Reactive Oxygen Species (ROS) and Oxidative Stress Lecture Notes PDF
Document Details
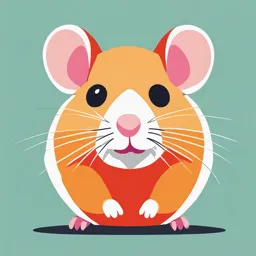
Uploaded by MonumentalPyrite
2024
Xin Jie Chen
Tags
Summary
These lecture notes detail Reactive Oxygen Species (ROS), covering their nature, sources, and the major defense mechanisms against oxidative damage. The document also outlines specific learning objectives related to ROS, emphasizing their chemical structures, generation mechanisms, and cellular effects. The notes focus on how the body manages ROS and the implications for human health and diseases.
Full Transcript
Reactive Oxygen Species (ROS) and Oxidative Stress 2024 Xin Jie Chen Department of Biochemistry & Molecular Biology General Learning Objec...
Reactive Oxygen Species (ROS) and Oxidative Stress 2024 Xin Jie Chen Department of Biochemistry & Molecular Biology General Learning Objectives: To know the nature and the sources of reactive oxygen species that cause oxidative damage and human diseases. To know the major defense mechanisms and antioxidants that protect cells from oxidative injuries. Specific Learning Objectives: To know the chemical structures of the five most important ROS. To know the reactions by which each of these five ROS is generated. To know the ways in which ROS can be interconverted and, in particular the role of free transition metal ions in generating highly reactive hydroxyl radicals. To know the major cellular damage caused by ROS. To understand the mechanism of ROS scavenging by cellular defense mechanisms and by dietary antioxidants. To understand the roles of reduced glutathione, thioredoxin, and peroxiredoxin in maintaining sulfhydryl-containing proteins in a functional state. To appreciate that strictly regulated generation of ROS at low levels mediates physiological functions (e.g., growth, differentiation, metabolism and anti-cancer) and cellular signaling. 1 Oxygen – a multifaceted story “Free radicals can kill you: Jacques‐Louis David: Portrait (1788) of Antoine‐Laurent Lavoisier (1743–1794) Lavoisier’s oxygen revolution” and his wife, Marie‐Ann (1758–1836). Gerald Weissmann, Editor‐in‐Chief, FASEB J. 24:649‐652, March 2010 Mme LAVOISIER: Imagine what it means to “ Experimental biologists understand what gives a leaf its color! What makes now hold oxidative stress a flame burn. Imagine! responsible for almost every aspect of development, diseases and bodily decline” 2 1. ROS, free radicals & oxidative stress O2 Oxygen 1e¯ ˙O2¯ Free Moderate Superoxide radical reactivity 1e¯ + 2H+ ROS H2O2 H Low (incomplete H Hydrogen Oxidant reactivity reduction of Peroxide oxygen) 1e¯ + 1H+ ˙OH H2O + Highest H Hydroxyl Free reactivity Radical radical 1e¯ + 1H+ Oxidative Oxidized ˙OH is an electron Stress Substrate H2O Substrate “stealer” Reactive oxygen species (ROS) is a collective term that describes chemically reactive molecular species formed upon incomplete reduction of oxygen. Those containing an unpaired electron are called free radicals. The free radicals we are most concerned with are oxygen free radicals such as superoxide and hydroxyl radical. Free radicals are generated by one-electron transfers. Molecular oxygen is reduced to superoxide (O2¯) by the one-electron reduction of molecular oxygen (O2). Superoxide, also known as the “primary ROS”, is a free radical as well as an oxidant (oxidizing agent). It participates in a redox reaction in which it is further reduced to hydrogen peroxide and hydroxyl radical - the “secondary ROS”. In hydroxyl radical, the unpaired electron is much more unstable compared with that in superoxide. This makes it highly reactive with a very short in vivo half-life of approximately 10-9 s. When produced, it reacts with cellular components close to its site of production by “stealing” an electron. This produces the stable water molecule with the biological substrates concomitantly oxidized. Over-oxidation of biological components causes oxidative stress in the cell. Hydrogen peroxide is not itself a free radical, but it is an oxidant and can generate other, more reactive oxidants such as hydroxyl radical. 