exhibit microsaccadic movements Small- typically ~ 12’ Several times a second during fixation May help prevent perceptual fading Driven by similar neural circuitry as larger saccades Vision is altered during a saccade preventing blur of images as eye moves 2 mechanisms ○ Saccadic suppression Altered sensitivity; brain selectively blocks visual so that neither motion of eye nor the gap in visual perception is noticeable Detection thresholds are elevated during saccades by up to 0.5 log unit It begins some 20 msec before the onset of a saccade and extends to 35 msec after completion of a saccade ○ Masking AKA saccadic omission (high VA before and after a saccade masks the period of low acuity during a saccade) Relates to visual masking phenomenon (forward and backward masking paradigm) Has the strongest effect Saccade latency Latency is the time taken between the appearance of a target and the eye movement to the target Average saccade latency is ~200 msec; slightly faster in a monkey Cortical and sub- cortical processing related to visual processing, target selection and motor programming contributes to latency Under certain conditions, saccades can be of ultra-short latency (~80 msec) and these are express saccades ○ When a fixation point is removed 200 msec prior to target onset (the gap condition) ○ Humans can make stimulus-controlled saccades that are initiated very rapidly (80-120 msec) Effects of blinks on saccades: Dynamic overshoots (DO) occur more frequently with blinks Peak velocity are smaller for similar size saccades when the subject blinks during saccades Skewness Skewness of a saccade defines the asymmetry of the trajectory Determined as (=time to reach peak velocity/total duration of saccade) ○ Time to reach peak velocity ○ Total duration of saccade ○ For small saccades, skewness is ~0.5 ○ For large saccades, ~0.2 Increases for anti-saccades, saccades made to remember target, in fatigue or decreased vigilance ○ This is because the premotor saccadic eye movement commands are different for different saccades Promptness Of a saccade is the reciprocal of saccadic latency ○ 1/(latency) The variability in saccade initiation time reflects the decision-making time plus neurological control processes Saccade adaptation Central conjugate and disconjugate adaptation of saccades is necessary to compensate for changes in the visual consequences of saccadic eye movements due to aging, disease, spectacle correction, etc Ex: an abducens nerve palsy can lead to apparent weakness of a single lateral rectus muscle. Patching the normal eye for a few days results in central adaptation within the saccadic system wherein innervation to the paretic lateral rectus is increased. The cerebellum is important for saccade adaptation Saccadic control Higher level: neuroanatomic sites (supranuclear) ○ Frontal Eye Fields (FEF) Most important for the generation of vertical and horizontal saccades Efferent projections from frontal eye fields go to: They receive sensory information from the parieto-occipital temporal region (POT) and determine the occurrence of a future saccade, and computes direction and size of the saccade Lesions of the frontal eye fields result in increased saccadic latencies, slowed saccades, impaired predictive tracking These contain a neural map of visual space thus provide the initial information on size and direction of intended saccade. Attention and selection Send spatial localization signal (amplitude and direction) to the superior colliculus Inhibits abnormal fixation reflexes ○ Parietal lobes Sends information related to localizing targets of interest plus computations of saccade amplitude and direction to the superior colliculus Directs visual attention to objects in the extra personal space They receive input signals from medial superior temporal (MST), supplemental eye fields, frontal eye fields, and striate cortex They project onto the dorso-lateral prefrontal cortex ○ Basal ganglia The substantia negra pars reticulata (SNpr) and the caudate nuclei are the nondopaminergic portions of the basal ganglia They control saccade-related neuron in the superior colliculus They gate selectively, reflexive and voluntary saccades generated by the superior colliculus They facilitate the initiation of more voluntary, self-initiated forms of saccades- tasks involving prediction and memory Aid steady fixation by preventing unwanted reflexive saccades Muscimol (GABA agonist) Bicuccline (antagonist to GABA) ○ Superior colliculus The superficial-3 layers of the superior colliculus are sensory cells with retinotopic organization and receptive fields. Cells in the deeper layers of the superior colliculus are motor and have efferent projection to brainstem premotor neurons. They help determine direction, speed and accuracy of saccades for targets in the peripheral visual field. Ablation of superior colliculus reduces the number of spontaneous saccades Processes signals related to an intended saccade received from frontal eye fields, parietal lobes, and caudate nuclei, encodes it for the desired eye position change with respect to the fovea, and transmits it to the brainstem ○ Cerebellum Concerned with Saccadic Gain-Control or adaptive processes Receives input from brainstem structures involved in