Lecture 8 Studyguide Start of Material for Test 2 (Module 06 Part B) - Ecology PDF
Document Details
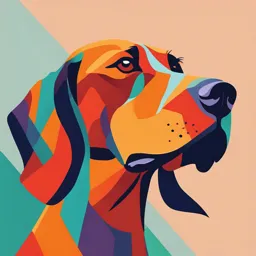
Uploaded by LuxuryOrphism
Tags
Summary
This document is a study guide for a lecture on ecology, module 06 part b, providing a summary of topics like size and its effect on organism function, and evolution, as well as adaptations to environmental pressures and resource acquisition.
Full Transcript
Size indeed plays a crucial role in shaping the function and evolution of organisms across various taxa. This phenomenon, known as allometry, describes how biological traits change in relation to the size of an organism. Let's delve into how size imposes fundamental constraints and influences the fu...
Size indeed plays a crucial role in shaping the function and evolution of organisms across various taxa. This phenomenon, known as allometry, describes how biological traits change in relation to the size of an organism. Let's delve into how size imposes fundamental constraints and influences the function and evolution of organisms: 1.Metabolic Rate and Energy Requirements: As organisms grow larger, their metabolic rate typically increases, but not at the same rate. This means that larger organisms need more energy to sustain themselves. Consequently, there's a trade-off between size and metabolic efficiency. For instance, small organisms like insects have higher metabolic rates per unit of mass compared to larger animals like elephants. This constraint influences various aspects of an organism's biology, such as reproduction, locomotion, and foraging behavior. 2.Surface Area to Volume Ratio: As organisms increase in size, their volume (and thus their mass) increases at a faster rate than their surface area. This has implications for processes like gas exchange, nutrient absorption, and heat dissipation. For example, small organisms with a high surface area to volume ratio can efficiently exchange gases through diffusion across their body surface, whereas larger organisms require specialized respiratory structures like lungs or gills to facilitate efficient gas exchange. 3.Mechanical Constraints: The structural integrity of an organism is influenced by its 1 size. Larger organisms face greater mechanical challenges due to increased gravitational forces acting on their bodies. This necessitates adaptations in skeletal and muscular systems to support larger body masses and withstand mechanical stress. Conversely, smaller organisms benefit from their reduced weight and can exploit different habitats and niches inaccessible to larger counterparts. 4. Reproductive Strategies: Size often dictates reproductive strategies. In many species, larger individuals have higher reproductive output and invest more resources in each offspring. This is known as "big-bang" reproduction. Conversely, smaller organisms tend to produce more offspring with less parental investment per individual, a strategy known as "r-selected" reproduction. These strategies are shaped by ecological factors such as predation pressure, resource availability, and habitat stability. 5.Ecological Interactions: Size influences interactions between organisms within ecological communities. For example, predation dynamics are often influenced by the size relationship between predators and their prey. Larger predators may target larger prey due to their higher energy content, while smaller predators may specialize in hunting smaller prey. Similarly, competition for resources can be influenced by size, with larger individuals often having access to higher quality resources or dominating competitive interactions. 6.Evolutionary Trajectories: Size evolution is subject to both selective pressures and genetic constraints. Natural selection acts on traits that enhance an organism's fitness within its environment, including size-related traits. However, genetic and developmental constraints may limit the range of possible sizes or constrain the rate at which size can evolve. Additionally, evolutionary trends in size can be influenced by factors such as climate change, habitat fragmentation, and interspecies interactions. In summary, size imposes fundamental constraints on the function and evolution of organisms through its effects on metabolism, physiology, mechanical structure, reproductive strategies, ecological interactions, and evolutionary trajectories. Understanding these size-related constraints is essential for elucidating the mechanisms driving organismal diversity and adaptation across different scales of biological organization. 1 1.Adaptations & Trade-offs: Organisms often face trade-offs when adapting to their environments, and size is a crucial factor in these adaptations. For instance, larger body size may provide advantages such as increased strength, predator avoidance, or access to larger prey. However, it also comes with trade-offs, such as higher energy requirements, reduced agility, or slower reproductive rates. Organisms evolve various adaptations to mitigate these trade-offs and optimize their fitness in their specific ecological niche. 2.Size as a Fundamental Constraint: Size serves as a fundamental constraint on the function of organisms and the evolution of populations. This constraint arises due to the interplay between an organism's size and its physiological, ecological, and evolutionary processes. For example, the size of an organism influences its metabolic rate, locomotion efficiency, reproductive strategies, and interactions with other organisms within its ecosystem. 3.Adaptations Related to Food Resources: Organisms adapt their size and feeding strategies based on the availability and distribution of food resources in their environment. For example, herbivores may evolve larger body sizes to efficiently process low-nutrient plant material, while carnivores may have smaller, more agile bodies to pursue and capture prey. Additionally, organisms may develop specialized feeding structures or behaviors to exploit specific food sources, such as the long neck 2 of giraffes for browsing leaves high in trees. 