3 2. Metabolism generates the primary ROS ‐ superoxide One‐electron transfer O2 + e- O - 2 4 Superoxide Production by Mitochondrial Respiratory Chain Transfer of paired Single electron electrons (2 e- / O atom) leak Parkinson’s disease MPTP Rotenone ---- +++++ Single electron leak 1 – 5% of total electrons leaked Adapted from Dröse and Brandt (2008) J. Biol. Chem. 283:21649-21654 In most cells, the production of superoxide occurs mainly (>90%) within mitochondria as a byproduct of ATP synthesis. During energy transduction in the electron transport chain, ~1- 5% of all electrons “leak” to oxygen prematurely to form superoxide. In complex III, semiquinone at the Qo site delivers one single electron to O2 to produce superoxide (O2-), which is injected into the intermembrane space. The complex I also generates superoxide. In a Complex I-catalyzed forward reaction, the reduced flavin group (and also the Q site) can deliver an electron to O2 to form superoxide. Superoxide produced from the complex I is exclusively released into the matrix. The membranes are not permeable to the anionic O2-. O2- production is increased under many pathophysiological conditions. Several xenobiotics interact with the mitochondrial electron transport chain and increase the rate of O2¯ production. Some of these compounds block electron transport, which increases the reduction level of electron carriers located upstream of the inhibition site. For example, MPTP (N- methyl-4-phenyl-1,2,3,6-tetrahydropyridine), an inducer of Parkinson’s disease, blocks electron flow to the Q site and increases electron leak in Complex I. The same happens to rotenone, which is a widely used pesticide that induces Parkinson-like symptoms in animal models. 5 Cellular oxidases also generate ROS Dedicated ˙O2‐ producer NADPH oxidase (NOX or “respiratory burst” oxidase) : NADPH + 2O2 NADP+ + 2 ˙O2‐ + H+ (In phagocytes, NOX‐based ROS generation is required for killing invading pathogens) NADPH oxidases (Nox) – NADPH oxidases are enzymes dedicated in superoxide production in cells such as phagocytes for killing invading pathogens. NADPH oxidases are also expressed in non-phagocytic cells including cardiomyocytes, which may play a role in ROS- mediated cellular signaling. 6 Cellular oxidases also generate ROS Xanthine oxidase (purine catabolism): Xanthine + O2 Uric Acid + ˙O2‐ + H2O2 Monoamine oxidase type b (MAO‐b) (e.g.; in dopaminergic neurons) ROS Dopamine + O2 + H2O DOPAC + NH3 + H2O2 as byproducts (3, 4‐dihydroxyphenylacetic acid) Microsomal Ethanol Oxidizing System (MEOS): CH3CH2OH + NADPH +O2 CH3CHO + NADP+ + H2O + (˙O2‐) Xanthine oxidase (XO) and monoamine oxidase (MAO) - Other cellular oxidases may produce ROS as metabolic by-products. For example, xanthine oxidase (XO), predominantly located in the liver and endothelial cells, catalyzes purine catabolism. Monoamine oxidase type b (MAO-b) catalyzes dopamine catabolism in neuronal cells. These oxidases use molecular oxygen as oxidant and produce H2O2, O2- , or a mixture of the two. The production of O2- and H2O2 by XO and MAO-b are believed to contribute to oxidative stress in ischemia/reperfusion injury and the hypersensitivity of dopaminergic neurons to oxidative stress, respectively. Microsomal Ethanol Oxidizing System (MEOS, or CYP2E1) – Oxidation of ethanol by MEOS produces O2- (see lecture on Alcohol Metabolism) 7 3. Interconversion determines toxicity O2 Oxygen 1e¯ O2¯ Superoxide (Primary ROS) 1e¯ + 2H+ Auto - Dismutation (very slow) Superoxide