Lecture 24 Lipolysis and B oxidation_handout.pdf
Document Details
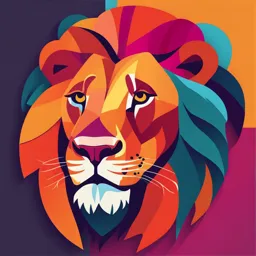
Uploaded by WieldyEpic
Tags
Full Transcript
PBBS508 Biochemistry & Genetics L24: Lipolysis and b Oxidation Asst. Prof. Witchuda Saengsawang, Ph.D. Learning Objectives VC1 Describe the role that hormone sensitive lipase plays in release of fatty acids from their st...
PBBS508 Biochemistry & Genetics L24: Lipolysis and b Oxidation Asst. Prof. Witchuda Saengsawang, Ph.D. Learning Objectives VC1 Describe the role that hormone sensitive lipase plays in release of fatty acids from their stores. VC2 Describe in detail the activation of fatty acids and the carnitine shuttle VC3 List the four major steps for each round of fatty acid catabolism. VC4 Describe the clinical outcome of a medium chain acyl-CoA dehydrogenase deficiency. VC5 Describe odd-chain fatty acid catabolism and its relationship to succinyl CoA VC5 Define the role of peroxisomes in fatty acid catabolism. VC6 Describe alpha oxidation of branch chain fatty acids and Rfsums disease. VC8 Describe ketone body synthesis. VC9 Describe ketone body utilization and rationalize why the liver does not use ketone bodies for its own energy generation. Lipolysis & b Oxidation Fatty acids stored in adipose tissue in the form of triglycerides (TAGs) Triacylglycerol (TAGs) Serve as the body’s major fuel source à broken down to release the stored energy. Lipolysis: break down TAGs into glycerol and fatty acids Lipolysis Glycerol b-oxidation: break down Fatty acids into Acetyl CoA Fatty acids b-oxidation b-oxidation Acetyl CoA Lipolysis Ketogenesis Glycerol is released into the blood and goes to the liver where it is phosphorylated à glycerol phosphate Glycerol phosphate can either be used to form new TAGs in the liver, or can be converted to DHAP and enter gluconeogenesis Lipolysis When? : Starvation / Hypoglycemia Where? : adipose tissues *** Adipocytes: Hormone-Sensitive Lipase (HSL) breaks down TAGs into Glycerol and Fatty Acids Glucagon, Epinephrine and cortisol activate HSL *** Insulin inhibits HSL*** Glucagon Epinephrine Cortisol Insulin b Oxidation Break down / Oxidation of Fatty Acid (FA) When? : Starvation / Hypoglycemia Where?: Occurs in mitochondria (matrix) in several tissues, muscles, liver, heart, adipose tissues *Erythrocytes (no mitochondria) and the brain (blood brain barrier exclusion) cannot use fatty acids for energy. How can FA get into mitochondrial matrix ?*** Short-chain FA (20 carbons) enter peroxisomes (unknown mechanism) Key substrate: Fatty acids (Fatty acyl-CoA) Key products: Acetyl CoA, FADH2 , NADH Summary of b Oxidation Rate-limiting enzyme* for b Oxidation is Carnitine acyltransferase-1, which is in the process of “carnitine shuttle” The carnitine shuttle is a system that moves fatty acids into the mitochondria Fatty acyl-CoA dehydrogenase catalyzes the first step of beta-oxidation (chain length specific) Summary of palmitate b Oxidation 7 FAD + 7 NAD+ + 7 CoA + 7 H2O + H(CH2CH2)7CH2CO-SCoA 8 Acetyl CoA + 7 FADH2 + 7 NADH + 7 H+ Enter Krebs -> ETC -> ox-phos to produce ATP. ETC Oxidized in ……………, yielding ATP. Pyruvate carboxylase In liver: stimulate gluconeogenesis by activating enzyme……………………………… may be used for ketone body synthesis in liver FA entry into mitochondria 1. Fatty acyl synthetase attachs fatty acid to CoA à FA-CoA FA-CoA 2. Carnitine acyltransferase-1*** (rate-limiting enzyme) transfers FA-CoA to carnitine à FA-carnitine Rate limiting enzyme Insulin à + ACC à Malonyl-CoA - *** Carnitine or Carnitine palmitoyl (insulin indirectly inhibit CAT1) Or Carnitine palmitoyl transferase-2 (CPT2) transferase-1 (CPT1)*** 4. Carnitine acyltransferase-2 transfer FA-Carnitine back to FA-CoA 3. FA-Carnitine is transported across the inner membrane of mitochondria b Oxidation in mitochondria Reverse