4. Adaptations Related to Environmental Variation (Conform/Regulate): Organisms can either conform to or regulate their internal physiological processes in response to environmental variation, including changes in temperature, humidity, and resource availability. Conforming organisms adjust their physiology passively to match environmental conditions, while regulating organisms actively maintain internal homeostasis despite external fluctuations. Size influences an organism's ability to conform or regulate; for example, smaller organisms may have less thermal inertia and thus experience greater temperature fluctuations, while larger organisms may have more resources to invest in regulatory mechanisms. 5.Traits & Mechanisms of Energy/Temperature Balance: Organisms employ various traits and mechanisms to manage their energy and temperature balance in response to size-related constraints and environmental challenges. These may include physiological adaptations such as insulation, thermoregulation, and metabolic adjustments, as well as behavioral strategies like seeking shelter, adjusting activity patterns, or migrating to more favorable habitats. For example, small mammals in cold climates may have thick fur and hibernate to conserve energy and maintain body temperature during winter months. In summary, size plays a central role in shaping the adaptations, trade-offs, and ecological interactions of organisms. Understanding how size influences the function and evolution of populations is essential for elucidating the mechanisms underlying biological diversity and ecosystem dynamics in response to environmental change. 2 Maintaining oxygen balance is essential for the survival of organisms, and different taxa face unique challenges related to environmental variation in oxygen availability. Let's explore how four different organisms— an insect, a mammal, a scallop, and a fish— address this challenge: a. Insect: Insects rely on a system of tracheae and tracheoles for gas exchange. Air enters the body through openings called spiracles and travels through a network of tracheal tubes to reach the cells. Oxygen diffuses directly from the tracheoles into the cells, where it is used for aerobic respiration. However, the tracheal system poses limitations for larger insects, as diffusion becomes less efficient over longer distances. As a result, some larger insects have evolved mechanisms such as spiracles that can open and close to regulate gas exchange, and active ventilation of the tracheal system through body movements. Additionally, some insects may exhibit behavioral adaptations, such as seeking out areas with higher oxygen concentrations or adjusting their metabolic rate in response to oxygen availability. b. Mammal: Mammals have a respiratory system consisting of lungs, where gas exchange occurs between the air in the lungs and the bloodstream. Oxygen from the air is absorbed into the bloodstream in the alveoli, small sacs within the lungs, and is transported to cells throughout the body by the circulatory system. Mammals face challenges in maintaining oxygen balance, particularly in environments with low 3 oxygen levels or high altitudes. To cope with these challenges, mammals may exhibit physiological adaptations such as increased lung capacity or efficiency, increased production of red blood cells to enhance oxygen transport, and alterations in breathing rate and depth. For example, mammals living at high altitudes, like the Tibetan yak or the Andean condor, may have evolved specialized adaptations to thrive in low-oxygen environments. c. Scallop: Scallops are marine bivalve mollusks that inhabit shallow coastal waters. They rely on gills for gas exchange, where oxygen from the surrounding water is absorbed into the bloodstream and carbon dioxide is released. Scallops face challenges related to environmental variation in water quality, temperature, and oxygen levels. In environments with low oxygen concentrations, such as hypoxic or anoxic zones, scallops may experience physiological stress or suffocation. To cope with these challenges, scallops have evolved mechanisms such as increasing the pumping rate of water over their gills or moving to areas with higher oxygen levels. Additionally, some species of scallops exhibit behavioral adaptations, such as burrowing into sediments or adjusting their feeding and metabolic rates in response to changes in oxygen availability. d. Fish: Fish have gills for gas exchange, where oxygen from the water is absorbed into the bloodstream and carbon dioxide is released. Fish face challenges related to environmental variation in water quality, temperature, and oxygen levels. In aquatic environments, oxygen availability can fluctuate due to factors such as temperature, pollution, and eutrophication. Fish have evolved various adaptations to cope with these challenges, including increasing ventilation rate and gill surface area, adjusting hemoglobin affinity for oxygen, and modifying behavior to seek out areas with higher oxygen concentrations. Some fish species, such as air-breathing fish like lungfish or mudskippers, have also evolved the ability to supplement oxygen uptake from the water with aerial respiration or by breathing air directly from the atmosphere. In summary, maintaining oxygen balance is critical for the survival of organisms, and different taxa have evolved a variety of physiological, anatomical, and behavioral adaptations to cope with environmental variation in oxygen availability. These adaptations enable organisms to thrive in diverse habitats and ecological niches, despite fluctuations in oxygen levels. 