Enzyme Regulation Lecture Notes PDF
Document Details
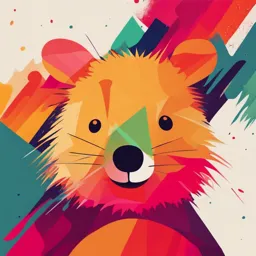
Uploaded by LaudableAgate8378
University of Virginia
Reginald H. Garrett & Charles M. Grisham
Tags
Summary
This document provides a comprehensive overview of enzyme regulation, covering various mechanisms like covalent modification, zymogen activation, and allosteric regulation. It highlights the roles these mechanisms play in controlling enzyme activity in biological systems, using diagrams where appropriate. It provides detailed explanations of important models such as the MWC and KNF models, and also discusses examples such as protein phosphorylation, acetylation and proenzyme activation within the context of enzyme regulation.
Full Transcript
Reginald H. Garrett Charles M. Grisham www.cengage.com/chemistry/garrett Chapter 15 Enzyme Regulation Reginald Garrett & Charles Grisham University of Virginia Lecture 20 – Study Guide Understand the different mechanisms by whic...
Reginald H. Garrett Charles M. Grisham www.cengage.com/chemistry/garrett Chapter 15 Enzyme Regulation Reginald Garrett & Charles Grisham University of Virginia Lecture 20 – Study Guide Understand the different mechanisms by which enzyme activity can be regulated Know examples for each type of enzyme regulation mechanism Understand how regulatory enzymes typically show sigmoidal kinetics, indicative of cooperative substrate binding Understand how allosteric effectors act and that they bind to sites distinct from the substrate binding sites Know that allosteric enzymes are typically oligomers Understand how the MWC model explains cooperativity in substrate binding and allosteric regulation Understand how the KNF model explains cooperativity in substrate binding and allosteric regulation Know the key features that distinguish the two models Understand that these models are not absolute – different enzymes have features that are consistent (to a greater or lesser extent) with one or other model, or with both Enzyme Regulation Enzymatic reactions (thus products) are regulated to match cell requirements This is achieved by regulating enzyme: 1) Abundance 2) Activity Abundance is controlled by gene expression (not treated) We will focus on regulation of enzyme activity Note that enzyme regulation is a natural physiological phenomenon. Enzymatic reaction velocity The velocity of a reaction is typically controlled by the concentrations of substrates and cofactors (cofactors: metal ions or organic coenzymes that participate in some enzyme reactions) E + S ↔ ES→ E + P Enzyme Regulation Accumulation of product reduces velocity of reaction For conversion of substrate, S, to product, P, reaction velocity v is given by d[P]/dt Keq = [P]/[S] Once the ratio of [P]/[S] approaches Keq, no further reaction is apparent, because of the increased rate of the reverse reaction Some enzymes may also be allosterically regulated by their product Enzyme Regulation Enzyme activity can be regulated in different ways: 1) Covalent modification 2) Zymogen (proenzyme) activation 3) Isozymes 4) Control by modulatory proteins 5) Allosterically Enzyme Regulation – covalent modification Covalent modification of an amino acid side chain can activate or inactivate an enzyme (e.g. : Serine, Threonine, Tyrosine, Aspartate) Example: Protein Phosphorylation The enzymes that introduce and remove modifications can be regulated (e.g. by allosteric control or by covalent modification) By controlling the ratios between inactive /active enzymes the generation of products can be controlled Enzyme Regulation – covalent phosphorylation Reversible phosphorylation: the most prominent form of covalent modification in cellular regulation Phosphorylation is accomplished by protein kinases Each protein kinase targets specific proteins for phosphorylation Phosphoprotein phosphatases catalyze the reverse reaction – removing phosphoryl groups Kinases and phosphatases themselves are targets of regulation Other Covalent Modification that Regulates Protein Function Several different chemical modifications of proteins have been discovered Only a few are used to achieve metabolic regulation through reversible conversion of an enzyme between active and inactive forms Covalent Modification - acetylation Acetylation is a prominent modification for the regulation of metabolic enzymes Acetylation of an ε-NH3+ group on a Lys residue changes it from a positively charged amino group to a neutral amide This change may have consequences for protein structure and thus function The acetylating enzyme is termed an acetyl-CoA-dependent lysine acetyltransferase or KAT (More than 30 KATs are known in mammals) Deacetylation by KDACs (lysine deacetylases) reverse the effects Acetylation of metabolic enzymes is an important mechanism for regulating the flow of metabolic substrates (e.g: carbohydrates and fats) Activation of malate dehydrogenase Enzyme Regulation – Proenzymes Synthesis of enzyme as an inactive precursor Activation requires specific proteolytic cleavage The hormone insulin (responsible for glucose uptake) is made as an inactive 86 amino acid precursor (proinsulin). Proteolytic removal of residues 31-65 generates the active form, (two chains and three disulfide bonds) Proenzyme activation by protease cleavage is irreversible Enzyme Regulation – Proenzymes of the digestive tract Enzyme Regulation – Proenzymes of the digestive tract Enzyme regulation - Isozymes Enzymes with slightly different subunits Structurally equivalent subunits (small primary sequence differences not identical) but catalytically distinct subunits Enzyme regulation – Binding of regulatory proteins The catalytic (C) subunit of cAMP-dependent protein kinase is kept in an inactive form by the regulatory (R) subunit. Binding of c-AMP to the R subunit releases the active enzyme. Allosteric regulation Activation or inhibition of enzyme activity by small molecules (metabolites) that interact non-covalently with the enzyme The allosteric effector binds to a site other than the substrate binding site (allo = other) Reversible binding of an effector to the enzyme allows for very rapid response times and thus rapid control of enzymatic