process of FA synthesis FA synthesis b Oxidation :Reducing :Oxidizing :Add acetyl-CoA :Release acytyl-CoA Repetetion of 4 steps Each 4-steps cycle : Releases 1 Acetyl-CoA (2C) : Oxidizing (reduces NAD+ and FAD) : Producing 1 NADH and 1 FADH2 Carnitine Repeat: total 7 rounds (for palmitate) Regulations********************** At least 4 Fatty acyl-CoA dehydrogenase ATP Availability of substrates and cofactors Each is specific for a different acyl chain length Involved in different stages of beta-oxidation. Product: rate of processing acetyl CoA in VLCAD: C21 and longer. the TCA cycle. LCAD: chains of C13-C20 *Malonyl CoA (and *insulin) inhibits CPT1 MCAD: chains of C6 to C12 SCAD: chains shorter than C6 Energy yield from b Oxidation for palmitate 4-steps cycle 1. Oxidation 2. Hydration 3. Oxidation 4. Cleavage 7 FAD + 7 NAD+ + 7 CoA + 7 H2O + H(CH2CH2)7CH2CO-SCoA a 8 Acetyl CoA + 7 FADH2 + 7 NADH + 7 H+ Important differences between synthesis and degradation of fatty acids Read this please Summary of Fatty synthesis and degradation b Oxidation of odd chain FA Oxidation of odd-chain fatty acids produces Propionyl-CoA The product of oxidation of odd chain fatty acids is propionyl CoA (instead of Acetyl CoA) Propionyl CoA is converted to Methylmalonyl CoA (intermediate) and Succinyl CoA (final product). Succinyl CoA enters TCA cycle Conversion of Propionyl CoA to Methylmalonyl CoA needs Biotoin Conversion of Methylmalonyl CoA to Succinyl CoA needs Coenzyme form of vitamin B12 In Vit B12 deficiency -> Methylmalonic aciduria TCA cycle Lippincott Fig. 16.20 Ketone body metabolism Ketone bodies*** During prolonged starvation 1. Acetoacetate 2. β-hydroxybutyrate (3-hydroxybutyrate) Liver converts excess Acetyl-CoA from b Oxidation into ketone bodies (ketogenesis) 3. Acetone (expired by lung) Ketone bodies are metabolized to Acetyl-CoA (ketolysis) and used by extrahepatic tissues*. (Fruity odor breath) Succinyl-CoA acetoacetyl- CoA transferase HMG-CoA synthase (Only expressed in liver) (Thiophorase)*** (Not expressed in liver) Ketone body metabolism Rate limiting step*** Ketogenesis Liver converts excess Acetyl-CoA produced from Only expressed in liver*** b Oxidation into ketone bodies Ketogenesis HMG-CoA synthase* is the rate limiting enzyme* HMG-CoA synthase (mito) is the expressed only in liver ***Therefore only liver can make ketone bodies Ketolysis Ketone bodies are used by extrahepatic tissues (not liver) Thiophorase is NOT expressed in liver ***Therefore Liver CANNOT use ketone bodies Cardiac & Skeletal Muscle Metabolizes ketones as soon as the liver releases them Prevent accumulation in blood Ketolysis Succinyl-CoA (Extrahepatic) Preserve the limited glucose for use by the brain and other acetoacetyl-CoA obligate tissues eg. RBC & cells with limited mitochondria transferase (Thiophorase) Only expressed in extrahepatic tissues*** Brain* begins to metabolizing ketone bodies after ~a week of fasting FA cannot pass BBB Note**: Brain cannot use fatty acid because ………………………… Excessive Ketone Body Production If Ketogenesis is higher than Ketolysis à ketone bodies rise in the blood (ketonemia) and urine (ketonuria) These 2 conditions are usually seen in uncontrolled type 1 diabetes (insulin dependent) (Diabetic ketoacidosis) Low insulin and high glucagon will increase lipolysis and increase free fatty acid in plasma High fatty acid degradation produces excessive Acetyl CoA --> depletes the NAD+ pool and slows down the TCA cycle This forces the excessive Acetyl CoA into ketogenesis A frequent symptom of diabetic ketoacidosis is a fruity odor breath (acetone) Acidemia as each ketone body will loose a proton as it circulates through the blood. Summary Lipogenesis and TAG synthesis Lipolysis Beta Oxidation Ketogeneis Ketolysis Fatty acid synthase Energy yield from b Oxidation for palmitate 