3 4 Maintaining water balance is crucial for the survival of organisms, particularly in environments where water availability fluctuates or where there are challenges related to osmotic regulation. Let's explore how two different organisms—a frog and a marine organism facing osmoregulation challenges in a saltwater environment— address these challenges: a. Frog: Frogs are amphibians that typically inhabit freshwater habitats, but they can also adapt to terrestrial environments for part of their life cycle. Maintaining water balance is essential for frogs, as they are susceptible to dehydration on land and face challenges related to water loss and gain in aquatic environments. Frogs have evolved various physiological and behavioral adaptations to cope with these challenges. Physiological Adaptations: Frogs have permeable skin that allows for cutaneous respiration and water exchange. While this facilitates gas exchange, it also makes them susceptible to water loss through evaporation. To mitigate water loss, frogs produce mucous secretions that help keep their skin moist, and some species have adaptations such as waxy coatings or thicker skin layers. Frogs have efficient renal systems that regulate water and ion balance. They excrete excess water and ions through their kidneys while reabsorbing essential nutrients and conserving water. 5 During times of water scarcity, frogs may enter a state of dormancy or estivation, where metabolic activity decreases, and they conserve water by minimizing activity and seeking out moist microhabitats. Behavioral Adaptations: Frogs are often found near water bodies, where they can replenish water lost through evaporation and maintain hydration. They may also exhibit behaviors such as burrowing into damp soil or hiding under vegetation during periods of drought to conserve water. b. Osmoregulation in a Saltwater Environment: Organisms living in saltwater environments face the challenge of maintaining water balance in the face of osmotic pressure that draws water out of their bodies. This is particularly challenging for marine organisms, as seawater has a higher salt concentration than their internal fluids. Physiological Adaptations: Marine organisms have specialized osmoregulatory organs, such as salt glands or ion transport mechanisms in their gills, that enable them to regulate internal salt concentrations. They may also drink seawater and excrete excess salts through specialized excretory organs or by secreting concentrated urine. Some marine organisms, such as marine teleost fish, have developed efficient mechanisms for water retention, including impermeable skin and low rates of water loss through urine or respiration. Behavioral Adaptations: Marine organisms may migrate to areas with more favorable salinity conditions, such as estuaries or brackish water habitats, where they can maintain water balance more easily. They may also adjust their feeding behavior to minimize salt intake or seek out sources of freshwater, such as rainfall or freshwater runoff. In summary, maintaining water balance is essential for organisms to survive and thrive in their respective environments. Frogs and marine organisms have evolved a suite of physiological and behavioral adaptations to cope with challenges related to water balance, allowing them to exploit diverse habitats and ecological niches. 5 Certainly! Let's delve into the challenges related to environmental variation faced by homeotherms and poikilotherms, and the concepts of temperature conformity and regulation: 1.Homeotherms: Homeotherms are organisms that maintain a relatively constant internal body temperature regardless of external environmental conditions. This group includes mammals and birds primarily. Maintaining a stable body temperature provides several advantages, including consistent enzyme function, optimal metabolic rates, and enhanced physiological performance. 2.Challenges: 1. Homeotherms face the challenge of thermoregulation, especially in environments with fluctuating temperatures. They must expend energy to maintain their body temperature within a narrow range, which can be particularly challenging in extreme climates. 2. Homeotherms have higher metabolic rates compared to poikilotherms, which require a constant supply of energy to sustain thermoregulation. This can be especially demanding during periods of food scarcity or environmental stress. 3.Poikilotherms: Poikilotherms, also known as ectotherms, are organisms whose internal body temperature varies with the temperature of their surroundings. This 6 group includes most invertebrates, fish, amphibians, and reptiles. Poikilotherms rely on external sources of heat, such as sunlight or warm environments, to regulate their body temperature. 4. Challenges: 1. Poikilotherms are more susceptible to fluctuations in environmental temperature, which can impact their physiological processes and behavior. Extreme temperatures can impair metabolic function, reduce activity levels, and increase susceptibility to predation or disease. 2. Poikilotherms may experience periods of inactivity or dormancy during colder temperatures, known as torpor or hibernation, to conserve energy and survive harsh environmental conditions. 5.Temperature Conformers & Regulators: 1. Temperature Conformers: Organisms that are temperature conformers have internal body temperatures that closely mirror the temperature of their surrounding environment. They do not actively regulate their body temperature but instead passively conform to external conditions. Many poikilotherms fall into this category. 2. Temperature Regulators: Organisms that are temperature regulators actively maintain a relatively constant internal body temperature, regardless of external temperature fluctuations. Homeotherms, such as mammals and birds, are primary examples of temperature regulators. 6.Example - Ambient Temperature vs. Body Temperature: 1. In a temperature conformer, such as a lizard basking in the sun, the lizard's body temperature closely matches the temperature of its surroundings. When the lizard moves to a cooler area, its body temperature decreases accordingly. 2. In a temperature regulator, such as a mammal like a human, the body maintains a constant internal temperature even when ambient temperatures fluctuate. For instance, a human's body temperature remains relatively stable around 37°C (98.6°F) regardless of whether it's hot or cold outside. In summary, environmental variation poses distinct challenges for homeotherms and poikilotherms in maintaining thermal balance. While homeotherms regulate their body temperature internally to withstand environmental fluctuations, poikilotherms rely on behavioral and physiological adaptations to cope with changing temperatures. Understanding temperature conformity and regulation provides insights into how different organisms adapt to their environments and cope with temperature variability. 