activity Allosteric regulation E1 E2 E3 E4 E5 A B C D E F Example: the product (F) as an allosteric inhibitor of the first enzyme of the pathway (E1). This is called feedback inhibition E1 is called regulatory enzyme (next slide) : Where activity of the metabolic pathway is regulated Allosteric activation is also common. Allosteric regulation – regulatory enzymes Regulatory enzymes are often subject to allosteric regulation Properties Kinetic does not follow the Michaelis-Menten equation 1. Sigmoid/S-shaped kinetics 2. Often show Cooperativity: Binding of S make binding of other S easier to same molecules 3. Cooperative substrate binding is a special case of allostery 4. Regulatory enzymes are oligomeric (it follows from cooperative kinetics, more than one substrate binding site) Allosteric regulation – regulatory enzymes Properties: Inhibition by feedback allosteric inhibitor does not conform to normal inhibition patterns Allosteric effector (inhibitor or activator) usually has little/no structural similarity to the substrate Effector binds at a site remote from the substrate binding site Regulatory effects alters distribution of enzyme distribution and conformational changes occurring in the enzyme as a result of effector binding Symmetry model for allosteric regulation: the Monod- Wyman-Changeux (MWC) model Allosteric proteins can exist in two states: R (relaxed) and T (taut) In this model, all the subunits of an oligomer must be in the same state T state predominates in the absence of substrate S S binds much tighter to R than to T In the absence of ligand, the two states are called R0 and T0 The equilibrium constant (L) for the T0/R0 equilibrium is large, that is T0 predominates Symmetry model for allosteric regulation: the Monod- Wyman-Changeux (MWC) model The substrate affinity of each state of the enzyme is described by a dissociation constant: KR (for the relaxed form) and KT (for the tense form) KT is much greater than KR. That is, R0 has a higher affinity for the substrate than T0 The substrate binds with higher affinity to the less abundant form of the enzyme The Monod-Wyman-Changeux (MWC) model : cooperativity T0 Substrate binds preferentially to R0. The substrate bound form is R1 Binding lowers the abundance of R0 Equilibrium between T0 to R0 conformations is driven to R0 R has >1 substrate binding sites Substrate binding increases the concentration of R (i.e. R1 + R0) Therefore, the amount (number) of substrate binding sites increases : positive cooperativity The Monod-Wyman-Changeux (MWC) model : cooperativity Y = fractional occupancy of substrate binding sites n = number of subunits L = T0/R0 c = KR/KT The degree of cooperativity depends on L, KR and KT The Monod-Wyman-Changeux (MWC) model : allosteric regulation Activator : positive effector Substrate (S) and activator (A) bind preferentially to the R form T0/R0 equilibrium is shifted towards R0 Number of S binding sites increases (without any addition of S) L is decreased Increase the affinity of S binding and reduce cooperativity Quiz 2 In the presence of a positive effector the YS/[S] curve will: A - Shift to the right B - Shift to the left C - Shift vertically D – None of the above The Monod-Wyman-Changeux (MWC) model : allosteric regulation Activator : positive effector Substrate (S) and activator (A) bind preferentially to the R form T0/R0 equilibrium is shifted towards R0 Number of S binding sites increases (without any addition of S) L is decreased Increase the affinity of S binding and reduce cooperativity The Monod-Wyman-Changeux (MWC) model : allosteric regulation Inhibitor : negative effector Inhibitor (I) binds preferentially to the T form T0/R0 equilibrium is shifted towards T0 Number of S binding sites decreases (L is increased) Decrease the affinity of S binding and increase cooperativity The Monod-Wyman-Changeux (MWC) model: allosteric regulation – K systems and V systems ‘K systems’: the concentration of substrate that gives half-maximal substrate binding (K0.5) changes in the presence of A and I. Vmax does not change In ‘V systems’, allosteric effectors change the Vmax and K0.5 remains the same R and T forms of the enzyme have the same affinities for S, but differ in their affinities for A and I and in their catalytic properties This regulation is important when the cellular concentration of S is much greater that K0.5 The Monod-Wyman-Changeux (MWC) model : allosteric regulation In the case of positive cooperativity, the substrate is acting as a positive effector, in this case called a positive homotropic effector A ligand other than the substrate that activates the binding of substrate is called a positive heterotropic effector or allosteric activator A ligand that Inhibit the binding of substrate is called a negative heterotropic effector or allosteric inhibitor The MWC model cannot explain negative cooperativity in substrate binding The Koshland-Nemethy-Filmer (KNF) model : allosteric regulation There is no equilibrium between different conformers of the enzyme with no S S binding causes a conformational change, and the subunits can adopt different conformations (unlike in MWC) S binding causes the other subunit to undergo a conformational change, to a form that has a higher or lower affinity for S. The model can explain both negative and positive cooperativity Allosteric activator works in the same way as S, but by binding to a different site Inhibitor works by preventing the conformational change induced by S binding Key differences between the MWC and KNF models cooperativity T R S S S In MWC, there is a pre-exisiting equilibrium between R and T forms, in the absence of ligand. Substrate or activator binds preferentially to the R form All subunits must be in the same conformation, there are only 2 possible conformations All subunits change conformation together, therefore mechanism is called the concerted or symmetry model S S S S In KNF, conformational change is induced by ligand binding Conformational change can be transmitted to a neighboring subunit Intermediate conformations are possible Subunits can be in different conformations and change sequentially, so mechanism is called sequential model