Electron Transport & Oxidative Phosphorylation Lecture 4 PDF
Document Details
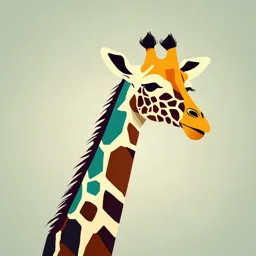
Uploaded by ImpeccableImagery
Nanyang Technological University
Prof. Dr. Gerhard Grüber
Tags
Summary
This lecture covers electron transport and oxidative phosphorylation, including diagrams and key questions. The document contains details about the process of cellular respiration and the electron transport chain.
Full Transcript
Electron transport and oxidative phosphorylation Prof. Dr. Gerhard Grüber Nanyang Technological University School of Biological Sciences [email protected] Acetyl CoA...
Electron transport and oxidative phosphorylation Prof. Dr. Gerhard Grüber Nanyang Technological University School of Biological Sciences [email protected] Acetyl CoA CoA-SH NADH + H+ H2O NAD+ Oxaloacetate Malate Citrate Isocitrate CITRIC NAD+ NADH ACID + H+ H2O CYCLE CO2 Fumarate CoA-SH a-Ketoglutarate CoA-SH FADH2 CO2 NAD+ FAD NADH Succinate Pi + H+ GTP GDP Succinyl CoA ADP ATP © 2017 Pearson Education, Ltd ATP yield per molecule of glucose at each stage of cellular respiration CYTOSOL Electron shuttles MITOCHONDRION span membrane 2 NADH or 2 FADH2 2 NADH 2 NADH 6 NADH 2 FADH2 GLYCOLYSIS PYRUVATE OXIDATION OXIDATIVE CITRIC PHOSPHORYLATION ACID Glucose 2 Pyruvate 2 Acetyl CoA CYCLE (Electron transport and chemiosmosis) + 2 ATP + 2 ATP + about 26 or 28 ATP Substrate-level Substrate-level Maximum per glucose: About 30 or 32 ATP © 2017 Pearson Education, Ltd Key questions of the lecture today Where in the mitochondria do electron transport and oxidative phosphorylation occur? What are reduction potentials, and how are they used to account for free energy changes in redox reactions? How is the electron-transport chain organized? How are the electrons of cytosolic NADH fed into electron transport? How does a proton gradient drive the synthesis of ATP? Diagram of the mitochondria and respiratory chain. Mathews, van Holde, Ahern: Biochemistry 3rd edition The fate of electrons removed from glucose Complete oxidation of glucose by molecular oxygen: C6H12O6 + 6 O2 → 6 CO2 + 6 H2O DGo’ = -2823 KJ/mol In the 1st half-reaction the glucose carbon atoms are oxidized: C6H12O6 + 6 H2O → 6 CO2 + 24 H+ + 24 e- in the second half-reaction the molecular oxygen is reduced: 6 O2 + 24 H+ + 24 e- → 12 H2O The 12 electron pairs involved in glucose oxidation are not transferred directly to O 2. They are transferred to the coenzymes NAD+ and FAD to form 10 NADH and 2 FADH2, which become oxidized in the electron-transport chain. Garett & Grisham: Biochemistry 4th edition Mitochondrial anatomy & FAD bound iCh enzyme enzymes are pump protons from matrix to embedded - internembrace space Cutaway diagram of a mitochondrion. The inner The proton and electrochemical gradients exiting across the membrane is highly folded into cristae. Heart inner mitochondrial membrane. The electrochemical gradient muscle cells, which have high rates of respiration, is generated by the transport of protons across the membrane contain mitochondria with densely packed cristae. by Complex I, III, and IV in the inner mitochondrial By contrast, liver cells have much lower membrane. matrix to intermembrane space respiration rates and mitochondria with more sparsely distributed cristae. Garett & Grisham: Biochemistry 4th edition Voet, Voet: BIOCHEMISTRY 3rd edition The malate-aspartate shuttle protons of NAPH can go into ematrix IH) [O] Centers ETC - from glycolysis The electrons of cytosolic NADH are transported to mitochondrial NADH in Step 1 to 3. Steps 4 to 6 then serve to regenerate cytosolic oxalacetate. Voet, Voet: BIOCHEMISTRY 3rd edition shuttle The glycerophosphate shuttle alternative to malte - aspartate The electrons of cytosolic NADH are transported to the mitochondrial electron-transport chain in