6 Certainly! Homeotherms, also known as endotherms, utilize metabolic energy to maintain a constant body temperature through various physiological mechanisms. Let's delve into how these mechanisms work in detail: 1.Metabolic Heat Production: Homeotherms generate metabolic heat through the oxidation of nutrients, primarily fats and carbohydrates, in their cells. This process occurs continuously as part of cellular respiration, which releases energy in the form of heat that contributes to maintaining a stable internal body temperature. 2.Regulation of Metabolic Rate: Homeotherms can adjust their metabolic rate to regulate heat production according to environmental conditions and physiological demands. For example, during periods of increased activity or exposure to cold temperatures, homeotherms can increase their metabolic rate to generate more heat and maintain body temperature within the optimal range. 3.Insulation: Homeotherms possess specialized insulating structures, such as fur, feathers, or layers of fat, that help retain generated heat and minimize heat loss to the external environment. These insulating layers act as barriers against thermal conductivity, reducing the transfer of heat between the body and the surrounding environment. 4.Vascular Control: Homeotherms can regulate blood flow to peripheral tissues and extremities through vasodilation and vasoconstriction mechanisms. In cold 7 environments, vasoconstriction reduces blood flow to the skin, conserving heat and maintaining core body temperature. Conversely, in warm environments, vasodilation increases blood flow to the skin, facilitating heat dissipation through convection and radiation. 5. Behavioral Thermoregulation: Homeotherms exhibit various behavioral strategies to regulate body temperature effectively. These behaviors include seeking shelter or shade to avoid excessive heat exposure, basking in the sun to increase heat absorption, or huddling with conspecifics to conserve body heat through mutual warmth. 6.Thermogenic Mechanisms: Some homeotherms possess specialized thermogenic tissues, such as brown adipose tissue (BAT), which can generate heat through nonshivering thermogenesis. BAT contains a high density of mitochondria and uncoupling protein 1 (UCP1), allowing for the uncoupling of oxidative phosphorylation from ATP production and resulting in the production of heat as a byproduct. 7.Temperature Regulation in Core Organs: Homeotherms prioritize the maintenance of stable temperatures in vital organs, such as the brain and heart, to ensure optimal physiological function. This regulation is achieved through thermoregulatory mechanisms that prioritize blood flow to these organs and ensure their thermal stability, even at the expense of peripheral tissues. In summary, homeotherms utilize metabolic energy and a suite of physiological and behavioral mechanisms to maintain a constant internal body temperature, irrespective of external environmental conditions. These mechanisms enable homeotherms to thrive in diverse habitats and climates, providing them with a competitive advantage in ecological niches where temperature stability is critical for survival. 7 Certainly! Poikilotherms, also known as ectotherms, rely on external sources of heat to regulate their body temperature. Unlike homeotherms, which generate metabolic heat internally to maintain a constant body temperature, poikilotherms adjust their body temperature by seeking out suitable environmental conditions or microhabitats. Let's explore how ectothermic mechanisms work in poikilotherms and how they find the right environment or microhabitats: 1.Behavioral Thermoregulation: Poikilotherms exhibit various behaviors to regulate their body temperature effectively. These behaviors include: 1. Basking in the sun: Many ectotherms, such as reptiles and amphibians, bask in sunlight to absorb heat energy. They may position themselves in open areas or on elevated surfaces to maximize exposure to sunlight. 2. Seeking shade or shelter: To avoid overheating, ectotherms may seek out shaded areas or sheltered habitats during the hottest parts of the day. This helps prevent excessive heat absorption and reduces the risk of dehydration. 3. Burrowing or hiding: Some ectotherms, such as reptiles and amphibians, burrow into the soil or hide under rocks or vegetation to regulate their body temperature. Burrowing provides insulation from extreme temperatures and helps maintain a more stable thermal environment. 8 2. Habitat Selection: Ectotherms are highly influenced by their choice of habitat, as different environments offer varying temperature gradients and microclimates. By selecting specific habitats, ectotherms can optimize their thermoregulation strategies. For example: 1. Aquatic ectotherms, such as fish and amphibians, may inhabit regions of water bodies with specific temperature ranges that suit their physiological needs. 2. Terrestrial ectotherms, such as reptiles and insects, may occupy habitats with diverse thermal profiles, ranging from sun-exposed rocks to shaded forest floors. 3.Microhabitat Utilization: Ectotherms often exploit microhabitats within their environment to regulate their body temperature more precisely. These microhabitats may offer localized temperature gradients or thermal refuges that ectotherms can use to their advantage. For example: 1. Rocks, logs, or other thermal masses: Ectotherms may thermoregulate by moving between sun-warmed surfaces and shaded areas provided by rocks, logs, or other thermal masses. These structures can act as thermal refuges, allowing ectotherms to maintain body temperature within their preferred range. 