saccade generation: nucleus prepositus hypoglossi (NPH), nucleus reticularis tegmenti pontis (NRTP), paramedian pontine reticular formation (PPRF), Rostral interstitial nucleus of medial longitudinal fasciculus (riMLF), and medial vestibular nucleus (MVN) Outputs to brainstem and other saccade-related sites to maintain, and/or adapt saccade gain Controls accuracy of secondary saccades Lesions of the cerebellum flocculus and vermis result in saccadic dysmetria (hypermetria). Defect is ipsilateral in hemi-cerebellectomy Total cerebellectomy produces persistent saccadic dystemia (especially hypermetria) Lower level: neuroanatomic sites (nuclear)- brainstem ○ Network of neurons in brainstem control saccade metrics (velocity, amplitude, duration) 1. Excitatory Burst Neurons (EBN) in the paramedian pontine reticular formation drive horizontal saccades 2. Excitatory Burst Neurons in rostral interstitial medial longitudinal fasciculus (riMLF) drive vertical saccades ○ Lesions on the corresponding areas will stop saccades in a particular direction ○ Paramedian pontine reticular formation (PPRF) at the pons contains the burst neurons for horizontal saccades ○ Rostral interstitial nucleus of the median longitudinal fasciculus (riMLF) contain the burst cells for vertical saccades. ○ Types of Neurons: Short- Lead (Excitatory Burst Neurons) Long- Lead (Excitatory Burst Neurons) Omnipause Neurons Neural integrator The neural velocity to position integrators transform the saccade related signal of the burst generators into an eye position related tonic signal they convey to motoneurons. They are largely cofined to three heavily interconnected midbrain structures: 1) The interstitial nucleus of Cajal (NIC), 2) The nucleus prepositus hypoglossi (NPH), 3) The vestibular nuclei (VN) Integration in the horizontal and vertical planes is accomplished largely independently by the NPH-VN and the NIC-VN complexes, respectively. Cells in these regions carry a more or less intense phasic Adaptive Role of the Cerebellum The cerebellum provides a positive feedback loop with a gain of K that improves the time constant of an inherently leaky brainstem neural integrator Posterior Commissure Lesions of the posterior commissure produce abnormalities upward gaze Possibly accounts for the dorsal-midbrain syndrom (Parinaud’s Syndrome) characterized by ○ Impairment of upwardly directed saccades - to - loss of vertical movements of the eyeball ○ Convergence retraction ○ Pupillary mydriasis ○ Corectopia ○ Light-near dissociation Paramedian pontine reticular formation (PPRF) Primary center responsible for generating horizontal conjugate gaze 4 types of cells: ○ 1. Excitatory burst cells which generate horizontal saccades that are ipsilateral to the N.VI. These discharge only when the need for a saccade arises. There are the long-lead burst neurons (LLBN) and short-lead excitatory burst neuron ○ 2. Pause cell Localized in the nucleus raphe interpositus at the rootlets of the abducens nuclei. These maintain tonic discharges. They discharge continuously (tonic) except immediately prior to, during saccades, and blinks They receive information from higher level centers (Frontal Eye Fields/Suplementary eye field, Superior Colliculus, and long lead burst neurons) that a saccadic response is intended. The role of the pause cells is to tonically inhibit burst cells thereby prevent unwanted saccades during fixation They inhibit the burst neurons within the same paramedian pontine reticular formation They help synchronize the activity of the premotor burst neurons Dysfunctional pause cells results in opsoclonus ○ 3. Inhibitory burst cells These act to inhibit the antagonist muscles of the intended eye movement Their discharge rates are inversely proportional to those of the excitatory burst cells (EBN) ○ Tonic cells These discharge in relation to eye position which increases proportionately with eccentric gaze position Saccadic control: Horizontal Frontal eye field sends information to the contralateral pons (where CN VI and the paramedian pontine reticular formation are) CN VI sends the signal to ipsilateral lateral rectus to contract and communicates with the contralateral CN III through the medial longitudinal fasciculus. That CN III activates its ipsilateral medial rectus. Example. An object of interest appears in the lea visual field. The right frontal eye field finds it and sends a signal to the lea CN VI. The lea CN VI activates the lateral rectus of the lea eye and sends a signal to the right CN III. The right CN III activates the right medial rectus (so it contracts). Saccadic control: Vertical Frontal eye field sends information to the midbrain (where CN III and CN IV are). These two nerves together control the vertical recti and the obliques CN III and CN IV are in the dorsal midbrain at the level of the superior and inferior colliculus respectively. They activate the corresponding muscles. The vestibular system can also move the eyes without interaction from the FEF A patient that moves their eyes to compensate for a movement of the head but can not complete a voluntary saccade will have a problem with… Higher or lower cortical areas of control? The supranuclear centers of control for saccades Anomalies with saccade initiation Delayed