Dismutase (fast) H2O2 Hydrogen Peroxide 1e¯ + 1H+ Fenton Reaction H2O + ˙OH Hydroxyl Radical 1e¯ + 1H+ H2O Water The superoxide anion radical ( O2-) spontaneously dismutes to O2 and hydrogen peroxide (H2O2), but at a very slow rate. Although H2O2 is less toxic than the superoxide anion radical ( O2-), it can also be converted into the highly reactive hydroxyl radical OH by the Fenton reaction. The Fenton reaction transfers an electron to H2O2 from free metal ions such as Fe2+, which is converted from the ferrous into the ferric (Fe3+) form. Fe3+ can be recycled back to Fe2+ by gaining an electron from O2-. In the net reaction, the presence of iron is truly catalytic, which converts hydrogen peroxide into hydroxyl radical and water. Thus, over-accumulation of free iron causes oxidative stress and human diseases (e.g., Friedreich’s Ataxia). Hydroxyl radical can also be produced by ionizing radiations (e.g,, UV and gamma rays), which induces homolytic fission of the O-O bond in H2O2. Free radical production is one of the mechanisms underlying the effects of cancer radiation therapy that kills actively proliferating tumor cells. 8 Fenton Reaction O2 Oxygen In the presence of metal ions 1e¯ (e.g., Fe and Cu) O2¯ Superoxide 1e¯ + 2H+ Fenton Reaction H2O2 (Ferrous) Hydrogen Peroxide 1e¯ + 1H+ (Ferric) H2O + ˙OH Hydroxyl Radical 1e¯ + 1H+ Fe H2O ˙O2‐ + H2O2 ˙OH + OH- + O2 Water 9 4. Reactive Nitrogen/Oxygen Species (RNOS) Nitric Oxide (NO˙) (a mild radical for redox NO synthase signaling) (NOS) Arginine + O2 + NADPH Citrulline + NADP+ + NO Peroxynitrite – produced from NO NO˙ + ˙O2‐ ONOO‐ (highly reactive like ˙OH) The chemical biology of reactive oxygen species is intimately linked to reactive nitrogen/oxygen species (RNOS). The primary RNOS produced by the cell is nitric oxide (NO ). NO is generated by specific nitric oxide synthase (NOS), which metabolizes arginine to citrulline with the formation of NO via a five electron oxidative reaction. NO is itself a mild radical with one unpaired electron (see above). However, NO can react with superoxide ( O2¯ ) to form peroxynitrite (ONOO¯), a very reactive oxidant. Because of their similar chemical properties, ROS and RNOS often act on similar or the same targets. 10 Summary – the five most common Reactive Oxygen Species ˙O2‐ superoxide H2O2 hydrogen peroxide ˙OH hydroxyl radical NO˙ nitric oxide ONOO‐ peroxynitrite 11 5. Oxidative Damage Irreversible damage DNA by ˙OH Lipids Reversible Thiol groups modifications by H2O2 in proteins 12 ˙OH causes DNA damage 8-oxodeoxyguanosine Consequence – Oxo dG mispairs with A ‐ G‐to‐T transversion ROS are mutagenic 8-OXO-dG can be measured by HPCL or monoclonal antibody DNA is an especially favored target of OH generated by Fenton reactions. OH extracts electrons from either sugar or base moieties. The resultant DNA radicals are resolved in a variety of ways, thereby producing a broad spectrum of lesions. A common oxidative lesion is the formation of 8-hydroxy-2’-deoxyguanosine (or 8-oxodeoxyguanosine), resulting from guanosine oxidation. The content of 8-hydroxydeoxyguanosine is frequently used as an indicator for the extent of DNA damage in the cell. 8-oxodeoxyguanosine can mispair with deoxyadenosine, leading to G-to-T transversion (i.e., an oxidized G pairs with A on the opposite strand. After the replication of the latter strand, the base on the originally damaged site becomes T). DNA damage is the first step in mutagenesis and carcinogenesis. 