2. Vegetation cover: In forested habitats, ectotherms may utilize vegetation cover to regulate their body temperature. They may perch on branches or foliage to access cooler or warmer microclimates, depending on their thermal requirements. 4.Seasonal and Daily Patterns: Ectotherms adjust their thermoregulatory behaviors and habitat selection patterns in response to seasonal and daily fluctuations in temperature. For example: 1. Many ectotherms are more active during the warmer parts of the day and may retreat to cooler microhabitats or enter torpor during colder periods. 2. Seasonal changes in temperature can influence the distribution and abundance of ectotherms, as they may migrate or hibernate to avoid extreme environmental conditions. In summary, ectothermic mechanisms in poikilotherms involve behavioral adaptations, habitat selection, and microhabitat utilization to regulate body temperature effectively. By selecting appropriate environments and exploiting thermal gradients within their habitats, ectotherms can optimize their thermoregulatory strategies and thrive in diverse ecological niches. 8 It seems like there might be missing information regarding the specific type of lizards and the graph you're referring to. However, based on the description provided, it appears that the relationship between body temperature and sprint speed follows a pattern of an optimal temperature range for sprint performance. 1.Optimal Temperature for Sprint Speed: From the description, it seems that there is an optimal temperature range at which sprint speed is highest. This optimal range likely represents the temperature at which physiological processes associated with muscle function and neural activity are most efficient, leading to peak sprint performance. Lizards would exhibit their highest sprint speeds within this optimal temperature range. 2.Tolerance Range: The tolerance range refers to the range of temperatures within which the lizards can function and survive. From the description provided, it seems that sprint speed is lowest when the body temperature is low and when it's at a higher range, suggesting that there are limits to the lizards' thermal tolerance for sprinting. The full tolerance range would encompass the temperatures at which sprint speed is both low and high, indicating the boundaries within which the lizards can maintain physiological function. 3.Uncertainty in Sprint Speed Measurements: The most uncertain measurements of sprint speed would likely occur at temperatures where the relationship between body 9 temperature and sprint speed transitions from low to high or from high to low. In other words, the points where the slope of the graph changes direction represent critical temperature thresholds where small variations in body temperature could lead to significant changes in sprint speed. At these transition points, the lizards may be operating close to their thermal limits, making it challenging to predict sprint speed accurately. Therefore, measurements of sprint speed in the middle range of body temperatures, where the transition occurs, would be the most uncertain. In summary, the optimal temperature for sprint speed would be within a specific range where physiological processes are maximally efficient. The tolerance range encompasses the full range of temperatures within which the lizards can function, with uncertainty in sprint speed measurements occurring at critical temperature thresholds where the relationship between body temperature and sprint speed transitions. 9 The type of phenotypic plasticity you're referring to, where an organism exhibits different phenotypes in response to seasonal temperature variation, is called "seasonal acclimatization" or "seasonal acclimation." This phenomenon involves changes in an organism's physiological, morphological, or behavioral traits in response to predictable changes in environmental conditions, such as temperature fluctuations between seasons. During seasonal acclimatization, poikilothermic organisms adjust their metabolic rates, thermal tolerance limits, locomotor performance, and other traits to cope with changing environmental temperatures. These adjustments help them optimize their physiological function and maintain fitness across different seasons. For example, a lizard may exhibit changes in its sprint speed, thermoregulatory behavior, or metabolic rate in response to shifts in ambient temperature between summer and winter months. Overall, seasonal acclimatization allows poikilothermic organisms to adapt to varying environmental conditions throughout the year, enhancing their survival and reproductive success in dynamic habitats. 10 Periods of torpor in heterotherms refer to temporary states of reduced metabolic activity and physiological function, often accompanied by lowered body temperature and decreased responsiveness to external stimuli. Heterotherms are organisms that exhibit variability in their metabolic rates and body temperatures, transitioning between periods of torpor and activity in response to environmental conditions or metabolic demands. During torpor, heterotherms conserve energy and minimize resource consumption by lowering their metabolic rate and entering a state of reduced activity. This adaptive strategy is particularly common in small mammals, such as bats, rodents, and some marsupials, as well as in certain bird species. Torpor allows heterotherms to survive periods of food scarcity, harsh environmental conditions, or energy depletion, enhancing their ability to persist in challenging habitats. There are different types of torpor observed in heterotherms: 1.Daily Torpor: Some heterotherms, particularly small mammals and birds, may enter brief periods of torpor lasting several hours each day. Daily torpor helps conserve energy during periods of inactivity, such as nighttime or unfavorable weather conditions, while still allowing the animal to resume normal activity when conditions improve. 2.Seasonal Torpor (Hibernation): Many heterotherms undergo prolonged periods of 11 torpor during the winter months in a state known as hibernation. Hibernation allows animals to survive cold temperatures and food shortages when resources are scarce. During hibernation, metabolic rate and body temperature are significantly reduced, often near the freezing point, enabling the animal to conserve energy over extended periods. 