three steps: (1) Cytosolic oxidation of NADH by dihydroxyacetone phosphate catalyzed by glycerol-3-phosphate dehydrogenase. (2) Oxidation of glycerol-3-phosphate by flavoprotein dehydrogenase with the reduction of FAD to FADH2. (3) Reoxidation of FADH2 with the passage of electrons into the electron-transport chain. everyompared lesser to makte aspartate shuttle to Tot IH THI [o] FADH2 -takes over 22- & 24 e-in & enter ETC G3P Voet, Voet: BIOCHEMISTRY 3rd edition ATP-ADP Translocase mediates the movements of ATP and ADP go out goin (A) The bovine ATP-ADP translocase. (B) Outward transport of ATP via the ATP-ADP translocase is favored by the membrane electrochemical potential. The ATP-ADP translocase accounts for 14% of the total mitochondrial membrane protein. ATP/ADP transport occurs via a single nucleotide-binding site, which alternatively faces the matrix and the intermembrane space. It binds ATP on the matrix side, reorients to face the outside, and exchanges ATP for ADP, with subsequent rearrangement to face the matix side of the inner membrane. The charge on ATP at pH 7.2 is about -4, and the charge on ADP at the same pH is about -3. Thus, net exchange of an ATP (out) for an ADP (in) results in the net movement of one negative charge from the matrix to the cytosol. Recall that the inner membrane is positive outside, and it becomes clear that outward movement of ATP is favored over outward ADP transport, ensuring that ATP will be transported out. Thus, the membrane electrochemical potential itself controls the specificity of the ATP-ADP translocase. (A) (B) ~ intermembrane space the charged site binding - 8 App & ATP Link Matrix in allows I this helix movement to occur Garett & Grisham: Biochemistry 4th edition The respiratory chain for oxidative phosphorylation F-type protons from H+ to) of MADH & H+ ATP from synthase H+ FADH2 are pumped H+ matix to Cyt c Protein complex of electron intervenbrave carriers space IV Q I III II 2 H+ + ½ O2 H 2O FADH2 FAD NAD+ [O] real 02 of NADH to] (carrying electrons provide e- (2- acceptor ADP + P i ATP from food) H+ Electron transport chain [trsf e - > Chemiosmosis Oxidative phosphorylation © 2017 Pearson Education, Ltd Concept of the electron transport chain H2 + 1/2 O2 2H + 1 /2 O2 Controlled 2 H+ + 2 e− release of energy stepwise - ATP of Free energy, G Free energy, G release every ATP Explosive release ATP 2 e− 1/ 2 O2 2 H+ accept e- H2O H2O (a) Uncontrolled reaction (b) Cellular respiration © 2017 Pearson Education, Ltd Concept of the electron transport chain NADH (least electronegative) ⚫ The electron transport chain is located in the 50 inner membrane (cristae) of the mitochondrion. 2 e− NAD+ ⚫ Most of the chain’s components are proteins, FADH2 which exist in multiprotein complexes. Free energy (G) relative to O2 (kcal/mol) 2 e− Complexes I-IV FAD ⚫ The carriers alternate reduced and oxidized states I as they accept and donate electrons. 40 FMN II i Fe S Fe S allthese ara e ⚫ Electrons drop in free energy as they go down N [H] Q ~III the chain and are finally passed to O2, forming & release e Cyt b H2O. to Fe S & is Fe S ⚫ Electrons are transferred from NADH or FADH2. 30 [H) & release eto Q & Cyt c1 to the electron transport chain. IV accep a Electrons are passed through a number of release e- Sifth Cyt c ⚫... (ascode) Cyt a proteins including cytochromes (each with an Electron transport 20 chain Cyt a3 acceptors ⚫ iron atom) to O2. The electron transport chain generates no ATP directly. ⚫ It breaks the large free-energy drop from food to release O2 into smaller steps that release energy in 2 e− manageable amounts. 