initiation- abnormally long latency (>250 msec) ○ Allowances must be made for the age of patient, state of consciousness and level of attention A defect of saccade initiation resulting in several seconds of reaction time ○ Focal lesions especially of the FEF ○ Patients (Huntington’s disease) have normal amplitude of random saccade and frequency of nystagmus but characteristically abnormal voluntary saccade initiation Anomalies with saccade size Dysmetric saccades with associated corrective saccades ○ Static overshoot ○ Static undershoot Attributed to probable gain fluctuations and biases in the cerebellum Static overshoot is a high gain saccade seen in cerebellar disease, not frequent in normals Dysmetria Hypermetric saccades are made also by patients with VF defects (hemianopia) ○ In these patients are present pulse-less step corrective movements (or postsaccadic drifts) known as glissades Anomalies with saccade velocity size Slowed dynamics ○ Peak velocity 2-std below mean value “increased saccadic duration ○ A saccade is too fast or too slow when its peak velocities fall outside the main sequence (amplitude-peak velocity relationship) ○ Slow saccades of restricted amplitude reflect abnormalities of the ocular motor periphery Example: lesions of the MLF (internuclear ophthalmoplegia) or ocular muscle paresis ○ They are a sign possibly pointing to: Introrbital tumors and contusions Graves syndrome, hyperthyroidism Myasthenia gravis Refixation saccades Normometric (orthometric) ○ Fits the main sequence ○ Low velocity Dysmetric ○ Single sweep saccades Hypometric ○ Pulseless ○ Glissadic undershoots Hypermetric ○ Glissadic overshoots ○ Dynamic overshoots Clinical examination of saccades 1. Check if quick phases are affected by the disease 2. Check for patient’s ability to made reflexive saccade 3. Determine if patient can perform saccades in the absence of a visual task a. While using a frenzel goggle b. Make saccades with lids closed 4. Check if patient is able to make predictive saccades PURSUITS Pursuits eye movement: allow the eyes to closely follow a moving object Pursuit is modified by ongoing visual feedback Pursuing a target needs catch up saccades if moving target velocity is greater than 30 degrees/sec Goal of smooth-pursuit is to stabilize the image of a slowly moving small object on the fovea Stabilizing the image results in the minimization of retinal slip and therefore improves visibility Functions of smooth pursuits 1. Stabilizes the image of a small moving target on the fovea 2. Cancels the VOR during combined eye-head tracking a. During smooth tracking of a target that moves in the same direction as the head, smooth pursuit cancels vestibulo-ocular reflex, otherwise, the VOR would move the eyes opposite the direction of intended gaze 3. Cancels optokinetic nystagmus during tracking of a small, moving target against a detailed stationary background a. During smooth tracking of a bird flying against a background of leaves, the optokinetic system will try to hold the gaze on the stationary background, but it is overridden by pursuit Smooth-Pursuit: Tracking a smoothly moving object Generated in response to retinal image motion Match eye velocity to target velocity; target velocities can be up to 90 deg/sec Latency about 130 msec (compare this to VOR and saccades) Accuracy of SP is determined by its gain; smooth pursuit gain -> eye velocity/target velocity Smooth pursuit is not a reflexive eye movement. Unlike vestibular ocular reflex and optokinetic nystagmus Smooth pursuit is mostly a closed loop response Aging leads to lower gain and more frequent catch-up saccades Factors influencing the smooth-pursuit response Size ○ Larger targets improve smooth pursuit response; central 5-10 deg dominates the smooth pursuit output Luminance ○ Brighter targets improve response; lower latency Contrast ○ Higher contrast is better than low contrast Saliency ○ Improving target saliency by color or increased luminance improves smooth-pursuit response (increased acceleration; decreased latency) ○ Presence of a distractor degrades the response Influence background ○ Smooth pursuit performance is degraded when the target is moving over a textured background that is in the same depth plane as the target ○ If the target moves in a plane closer than the background, then smooth pursuit performance is improved Pursuit drivers 1. Target velocity a. Most important goal is to match eye velocity to target velocity b. The step-ramp stimulus is evidence for the importance of target velocity 2. Target position a. If the eye is already tracking and the target jumps ahead a little bit, there is a position error but no additional velocity error b. In this case, the eye can accelerate to catch-up, suggesting that the pursuit system is responding to the position error c. If the position error is large, a catch-up saccade is executed 3. Efference copy a. When pursuit eye velocity approaches target velocity, retinal slip is close to zero b. The brain continues to generate pursuit eye movements and we continue to perceive target motion c. Such a system can be achieved if the brain uses efference copy (an internal copy of a motor innervation) of the eye movement to reconstruct the motion of the target in the brain