3. Aestivation: In addition to hibernation, some heterotherms enter a state of torpor known as aestivation during hot and dry periods. Aestivation helps animals conserve water and cope with high temperatures and limited food availability in arid environments. During aestivation, metabolic rate and activity are reduced to minimize water loss and energy expenditure. Overall, periods of torpor in heterotherms represent adaptive responses to environmental challenges, allowing these organisms to survive and thrive in diverse habitats and climates. Torpor helps heterotherms conserve energy, reduce resource requirements, and withstand fluctuations in food availability, temperature, and other environmental factors. 11 The surface area to volume ratio plays a crucial role in determining the metabolic rates of organisms, as it affects their ability to exchange heat and gases with the environment. This relationship results in trade-offs between surface area and volume, impacting metabolic rates across different body sizes. The mouse and elephant example you provided illustrates these trade-offs effectively. 1.Surface Area to Volume Ratio Trade-Off: 1. As organisms increase in size, their volume (and thus their mass) increases at a faster rate than their surface area. This is due to the geometric properties of three-dimensional shapes. For instance, if you double the size of a cube, its volume (and mass) increases by a factor of eight, while its surface area increases only by a factor of four. 2. This means that larger organisms have a relatively smaller surface area compared to their volume, resulting in a lower surface area to volume ratio. Conversely, smaller organisms have a relatively larger surface area compared to their volume, leading to a higher surface area to volume ratio. 2.Metabolic Rates and Body Size: 1. Metabolic rates are closely linked to an organism's body size. Smaller organisms generally have higher metabolic rates per unit of mass 12 compared to larger organisms. This is because smaller organisms have a higher surface area to volume ratio, which facilitates more efficient exchange of gases (such as oxygen and carbon dioxide) and heat with the environment. 2. As a result, smaller organisms like mice have higher oxygen consumption rates relative to their body mass compared to larger organisms like elephants. Mice have a higher surface area to volume ratio, allowing them to exchange gases more efficiently, which in turn supports their higher metabolic rates. 1.Energy Requirements and Trade-Offs: 1. Higher metabolic rates in smaller organisms like mice come with the trade-off of increased energy requirements. To sustain their high metabolic rates, small animals need to consume more food relative to their body mass compared to larger animals. This is why small animals are often observed eating frequently throughout the day. 2. In contrast, larger organisms like elephants have lower metabolic rates per unit of mass, resulting in lower energy requirements. While elephants have a larger overall energy intake due to their greater body mass, they can sustain themselves on lower-quality food sources and may not need to eat as frequently as smaller animals. In summary, the trade-offs between surface area and volume influence metabolic rates in organisms of different sizes. Smaller organisms like mice have higher metabolic rates per unit of mass due to their higher surface area to volume ratio, while larger organisms like elephants have lower metabolic rates per unit of mass due to their lower surface area to volume ratio. These adaptations reflect the complex interplay between body size, metabolic demands, and environmental constraints across different species. 12 Indeed, the relationship between surface area, volume, and metabolism is fundamental in understanding how organisms' metabolic rates scale with size. Here's a detailed explanation: 1.Surface Area to Volume Ratio: 1. As organisms increase in size, their volume (and thus their mass) increases at a faster rate than their surface area. This is due to the geometric properties of three-dimensional shapes. For example, if you double the size of a cube, its volume (and mass) increases by a factor of eight, while its surface area increases only by a factor of four. 2. This leads to a decrease in the surface area to volume ratio as organisms grow larger. Larger organisms have relatively less surface area available for exchange compared to their volume, while smaller organisms have relatively more surface area. 2.Metabolism and Surface Area: 1. Metabolism, particularly oxygen consumption in aerobic organisms, is closely related to surface area because it governs the exchange of gases (such as oxygen and carbon dioxide) with the environment. 2. Smaller organisms have a higher surface area to volume ratio, allowing for more efficient exchange of gases. As a result, smaller organisms tend 13 to have higher metabolic rates per unit of mass compared to larger organisms. This relationship is known as mass-specific metabolism. 3. The higher surface area to volume ratio of smaller organisms facilitates greater heat and gas exchange per unit of mass, supporting higher metabolic rates necessary to sustain cellular processes. 1.Mass-Specific Metabolism: 1. Mass-specific metabolism refers to the metabolic rate per unit of body mass. It describes how metabolic rates scale with organism size. In general, smaller organisms have higher mass-specific metabolic rates, while larger organisms have lower mass-specific metabolic rates. 2. This decrease in mass-specific metabolism with increasing body size is due to the decrease in surface area to volume ratio. Larger organisms have relatively less surface area available for exchange per unit of mass, leading to lower mass-specific metabolic rates compared to smaller organisms. 