10 for [H) of O2 2 H+ + ½ O 2 0 (most electronegative) H2O © 2017 Pearson Education, Ltd Measurements of redox potentials [H) The half-cell undergoing oxidation (Cu+→Cu2+ + e-) passes the liberated electrons through the wire to the half-cell undergoing reduction (here e- + Fe3+→Fe2+). Electroneutrality in the two half-cells is maintained by the transfer of ions through the electrolyte-containing salt bridge. The voltmeter shows the potential difference DE between the half cells. Any redox reaction can be divided into its component half-reaction, whereby both half-reactions are written as reductions: An+ox + ne- ↔ Ared Bn+ox + ne- ↔ Bred These half-reactions can be assigned reduction potentials EA and EB, in accordance with the Nernst equation and with E° as standard redox potential when all components are in the standard states: EA = E°A – RT/nF ln([Ared]/An+ox]) EB = E°B – RT/nF ln([Ared]/An+ox]) For the redox-reaction of any two half-reactions: DE° = E°(e- acceptor) - E°(e- donor) The free energy of a redox reaction is defined as DG = -n·F·DE (n is the moles of electrons through the electric potential difference DE; F, the faraday, is the electrical charge of 1 mol of electrons (96,494 C/mol). Voet, Voet: BIOCHEMISTRY 3rd edition Biochemical half-reactions reduced ~ The oxidized form of a redox couple with a large positive standard reduction potential has a high affinity for electrons and is a strong electron acceptor (oxidizing agent), whereas its conjugate reductant is a weak electron donor (reducing agent). O2 is a strong oxidizing agent, whereas H2O, which tightly holds its used e-carrier electrons, is a weak reducing agent. The converse is mostly as true of half-reactions with large negative standard for biosynthesis processes canabolic processes reduction potentials. In other words, the more positive the standard reduction potential, the greater the tendency for the redox couple’s oxidized form to accept electrons and thus become reduced. Voet, Voet: BIOCHEMISTRY 3rd edition NADH oxidationis a highly exergonic reaction The half-reactions for O2 oxidation of NADH are: [0 NAD+ + H+ + 2e- ↔ NADH E°’ = -0.315 V 0.5O2 + 2H+ + 2e- ↔ H2O E°’ = 0.815 V > - [H] Since the O 2/H2 O half-reaction has the greater standard reduction potential and therefore the higher electron affinity, the NADH half-reaction is reversed, so that NADH is the electron donor in this couple and O2 the electron acceptor. The overall reaction is 0.5O2 + NADH + H+ ↔ H2O + NAD+ so that DE°’ = 0.815 – (–0.315) = 1.130 V using the equation DG = -n·F·DE DG°’ = –2 (mol e-/mol reactant) x 96,485 (C/mol e-) x 1.13 J·C-1 DG°’ = –218 kJ·mol-1 > mol ofATP (30k]molt) In other words, the oxidation of 1 mol of NADH by O2 (transfer of 2 e-) under standard conditions is associated with the release of 218 kJ of free energy. Quiz: Calculate the free energy change for FADH2 oxidation by oxygen. Voet, Voet: BIOCHEMISTRY 3rd edition The mitochondrial electron-transport chain The standard free energy requires to synthesize 1 mol of ATP from ADP + Pi is 30.5 kJ. The standard free energy of oxidation of NADH by O2 is therefore sufficient to drive the formation of several moles of ATP. This coupling is achieved in the electron-transport chain, in which e- are passed through three protein complexes containing redox Complex I centers with progressively greater affinity for e- (increasing standard reduction potentials) instead of directly to O2. This allows the free Complex II succinate energy change to be broken up into smaller packets, each of which is coupled with ATP synthesis in a process called oxidative phosphorylation. Oxidation of 1 NADH results in the formation of about 3 ATP. The thermodynamic efficiency of oxidative phosphorylation is therefore 3 x 30.5 kJ/mol x 100/218 kJ/mol = 42% Complex III under standard conditions. However, under physiological conditions in active mitochondria, this thermodynamic efficiency is thought to be about 70%. Complex IV pump e-released Karlson, P.: Biochemistry for medicine