13 ˙OH causes lipid peroxidation No need to memorize ! Membrane damage Altered fluidity Malondialdehyde (MDA) 4-hydroxy-(2E)- nonenal (4-HNE) (Highly active aldehydes) OH is highly active in mediating lipid oxidation. Polyunsaturated acyl chains of phospholipids or polyunsaturated fatty acids (PUFA) such as arachidonic acid and linoleic acid are highly susceptible to peroxidation, as the hydrogens close to the double bonds are highly reactive and prone to lose electron to OH. Lipid peroxidation can initiate a free radical chain reaction in the membrane to produce lipid peroxide which causes membrane damage. Lipid peroxidation introduces charged peroxide group in the acyl chain of phospholipids and causes membrane damage. Furthermore, the non-enzymatic breakdown of lipid peroxidation products produces highly reactive aldehydes such as malondialdehyde (MDA) and 4- hydroxy-(2E)-nonenal (4-HNE). Accumulation of these products is often used as biomarkers for lipid peroxidation. 14 4‐HNE causes protein carbonylation 4-HNE 4-HNE OH OH Protein X + Protein X (X = His, Cys, Lys) O O Conformational change Loss of activity Protein turnover Hydroxyl radicals can directly oxidize amino-acid side chains, causing protein damage. More importantly, hydroxyl radicals mediate protein carbonylation, a process defined by the addition of reactive carbonyl functional groups on proteins. The most reactive and common carbonyl groups are the reactive aldehydes generated from lipid peroxidation such as 4-hydroxy-(2E)- nonenal (4-HNE), which react with the side chain of cysteine, hisidine and lysine residues (labeled as X in the following figure). The lipid peroxidation products can diffuse across membranes, allowing the reactive aldehyde-containing lipids to covalently modify proteins localized throughout the cell and relatively far away from the initial site of ROS formation. Carbonylation affects the activity of target proteins or cause them to become degraded by protein quality control machineries. 15 Summary ‐ Biomarkers for oxidative stress DNA damage 8‐OXO‐deoxyguanosine Lipid peroxidation Malondialdehyde (MDA) 4‐hydroxy‐(2E)‐nonenal (4‐HNE) 16 Excessive H2O2 causes protein damage H2O2 oxidizes SPECIFICALLY protein cysteinyl residues to form disulfide crosslinking in proteins R R SH + H2O2 S + 2H2O SH S + Anti-oxidants R (electron donors) R Sulfhydryl Disulfide group bond Loss of activity Protein aggregation H2O2 is not a free radical. It is two-electron oxidant and reacts poorly with most biological molecules. But it can oxidize some protein cysteinyl residues to form disulfide cross-links with other cysteines. The reaction of H2O2 with thiol proteins confers its role in cell signaling. 17 6. Defense mechanisms O2 Oxygen 1e¯ ˙O2¯ Superoxide 1. Conversion H2O2 2. Degradation Hydrogen Peroxide H2O 1e¯ + 1H+ 3. Scavenging by H2O + ˙OH antioxidants Hydroxyl Radical Removal 1e¯ + 1H+ H2O Water Cells are equipped with both enzymatic and non-enzymatic mechanisms for defense against ROS. Cells can convert the primary ROS, superoxide, into hydrogen peroxide by superoxide dismutase. Hydrogen peroxide is then converted into water by the catalase, glutathione peroxidase and peroxiredoxin pathways. If cells fail to remove hydrogen peroxide, hydroxyl radicals are generated. Hydroxyl radicals are scavenged by antioxidants, as the last line of defense. 18 Superoxide dismutase (SOD) converts ˙O2‐ into H2O2 SOD ‐ 2˙O2 + 2H+ O2 + H2O2 Superoxide dismutase (SOD) converts 2 molecules of O2‐ (one as electron donor and the other one as electron acceptor) into one molecule of O2 and one of the less toxic H2O2. 