2.Ecological Implications: 1. The scaling of metabolism with body size has important ecological implications. Smaller organisms with higher mass-specific metabolic rates require more energy per unit of body mass to sustain themselves. They may need to consume food more frequently and in larger quantities relative to their body size. 2. Larger organisms, with their lower mass-specific metabolic rates, can sustain themselves on lower-quality food sources and may have lower energy requirements per unit of body mass. They may also be less vulnerable to fluctuations in resource availability compared to smaller organisms. In summary, the trade-offs between surface area, volume, and metabolism underpin the scaling of metabolic rates with organism size. Smaller organisms have higher mass-specific metabolic rates due to their higher surface area to volume ratio, while larger organisms have lower mass-specific metabolic rates due to their lower surface area to volume ratio. 13 The future for species in human-modified environments, such as cities, is characterized by both opportunities and challenges. Here are some potential tradeoffs of the faster evolution of species in urban environments: Pros: 1.Rapid Adaptation: Species living in cities may undergo rapid evolutionary changes to cope with novel environmental conditions, such as pollution, artificial light, and altered habitat structures. This rapid adaptation could enhance their survival and reproductive success in urban landscapes. 2.Increased Biodiversity: Some species may thrive in urban habitats and diversify into new ecological niches. Urban environments can provide a wide range of resources and opportunities for adaptation, leading to increased biodiversity within cities. 3.Human-Wildlife Coexistence: Species that can adapt to urban environments may coexist more successfully with humans, potentially reducing conflicts between wildlife and human activities. This could lead to greater appreciation and conservation efforts for urban wildlife. 4.Ecosystem Services: Urban-adapted species may provide valuable ecosystem services, such as pest control, pollination, and waste management, which contribute to the functioning and resilience of urban ecosystems. Cons: 14 1. Genetic Bottlenecks: Rapid evolutionary changes in response to urbanization may lead to genetic bottlenecks, reducing genetic diversity within populations. This could make species more vulnerable to environmental changes and decrease their longterm adaptive potential. 2.Maladaptation: Some evolutionary changes in urban-adapted species may result in maladaptation to natural environments outside of cities. Traits that provide a fitness advantage in urban habitats may be detrimental in natural ecosystems, limiting the species' ability to thrive outside of human-modified environments. 3.Altered Ecosystem Dynamics: Rapid evolution in urban-adapted species may disrupt natural ecosystem dynamics and interactions. For example, the spread of invasive species or the loss of native species could have cascading effects on food webs and ecosystem functioning. 4.Urbanization Pressures: The rapid pace of urbanization and habitat modification may outpace the ability of some species to adapt or disperse to new habitats. This could lead to population declines, local extinctions, and fragmentation of habitat networks, reducing overall biodiversity. In summary, the faster evolution of species in human-modified environments presents both opportunities and challenges for biodiversity conservation and ecosystem management. Understanding the trade-offs associated with urban adaptation can inform strategies for sustainable urban planning, habitat restoration, and wildlife conservation efforts in cities. 14 Animal adaptations play a crucial role in determining the resilience and survival of species in response to human-induced changes to the environment. Here's how animal adaptations interface with human-driven environmental changes: 1.Flexibility and Plasticity: 1. Some species possess phenotypic plasticity, allowing them to adjust their behavior, physiology, or morphology in response to changing environmental conditions. This flexibility enables species to cope with human modifications to their habitats, such as altered resource availability, pollution, or habitat fragmentation. 2.Urban Adaptations: 1. Many species have adapted to urban environments, exhibiting behaviors or traits that enhance their survival and reproduction in cities. Examples include changes in foraging behavior, nest construction, or tolerance to human disturbance. Urban-adapted species may exploit novel resources, such as artificial light or food waste, to thrive in urban landscapes. 3.Coexistence and Exploitation: 1. Some species may exploit human-altered environments to their advantage. For example, certain bird species have adapted to nesting on buildings or bridges, while others have adapted to foraging in agricultural 15 fields or scavenging in urban areas. These adaptations allow species to coexist with humans and even benefit from human activities. 4. Extinction and Decline: 1. On the other hand, many species may struggle to adapt to rapid humaninduced environmental changes, leading to population declines, local extinctions, or range contractions. Habitat destruction, pollution, climate change, and invasive species can pose significant challenges to species with limited adaptive capacity. 5.Evolutionary Responses: 1. In some cases, species may undergo rapid evolutionary changes in response to human-induced selective pressures. This may include shifts in behavior, morphology, or life history traits to better exploit or cope with anthropogenic changes. However, the pace of human-induced environmental changes may outstrip the ability of some species to evolve adaptive responses, leading to population declines or extinctions. 