and science 13th edition The respiratory chain for oxidative phosphorylation moreto H+ ATP H+ H+ ↑ H+ synthase Protein complex Cyt c of electron carriers IV Q & I III II 2 H+ + ½ O2 H 2O FADH2 FAD NADH NAD+ transporte (carrying electrons from 1411 to III ADP + P i ATP from food) pan moves H+ Electron transport chain Chemiosmosis Oxidative phosphorylation © 2017 Pearson Education, Ltd Complex I (NADH dehydrogenase) ⚫ Eukaryotic complex I consists of multi-subunits (app. 45) and has an apparent molecular mass of about 1000 kDa. – However, the bacterial complex contains only 14 “core” subunits. ⚫ It harbors one bound FMN (flavin [H] Lshape ① mononucleotide) and eight iron– format ? sulfur clusters. ⚫ Electrons within complex I are I transferred in multiple steps from Structural organization of mamalian Complex I. NADH to coenzyme Q (CoQ) via 5 FMN and iron–sulfur clusters round e & H Never forget me! Garett & Grisham: Biochemistry 4th edition Voet, Voet: BIOCHEMISTRY 3rd edition Structure of the entire T. thermophilus complex I soluble - region transport have e-stepwise downwards diff reduct pot ? [H) lower iron suffice cluster (reduced) will have ? released red ? pot Crystallographic structure of the archaea Thermus thermophilus complex I (PDB ID: 4hea). Electron transfer chain in the In the hydrophilic peripheral arm, iron-sulfur clusters are shown in blue, and the FMN is green. T. thermophilus complex I. & backwards leading toStructural alterati, moves forward & , drives proton release to intermembrace space © 2019 Pearson Education, Ltd Biochimica et Biophysica Acta – Bioenergetics (2012) Efremov, R.G & Sazanov, L.A. Ubiquinone (coenzyme Q, or CoQ) Oxidation-reduction reaction Cyt c Q IV I III II membrane) hydrophobic (can - cross e-transported along ~ i membrane ⚫ Ubiquinone is a lipophilic electron carrier with a benzoquinone linked to an isoprene-containing tail. ⚫ It can transfer two electrons in one electron steps (via a stable semiquinone intermediate). ⚫ Ubiquinone provides a link between two-electron carriers and one-electron carriers. Never forget me! Voet, Voet: BIOCHEMISTRY 3rd edition Complex II (Succinate:Coenzyme Q Oxidoreductase) Cyt c Q IV I III II ⚫ In addition to accepting electrons from NADH, CoQ can also accept electrons from intermediates in fatty acid oxidation and from succinate. ⚫ Complex II (= succinate dehydrogenase) is an inner membrane multi-subunit protein complex, which is also part of the citric acid cycle, transferring electrons from succinate through FAD and a series of iron– sulfur clusters to CoQ. ⚫ Complex II transfers electrons from succinate through FAD and a series of iron–sulfur clusters to CoQ, but complex II does not pump protons into the intermembrane space. © 2019 Pearson Education, Ltd Complex II (Succinate:Coenzyme Q Oxidoreductase) Cyt c Q IV I III II diff red" potential & Structure of the iron–sulfur 3 forms of iron sulfer clusters clusters [2Fe–2S] & linked to i by cystine resides covalently bonded to thiol grp) Reduction potentials of electron-transport chain components in resting mitochondria Garett & Grisham: Biochemistry 4th edition Voet, Voet: BIOCHEMISTRY 3rd edition © 2019 Pearson Education, Ltd Role of Coenzyme Q in Electron Transport Coenzyme Q collects electrons from complex I, complex II and other flavoproteins for transfer to complex III. e-provided frm FAIO) brings to Q From cytosolic MADH © 2019 Pearson Education, Ltd Complex III (Cytochrome bc1 complex) Cyt c Q IV I III II 2 nerves si low spin ⚫ Complex III catalyzes the transfer of electrons from CoQH2 (reduced coenzyme Q) to cytochrome c in the intermembrane space, and pumps two protons into the IMS for every two electrons transferred. ⚫ Mammalian complex III is about 250 kDa and functions as a dimer with each monomer composed of 10 or 11 