4-steps cycle 1. Oxidation LCAD 2. Hydration X MCAD 3. Oxidation SCAD 4. Cleavage 7 FAD + 7 NAD+ + 7 CoA + 7 H2O + H(CH2CH2)7CH2CO-SCoA a 8 Acetyl CoA + 7 FADH2 + 7 NADH + 7 H+ Deficiencies of carnitine shuttle à Decreased ability of tissues to use Long-Chain Fatty Acid (LCFA) as a fuel LCFA need to be transported into mitochondrial matrix by carnitine shuttle Primary carnitine deficiency Defects in a membrane transporter Cannot uptake carnitine by cardiac and skeletal muscle and the kidneys à Carnitine is excreted. Treatment: carnitine supplementation. Secondary carnitine deficiency Occurs primarily as a result of defects in fatty acid oxidation Leading to the accumulation of acylcarnitines that are excreted in the urine, decreasing carnitine availability (cannot be recycled). Acquired secondary carnitine deficiency Patients with liver disease (decreased carnitine synthesis) Patients taking the antiseizure drug valproic acid (decreased renal reabsorption). CPT-I deficiency (affects liver) Cannot use LCFA à impair ATP à impair glucneogenesis (an endergonic process) Severe fasting hypoglycemia, coma, and death. CPT-II deficiency (defect in muscle specific CPT-II) The most common (and least severe) form affects skeletal muscle. Muscle ache, weakness with rhabdomyolysis -> myoglobinemia, myoglobinuria (red urine) following prolonged exercise (after fasting). Treatment à avoid fasting à adopting a diet high in carbohydrates and low in fat (supplemented with medium-chain TAGs) Medium-Chain fatty Acyl CoA Dehydrogenase (MCAD) deficiency***: An autosomal-recessive disorder Most common inborn error of β-oxidation, being found in 1:14,000 births worldwide Results in decreased ability to oxidize medium-chain fatty acids (accumulation in blood and urine) Low to absent ketones (because production of Acetyl CoA is decreased) Hypoketonic hypoglycemia Severe fasting hypoglycemia (lack of ATP to support gluconeogenesis) Lethargy, coma, death if untreated Treatment: avoide prolonged fasting Some cases of Sudden infant death syndrome (SIDS) are due to MCAD deficiency Fatty acid oxidation in Peroxisomes b-Oxidation of Very-Long Chain Fatty Acids (VLCFA) Very-long chain fatty acids (VLCFA) (C21 or longer) must go to the peroxisomes for preliminary β-oxidation (chain shortening). Then shortened FA (linked with carnitine) then diffuse to mitochondria for further oxidation Genetic diseases associated with VLCFA oxidation: Zellweger syndrome – a defect in peroxisomal assembly in all tissues Accumulation of VLCFA in the blood and tissues Neuropathy is a major consequence Adrenoleukodystrophy – X-linked, defect in peroxisomal activation of VLCFA (brain, liver and kidneys) a-Oxidation of Branched-Chain Fatty Acids (phytanic acid -> most common) Branched-chain fatty acids are catabolized by a-Oxidation Phytanic acids are produced in animal that consume plants, algae. -> Human ingest red meat, dairy products and fatty marine foods Refsum’s disease*** A rare, inherited disorder in which patients lack the a-oxidizing enzyme. Accumulate large quantities of phytanic acid in tissues and serum. Leads to severe symptoms including: cerebellar ataxia, retinitis pigmentosa, nerve deafness and peripheral neuropathy Skeletal dysplasia may be seen (shortening of bones in the hands and feet and abnormal growth plate formation) Summary 1. When and where does lipolysis occur? What is key enzyme used? and how is it regulated? 2. What are key steps for beta oxidation? (location? substrates, translocation and activation? products? 3. What is beta oxidation rate limiting enzyme? regulations? 4. What are the roles of peroxisome in lipid metabolism? what is Refsum disease? 5. What are ketone bodies? How are they synthesized? (which tissues? location? substrate? key enzymes?) 6. What tissues can/cannot use ketone bodies? why?