d. The perceived or reconstructed target motion drives pursuit 4. Pursuit without image motion a. We can track the motion of our finger in darkness b. Proprioception information is used to reconstruct apparent motion of the target and drive pursuit c. We can track motion of sound; the moving sound stimulus can be reconstructed in the brain as a moving visual target that drives pursuit 5. Pursuit to stimuli that do not move a. Stroboscopic illumination can result in a perception of motion and pursuit eye movements b. Can generate pursuit eye movements when attempting to track the center of a rolling wheel Phases of the smooth-pursuit response Open loop period (first 40 ms of tracking response after latency period) ~initial acceleration does not depend on target motion parameters The next ~60-80 msec is also an open loop. However, the eye acceleration depends on the target velocity. Larger target velocities results in greater acceleration The period after that is the closed loop phase. Here the pursuit movement is influenced by visual feedback from the retina Ocular following Visual tracking reflex brought about by sudden step motion of a large-field stimulus Unlike smooth pursuit, ocular following latency is ~60 ms Stereotypic eye movement response While smooth pursuit helps keep the eye on a moving target, ocular following helps stabilize vision if a sudden motion of the visual world occurs NEUROANATOMIC CONTROL OF AND SIGNAL PROCESSING FOR PURSUIT EYE MOVEMENTS The primary neuroanatomic structures involved in the generation of pursuit eye movements along with the aspects most relevant to the clinician are: The primary striate visual cortex (V1) contains cells responding to stimulus motion, which project heavily to the middle temporal (MT) area of the extrastriate visual cortex. The MT area encodes and processes the direction and velocity of stimulus motion and then it projects to the adjacent media superiortemporal (MST) visual area The MST area probably encodes both visual signals related to pursuit and the efference copy of the eye movement command Both the MT and MST areas project to the posterioparietal cortex (PPC), which probably plays role in attentional aspects of target motion. The MT and MST areas and the PPC project to the frontal eye fields (FEFs, area 8), which contain neurons that fire during pursuit, perhaps especially during predictive movements. The MT and MST areas and FEFs project to the dorsolateral pontine nucleus (DLPN), which contains cells exhibiting direction selectivity, and they discharge in response to pursuit movements. The DLPN probably also receives the efference copy signal from the MST area The DLPN projects to the cerebellum (Gocculus, paraflocculus, and vermis). The Gocculus and paraflocculus contain Purkinje cells that discharge with respect to gaze velocity during pursuit; the vermal neurons encode target velocity in space (eye velocity plus retinal slip velocity). The cerebellum projects to the brainstem, especially to the medial vestibular nucleus (MVN), which discharges according to gaze velocity, and the nucleus prepositus hypoglossi (NPH). Both brainstem structures are probably involved in neural integration, which converts the eye velocity signals to eye position signals. Which in turn project to the oculomotor neurons to move the eye smoothly. Defects in visual pathways for pursuits movements Insult or disease anywhere along these pathways may cause a pursuit defect Lesions leading to smooth pursuit deficits Lesions in Middle Temporal Cortex (MT) produce Akinetopsia (motion blindness) in the contralateral visual field, so that saccades to fixed targets may be accurate but pursuit responses to moving targets presented in the affected field will be absent or deficient. Ipsilateral field may also be affected to a small degree due to overlap of representation for these areas. Lesions in Medial Superior Temporal Cortex (MST) produce visual ekects similar to MT, but also produce a unidirectional pursuit deficitfor targets in both visual hemifields moving toward the side of the lesion. Lesions in Posterior Parietal Cortex (PP) produce attentional deficits, which make pursuit of small targets more divcult than larger fields. Some patients show particular difficulty when the targets are moving toward contralateral hemispace Lesions in the frontal eye fields (FEF) produce a deficit for horizontal pursuit toward the side of the leson Lesions of the dorsolateral pontine nucleus (DLPN) produce a deficit for horizontal pursuit toward the side of the lesion Lesions of the cerebellum can abolish pursuit if they are extensive. More focal lesions in either the flocculus or vermis will reduce pursuit gain if the other is intact Abnormal pursuits classification Defects found in: ○ Initiation Latency Initial 100 msec open loop response Maximum eye acceleration ○ Gain ○ Saccadic overlay Examples of lesions that affect smooth pursuit A cerebellar infraction on the right side causes asymmetric impairment of pursuit. Tracking is smoother to the left than to the right Multiple sclerosis, and cerebellar ataxia causes jerky pursuits in both horizontal directions Cogwheel pursuit- cerebellar lesion Cerebellar and pontine lesions are frequent causes of smooth-pursuit dysfunction