protein chains. © 2019 Pearson Education, Ltd Voet, Voet: BIOCHEMISTRY 3rd edition Path of Electrons from CoQH2 to Cytochrome c – the Q Cycle (1 of 2) Complex III 24t come from Matrix ↓ 2nd goes accepted e- unde - is heave b. then to QH & reduces by &reaches it to QU2 , to by& & reducesG to QH (semiquinone) receiving from one proton & take 1 It from matrix matrix Creduced) ste-given to iron - sulfur cluster w moves 2 memb to along 4Ht ↓ complex IV coming out pH gradient generated © 2019 Pearson Education, Ltd Path of Electrons from CoQH2 to Cytochrome c – the Q Cycle (1 of 2) ⚫ During the Q cycle a two-electron donor, CoQH2, is transferring electrons to one- electron acceptors. Ci ⚫ Each complex III monomer has two Q-binding sites. ⚫ This requires that the electrons transferred from CoQH2 take different paths, and in two stages. ⚫ The net process pumps four protons into the intermembrane space. 2 birding © 2019 Pearson Education, Ltd Complex III (Cytochrome bc1 complex) Vinyl group identical in all vings differ in of , - terms Porphyrin rings in cytochromes. Conjugated double-bond Absorption spectrum of reduced cytochrome c showing gups its characteristic a, b, and g (Soret) absorption bands. system is responsible for absorption of visible light. As in Fe-S centers, Fe undergoes oxidation-reduction with one electron. Voet, Voet: BIOCHEMISTRY 3rd edition Cytochrome c Cyt c Q IV I III II Mitochondrial cytochrome c with the heme shown at the center of the structure. It is covalently linked to the protein via two sulfur atoms. A third sulfur from a methionine coordinates the iron. Cytochrome c, like ubiquinone, is a mobile electron carrier. It transports electron from the Fe-S-cyt c1 aggregate of Complex III, and then it migrates along the membrane surface in the reduced state, carrying electrons to cytochrome c oxidase. (complex IV] X-ray structures of cytochrome bc1 Garett & Grisham: Biochemistry 4th edition Voet, Voet: BIOCHEMISTRY 3rd edition Complex IV (Cytochrome c oxidase) Cyt c Q IV I III II highest redo Isoprenoid chain The electron-transfer for cytochrome c oxidase. Cytochrome c binds on the intermembrane space side, transferring electrons through the copper and heme centers to reduce O2 on the matrix side of the membrane. Garett & Grisham: Biochemistry 4th edition Voet, Voet: BIOCHEMISTRY 3rd edition © 2019 Pearson Education, Ltd The electron jump in Complex IV methionise histidine cysteines histidine glutamate intermembrane e- space moves binuclear down unter - matrix - 2 - The redox centers in the X-ray structure of bovine heart cytochrome c oxidase. An electron obtained from cytochrome c is The electron-transfer for cytochrome c oxidase. first acquired by the CuA center and is then transferred to heme a, probably because the shortest CuA ··· heme a distance of 11.7 Å is less than the shortest CuA ··· heme a3 distance of 14.7 Å. The electron is then rapidly transferred to the heme a3-CuB binuclear center, where it participates in reducing the bound O2 to H2O. Garett & Grisham: Biochemistry 4th edition Voet, Voet: BIOCHEMISTRY 3rd edition Coupling of electron transport and ATP synthesis proton motive force H+ is pumped out of the mitochondrial by Complexes I, III and IV of the electron-transport chain, thereby generating an electro- chemical gradient across the inner membrane. The exergonic return of these protons to the matrix powers the synthesis of ATP. Voet, Voet: BIOCHEMISTRY 3rd edition Coffee break Final outcome of electron transfer Cyt c Q IV I III II ⚫ The free energy stored in the proton gradient is called proton-motive force (PMF), which has two components: 1. chemical potential energy due to difference in concentration of protons, which can be expressed as ∆pH (pH difference across the membrane, and 2. electrical potential energy due to