19 ENZYMATIC defense mechanisms (Superoxide conversion and peroxide destruction) SOD: superoxide dismutase Cytosol GPx: Glutathione peroxidase GR: Glutathione reductase “Sod1” Prx: Peroxiredoxin Trx: Thioredoxin TrxR: Thioredoxin reductase ICDH: NADP+-dependent Mito. “Sod2” Isocitrate Dehydrogenase TH: Transhydrogenase TCA cycle Redox relay Adapted from M.P. Murphy, Biochem. J. (2009) 417:1-13. SODs are compartmentalized in the cell: The cytosolic SOD (Sod1) contains one atom each of Cu and Zn. It is also known as Cu,Zn-SOD. The mitochondrial SOD (Sod2) uses Mn as a ligand. So it is also called the Mn-SOD. O2- can freely cross the mitochondrial outer but not inner membrane. Mn- SOD is therefore responsible for degrading O2- in the mitochondrial matrix. Three different enzymes/pathways decompose hydrogen peroxide Glutathione peroxidase - Glutathione peroxidase, which contains the micronutrient selenium (Se), converts H2O2 to water, consuming two molecules of reduced glutathione. Glutathione is a tripeptide with an unconventional structure (-Glu-Cys-Gly). It contains the amino acid cysteine, which bears a sulfhydryl group (-SH). This functional group is electron-rich and is the ‘business end’ of the molecule, hence the abbreviation, GSH. Like all sulfhydryls, GSH can, in the presence of an oxidizing agent such as H2O2, react with another sulfhydryl-containing compound (e.g., a second molecule of GSH) to form a disulfide after losing two electron. In this reaction reduced glutathione (GSH) is oxidized; the product is oxidized glutathione (GSSG). Reduced glutathione is regenerated by glutathione reductase, an NADPH-dependent enzyme. In mitochondria, NADPH is mainly produced by the TCA cycle enzyme, NADP-dependent isocitrate dehydrogenase. The peroxiredoxin pathway - The second mechanism for the disposal of H2O2 involves a conceptually similar pathway that uses a small sulfhydryl-containing protein, peroxiredoxin. Catalase - A heme-containing enzyme that catalyzes the decomposition of hydrogen peroxide to water and oxygen. Again, note that this is basically a reaction using one H2O2 molecule to reduce another. 20 The ALS Conundrum (Appendix 2) Amyotrophic Lateral Sclerosis (Lou Gehrig’s disease) FALS: Point mutations in the cytosolic CuZn‐SOD (Sod1) Oxidative stress is significant in ALS But: Mutant Sod1 may not necessarily have reduced activity. The mutant Sod1 is misfolded and becomes toxic. Current model – Sod1‐induced ALS is caused by the “gain of toxicity” Secondary oxidative stress (mechanism unknown) Sod1 is a very abundant protein in the cytosol. Specific missense and dominant mutations in Sod1 cause Amyotrophic Lateral Sclerosis (ALS or Lou Gehrig’s disease). Note that the mutant Sod1 is misfolded and becomes cytotoxic, but does not necessarily lose enzymatic activity. 21 7. Non‐enzymatic antioxidants ‐ Scavenging free radicals ‐ Protecting proteins against sulfhydryl oxidation by H2O2 22 Glutathione (tripeptide: ‐Glu‐Cys‐Gly) as a free radical scavenger H O O + H3N C CH 2 CH 2 C NH CH C NH CH 2 COO GSH COO CH 2 reduced glutathione SH Free radicals Sulfhydryl group Non-radicals GS-SG (Oxidized glutathione) In addition to its roles as a co-factor for the glutathione peroxidase and a free radical scavenger, free glutathione (GSH) is an important non-enzymatic antioxidant to keep sulfhydryls (or cysteines) of proteins reduced and maintains their biological activity. GSH is highly abundant in the cytosol, nuclei and mitochondria and is the major soluble antioxidant in these cell compartments. In the cytoplasm of a typical mammalian cell, there are many cysteine-containing proteins. For these proteins to remain functional, their sulfhydryl groups must remain reduced. This is often achieved by maintaining a high intracellular concentration of glutathione and a high ratio of GSH to GSSG. 23 Glutathione keeps sulfhydryls of proteins reduced Protein Protein High GSH/GSSG S SH + 2 GSH + GSSG S SH Low GSH/GSSG Another biomarker: GSH/GSSG ratio as an indicator of redox balance In the cytoplasm of a normal cell (GSH>>GSSG), mass action will drive the reactions toward the right direction (because the concentration of GSH is high relative to any proteins). If GSH becomes low relative to GSSG, formation of inactive disulfide-linked protein complexes is