6.Hope for Persistence: 1. There are instances where adaptations to extreme natural environments offer hope for the persistence of species in human-altered environments. For example, species adapted to extreme conditions such as toxic sulfide springs may possess genetic or physiological traits that confer resilience to human-caused environmental stressors, such as pollution or habitat degradation. In summary, animal adaptations play a critical role in mediating the impacts of human-induced changes to the environment. While some species may exhibit remarkable resilience and adaptability to human-altered landscapes, others may struggle to cope with rapid environmental transformations, leading to population declines or extinctions. Understanding the interplay between animal adaptations and human environmental changes is essential for informing conservation strategies and mitigating the negative impacts of human activities on biodiversity. 15 16 Vocab Size imposes fundamental constraints on animals, influencing their structure, function, and ecological interactions. Here's a breakdown of the consequences of size for animals, along with the challenges, adaptations, and responses associated with different resource acquisition strategies and environmental variations: 1. Size and Structure/Function: Large animals have proportionally more mass and volume, which affects physiological processes such as respiration, circulation, and locomotion. They may require more energy and resources to support their larger bodies. Small animals have a higher surface area-to-volume ratio, which facilitates heat exchange, gas exchange, and nutrient absorption. However, they may be more susceptible to heat loss and desiccation. 2. Challenges and Adaptations Associated with Resource Acquisition: Herbivores: Herbivores face the challenge of efficiently extracting nutrients from plant material, which can be structurally complex and contain cellulose. Adaptations include specialized digestive systems (e.g., fermentation chambers in ruminants) and symbiotic relationships with gut microbes that aid in cellulose digestion. Carnivores: Carnivores must capture and consume prey to obtain nutrients, requiring adaptations for hunting, killing, and processing animal tissue. Specialized teeth, claws, and sensory organs facilitate predation, while efficient digestive systems 17 maximize nutrient extraction from animal protein and fat. Omnivores: Omnivores consume a diverse diet of both plant and animal matter, requiring adaptations for processing a wide range of food types. Flexible digestive systems and generalized feeding structures allow omnivores to exploit varied food resources and adjust their diet based on availability. 3. Assimilation Efficiency: Assimilation efficiency refers to the ability of animals to extract and utilize nutrients from food. It is influenced by factors such as digestive physiology, gut microbial communities, and energy expenditure. Efficient digestion and absorption maximize nutrient uptake, supporting growth, reproduction, and energy balance. 4. Animal Responses to Environmental Variation: Homeostasis: Animals maintain internal stability through homeostatic mechanisms that regulate body temperature, blood pH, water balance, and nutrient levels. Homeostasis ensures optimal physiological function and survival in fluctuating environments. Oxygen Balance: Animals require oxygen for cellular respiration, which generates energy. Oxygen balance involves mechanisms for obtaining oxygen from the environment (e.g., lungs or gills), transporting it in the bloodstream, and regulating oxygen uptake and release at the cellular level. Water Balance: Animals regulate water balance through processes such as drinking, excretion, and osmoregulation. Adaptations for water conservation (e.g., kidneys that concentrate urine) and thermoregulation (e.g., evaporative cooling) help maintain water balance in diverse environments. Energy/Temperature Balance: Animals balance energy intake and expenditure to meet metabolic demands and maintain body temperature. Endothermic animals generate metabolic heat internally, while ectothermic animals rely on external heat sources. Behavioral adaptations (e.g., seeking shade or huddling for warmth) help regulate body temperature and conserve energy. 5. Thermoregulatory Strategies: Homeotherms: Homeotherms maintain a constant internal body temperature, typically through metabolic heat production and behavioral thermoregulation. They can withstand environmental temperature fluctuations while maintaining physiological function within narrow limits. Poikilotherms: Poikilotherms have variable body temperatures that fluctuate with environmental conditions. They rely on behavioral strategies (e.g., basking in sunlight) and physiological adjustments to regulate body temperature and metabolic activity. Heterotherms: Heterotherms exhibit flexible thermoregulatory strategies, transitioning between homeothermy and poikilothermy based on metabolic demands and environmental conditions. They may enter periods of torpor or hibernation to conserve energy during resource scarcity or extreme temperatures. 6. Endothermic vs. Ectothermic Metabolism: Endothermic: Endothermic animals regulate body temperature internally through 17 metabolic heat production. They have high energy requirements to sustain thermoregulation and maintain stable body temperature, enabling them to remain active in diverse environments. Ectothermic: Ectothermic animals rely on external heat sources to regulate body temperature. They have lower energy requirements compared to endotherms but may be limited by environmental temperatures. Ectotherms exhibit behavioral thermoregulation to optimize body temperature and metabolic function. 7. Torpor: Torpor is a physiological state of reduced metabolic activity and lowered body temperature, typically used by animals to conserve energy during periods of resource scarcity or environmental stress. Torpor can be facultative or obligatory and may vary in duration and depth depending on species and environmental conditions. In summary, animal adaptations to size constraints, resource acquisition strategies, and environmental variation are diverse and multifaceted. Understanding these adaptations and their ecological implications is essential for comprehending animal physiology, behavior, and survival strategies in dynamic environments. 17