separation of charge across the membrane, which can be expressed as ∆ψ, the electrical Complex I - IV potential difference. ⚫ Thus proton motive force is an electrochemical gradient. Karlson, P.: Biochemistry for medicine and science 13th edition The chemiosmotic hypothesis Peter Mitchell postulated in 1961 that the free energy of electron transport is conserved by pumping H+ from the mitochondrial matrix to the intermembrane space so as to create an electrochemical H+ gradient across the inner mitochondrial membrane. The electrochemical potential o f t h e g r a d i e n t i s h a r n e s s e d t o s y n t h e s i z e AT P. pumps protons The reconstitued vesicles containing F1FO ATP synthase and bacteriorhodopsin used by Stoeckenius and Racker to confirm the Mitchel chemiosmotic hypothesis Garett & Grisham: Biochemistry 4th edition Voet, Voet: BIOCHEMISTRY 3rd edition Structure and mechanism of the F-ATP synthase motor X & B are catalytic sites Orange-segment shape read piece One α subunit and one β subunit, from opposite sides of the hexameric ring, and the γ subunit are shown. & can bind 6 nucleotides where c-ring (grey) rotates , it will drive orientati of U && Iblre , violet) © 2017 Pearson Education, Ltd Abrahams, J.P., Leslie, A.G.W., Lutter, R. & Walker, J.E. (1994) Nature 370, 621-628 Structure and mechanism of molecular motors Guo et al. (2019) eLife 2019;8:e43128 doi: 10.7554/eLife.43128 Hausrath, A.C., Grüber, G., Matthews, B.W. & Capaldi, R.A. (1999) PNAS 96, 13697-13702 Binding-Change mechanism in the F1FO ATP synthase (Boyer mechanism) constrate sits asymetrically FEE ⚫ The three αβ dimers of F1 exist in three different conformations, L (loose), T (tight), and O (open). ⚫ The γ subunit rotates counter- clockwise (driven by the passage of protons through FO) while the F1 components are held in a fixed position by the stator ⚫ As the γ subunit rotates, it interacts differently with each subunit and simultaneously causes conformational changes in all three dimers ⚫ These conformational changes allow for the binding of ADP and Pi, the synthesis of ATP, and the release of ATP © 2016 and 2019 Pearson Education, Ltd Regulation through Coupling As ATP chemical bond energy is used by energy- requiring reactions, ADP and Pi concentrations increase. The more ADP is present to bind to the ATP synthase, the greater will be proton flow through the ATP synthase pore, from the intermembrane space to the matrix. Thus, as ADP levels rise, proton influx increases, and the electrochemical gradient decreases The proton pumps of the electron-transport chain respond with increased proton pumping and electron flow to maintain the electrochemical gradient. The result is increased (substrate) O2 consumption. The increased oxidation of NADH in the electron-transport chain and the increased concentration of ADP stimulate the pathways of fuel oxidation, such as the TCA cycle, to supply more NADH and FAD(2H) to the electron-transport chain. For example, during exercise we use more ATP for muscle contraction, consume more oxygen, oxidize more fuel, and generate more heat from the electron-transport The concentration of ADP (or the phosphate potential, chain. If we rest, and the rate of ATP use decreases, [ATP]/[ADP][Pi]) controls the rate of oxygen consumption proton influx decreases, the electrochemical gradient increases, and proton “back pressure” decreases the rate of the electron-transport chain. NADH and FAD(2H) cannot be oxidized as rapidly in the electron-transport chain, and consequently, their buildup inhibits the enzymes that generate them. C. M. Smith, A. D. Marks & M. A. Liebermann, Marks’ Basic Medical Biochemistry: A Clinical Approach, 7th edition ATP yield per molecule of glucose at each stage of cellular respiration CYTOSOL Electron shuttles MITOCHONDRION span membrane 