favored. Therefore, the GSH/GSSG ratio is often used as an indicator for the redox state of the cell. 24 Dietary free radical scavengers and supplements Vitamin E (‐tocopherol) Fat soluble ‐ protecting membrane lipids & lipoproteins Vitamin C (ascobate) – water soluble Plant polyphenols – e.g., resveratrol Flavonoids – e.g., quercetin (green tea) N‐Acetylcysteine, CoQ10, …etc 25 Scavenging of free radicals by Vitamin C ONOOˉ No need to ˙NO memorize ! O2 SQ˙ ˙O2ˉ ˙OH ˙NO2 Ascorbate (AA) Non‐ radicals AA˙ˉ (Ascorbyl radical) Disproportionation AA + Dehydroascorbate (DHA) Mechanism of free radical scavenging by antioxidants (e.g., ascorbic acid or vitamin C) – Vit. C is an excellent antioxidant because it reacts with a wide spectrum of radicals. After losing an electron to free radicals, ascorbate (AA) is converted into ascorbyl radicals (AA -). The preferred reaction of ascorbyl radicals is dispropotionation after collision of two molecules to give the non-radical ascorbate and dehydroascorbate (DHA). 26 ROS and human diseases Neurodegeneration (e.g., Parkinson’s disease) Cardiovascular diseases (atherosclerosis) Cancer Diabetes Hemolytic anemia Reperfusion injury Chronic Granulomatous Disease Friedreich’s Ataxia (FA) 27 Should we be taking megadoses of antioxidants such as Vitamins E and C every day? The Linus Pauling legacy: 18 g/day So far, large‐scale clinical trials indicate that megadoses of antioxidants may do more harm than good. 28 8. Physiological roles of ROS/RNOS ROS is part of our life, not an evolutionary drawback Reducing Cell proliferation Mild oxidizing Cell differentiation Moderate oxidizing Apoptosis Strong oxidizing Oxidative damage 29 Physiological roles of ROS/RNOS Oxidative folding (e.g., insulin maturation) Redox signaling for proliferation & differentiation Innate immunity (e.g., phagocytes) Apoptosis Inflammatory response Wound healing 30 Indiscriminate scavenging could desensitize anti‐cancer therapy ! (e.g., Radiotherapy) Adapted from Dolado and Nebreda, Cancer Cell (2008) 14:427‐429.) ROS have been proposed as common mediators for apoptosis and cellular senescence. A broad spectrum of models of oxidative stress, including H2O2, nitric oxide and derivatives, endotoxin-induced inflammation, photodynamic therapy, ultraviolet-A and ionizing radiations, have been shown to induce apoptosis. Apoptosis and cellular senescence are important for removing damaged cells to avoid mutagenesis, and to prevent cancer development and progression. Some studies suggested that antioxidant protection therapy in cancer patients should be used with caution. At the progression stage of cancer, lowering ROS by antioxidant therapy might actually stimulate growth of tumors by suppressing apoptosis which requires elevated levels of free radicals. Generally speaking, antioxidants can protect healthy people from cancer but promote the growth of pre-initiated tumor cells. Studies have shown that ROS production is required for preventing outgrowth of cells that are displaced from their natural microenvironment. Thus, antioxidants may promote the survival of pre-initiated tumor cells in unnatural environment and thus enhance malignancy. Finally, ROS production is a critical mediator of ionizing-radiation therapy of cancer. Excessive antioxidants can therefore contribute to tumor radioresistance. 31 Mitochondria 9. Summary NADPH oxidase Redox Xanthine oxidase Monoamine 5 ROS producers oxidase balance 5 ROS 4 biomarkers 3 defense lines = 1 electron charger ˙O2¯ Health Sod EnzymaticD efense Catalase H2O2 Cellular signaling GPx, Prx NADPH e- Fenton Pathogen reaction destruction Scavengers Apoptosis (Vit. C, Vit. E, ˙OH (anti-tumorigenic) GSH, etc.) 8-OXOdG DNA damage, MDA Protein damage, ONOO¯ Biomarkers 4-HNE Lipid peroxidation GSH/GSSG NO˙ ratio Diseases NO synthase 32