2 NADH or 2 FADH2 2 NADH 2 NADH 6 NADH 2 FADH2 GLYCOLYSIS PYRUVATE OXIDATION OXIDATIVE CITRIC PHOSPHORYLATION ACID Glucose 2 Pyruvate 2 Acetyl CoA CYCLE (Electron transport and chemiosmosis) + 2 ATP + 2 ATP + about 26 or 28 ATP Maximum per glucose: About 30 or 32 ATP © 2017 Pearson Education, Ltd Uncoupling of oxidative phosphorylation Uncouplers act by dissipating the proton gradient across the inner mitochondrial membrane created by the electron-transport system. These compounds share two common features: hydrophobic character and a dissociable proton. Their tendency is to aquire protons on the cytosolic surface of the membrane and carry bind them to the matrix side, thereby proton destroying the proton gradient that ~ hydrophobia couples electron transport and the character ATP synthase. In mitochondria - treated with uncouplers, electron transport continues and protons are I driven out through the inner & release membrane. However, they leak back proton in so rapidly via the uncouplers that easily ATP synthesis does not occur. Instead, the energy released in electron bind transport is dissipated as I proton heat. -hydrophobic across e memb Voet, Voet: BIOCHEMISTRY 3rd edition Brown fat story ⚫ Most newborn mammals, including humans, have a type of adipose tissue called brown fat. ⚫ These cells contain large number of mitochondria (brown color is due to cytochromes in mitochondria) which contain, in the inner membranes, a protein called thermogenin (also called uncoupling protein, UCP). In cold-adapted animals, thermogenin constitutes up to 15% of brown fat inner mitochondrial membrane proteins. ⚫ UCP dissipates the proton gradient by allowing the protons to return to the matrix. The free energy of the gradient is dissipated as heat. This helps to maintain the body temp of newborn mammals that lack fur, as well as hibernating mammals. ⚫ This is a type of nonshivering thermogenesis. ⚫ Skunk cabhage and related plants contain floral spikes that are maintained as much as 20°C above ambient temperature in this way. The warmth of the spikes serves to vaporize odiferous molecules, which attract insects that fertilize the flowers. Garett & Grisham: Biochemistry 4th edition Uncoupling of oxidative phosphorylation in brown fat mitochondria (1) Norepinephrine (Noradrenaline) binds to i t s c e l l s u r f a c e r e c e p t o r. ( 2 ) T h e norepinephrine-receptor complex stimulates adenylate cyclase, thereby causing cAMP levels to rise. (3) cAMP binding activates protein kinase A (PKA). (4) PKA phosphor ylates hormone-sensitive triacylglycerol lipase, thereby activating it. (5) Triacylglycerols are hydrolyzed, yielding free fatty acids. (6) Free fatty acids overcome the purine nucleotide block of the H+ channel formed by thermogenin, allowing H+ to enter the mitochondrion uncoupled from ATP synthesis. Voet, Voet: BIOCHEMISTRY 3rd edition The Versatility of Catabolism Proteins Carbohydrates Fats Amino Sugars Glycerol Fatty acids acids GLYCOLYSIS Glucose Glyceraldehyde 3- P NH3 Pyruvate Acetyl CoA CITRIC OXIDATIVE ACID PHOSPHORYLATION CYCLE Quiz 1. Which of the following components of the respiratory chain complexes undergo a one or two electron step? FMN, Ubiquinone, CuA, CuB, heme b, heme a, heme a3 ⚫ Two electron steps: FMN, ubiquinone ⚫ One electron step: CuA, CuB, heme b, heme a, heme a3 2. Which of the four complexes (I-IV) do(es) not pump protons from the matrix to the intermembrane space? Complex II Quiz 3. Call the two components of the proton-motive force (PMF). 1. chemical potential energy due to difference in concentration of H+, which can be expressed as ∆pH (pH difference across the membrane, and 2. electrical potential energy due to separation of charge across the membrane, which can be expressed as ∆ψ, the electrical potential difference. Thank you!