Lecture 2 (Clinical Buffering) PDF
Document Details
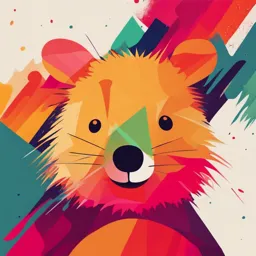
Uploaded by FriendlyTrust
UKZN
Dr S Naidoo
Tags
Summary
This document provides lecture notes on clinical buffering, covering topics such as blood buffering, thermodynamics, and related concepts. It's likely part of a larger curriculum related to medical/biological science.
Full Transcript
PHRM246 Clinical Buffering UKZN/PHRM246/Biochem/Dr S Naidoo in brief… Clinical buffering capacity n blood & tissues The Bohr Effect Transport of O2 & CO2, and role of haemoglobin Clinical consequences of inadequate buffering Regulation of acid-base balanc...
PHRM246 Clinical Buffering UKZN/PHRM246/Biochem/Dr S Naidoo in brief… Clinical buffering capacity n blood & tissues The Bohr Effect Transport of O2 & CO2, and role of haemoglobin Clinical consequences of inadequate buffering Regulation of acid-base balance Thermodynamics Buffering Ability of solutions to maintain a pH and to resist changes to this pH even upon addition of acid or base components pH remains relatively stable, by neutralising the added acid or base. This is important for processes and/or reactions which require specific and stable pH ranges Buffer solutions have a working pH range and capacity - dictates how much acid/base can be neutralized before pH changes, and the amount by which it will change Clinical Buffering Capacity Most biological reactions & enzymes require specific pH range to work optimally. Blood pH - very narrow range for normal homeostasis (7.35 – 7.45) Severe metabolic consequences 7.8 Buffering in Blood Human blood plasma contains a buffer of carbonic acid (H2CO3) and bicarbonate anion (HCO3-) hydronium and bicarbonate anion are in equilibrium with carbonic acid CO2 + H2O H2CO3 H2CO3 H+ + HCO3- 2 equilibrium systems: (i) Carbonic acid can decompose into CO2 (g) and water, resulting in (ii) equilibrium system between carbonic acid and water. CO2 (& O2) are important components that have to be regulated in blood buffer Buffering in Blood continued… Human RBC - equilibrium between hydronium and oxygen - involves the binding ability of haemoglobin Increase in hydronium causes this equilibrium to shift towards the oxygen side Oxygen from hemoglobin molecules released into surrounding tissues/cells This system continues during exercise, providing continuous oxygen to working tissues CO2 + H2O H2CO3 H2CO3 H+ + HCO3- carbonic anhydrase CO2 + H2O H2CO3 H+ + HCO3- weak weak acid conj. base (controlled by lungs) (controlled by kidneys) pH = - Log [H+] 0.03 pCO2 pKa 3.8 CO2 (g) + H2O H2CO3 H+ + HCO3- Henderson-Hasselbach equation pH = pKa + Log [HA] [A-] pH = 6.1 + Log [HCO3-] (0.03)(pCO2) Bohr Effect The Bohr effect is the reversible shift in Hb affinity for O2 with changes in pH Pressure difference between CO2 in peripheral tissues and circulation Diffusion of CO2 from the tissues into blood vessels CO2 + H2O H2CO3 H+ + HCO3- In RBC, CO2 is in equilibrium with carbonic acid and the ionisation into H+ (buffered by Hb) H+ ions compete with O2 for Hb (HbO2) and results in release of O2 into the tissues CO2 Transport (from tissue to lungs) DISSOLVED CO2 Carbon dioxide is much more soluble in blood than oxygen About 5 % of CO2 is transported unchanged, simply dissolved in the plasma BOUND TO HAEMOGLOBIN AND PLASMA PROTEINS CO2 combines reversibly with Hb to form carbamino-haemoglobin/ haemoglobin carbamate. CO2 does not bind to iron, as oxygen does, but to amino groups on the polypeptide chains of Hb CO2 also binds to amino groups on the polypeptide chains of plasma proteins About 10 % of CO2 is transported bound to Hb & plasma proteins BICARBONATE IONS (HCO3- ) The majority of CO2 is transported in this way CO2 enters RBCs in tissue capillaries, combines with H2O to form H2CO3 , catalysed by carbonic anhydrase (found in RBCs) H2CO3 then dissociates to form HCO3- & H+ Tissue Plasma Red Blood Cells Lung (circulation) Alveoli HbO2 Partial pressure differential O2 O2 H+ HbH+ HbCO2 HCO3- + H+ CO2 Chloride shift Cl- HbCO2- H2CO3 Hb CO2 CO2 + H2O Partial pressure differential HEMOGLOBULIN MOVES CO2 & O2 http://www.youtube.com/watch?v=QP8ImP6NCk8 Clinical consequences of inadequate buffering Metabolic (renal) and pulmonary acidosis and alkalosis Can be fatal Dysfunctional physiological state with an excess accumulation of acidic or basic compounds May occur by metabolic defect or ingestion 4 important conditions that are associated with blood buffering Respiratory Acidosis Too much CO2 in the lungs Hypoventilation (Hypoxia) Rapid, shallow respiration Lungs retaining too much BP decreases with vasodilation CO2, therefore pCO2 Dyspnoea increases more than Headache 45mmHg Hyperkalaemia Too much weak conjugate Dysrhythmia base leads to increase in H+, Drowsiness, dizziness, disorientation thereby decreasing pH Muscle weakness, hyper-reflexia pH goes below 7.35 Possible causes: decrease in respiratory stimuli (anaesthesia, drug overdose), COPD, Pneumonia, Atelectasis Respiratory Alkalosis Lungs remove too much CO2 Seizures Deep, rapid breathing pCO2 decreases more than Hyperventilation 35mmHg Tachycardia Reduced amount of acid will Decrease or normal BP result in reduction of [H+] Hypokalaemia Numbness, tingling of extremities pH will go up thereby Lethargy & confusion creating an alkaline condition Light headedness pH goes above 7.45 Nausea & vomiting Possible causes: Hyperventilation (anxiety, fear), mechanical ventilation Ampholytes, Polyampholytes, pI & Zwitterion Molecules that contain both acidic & basic groups within same structure capable of both accepting and losing protons Proteins with aa that have ionizable acidic/basic side groups pI (isoelectric point): pH of protein at 0 net charge Able to predict charge of an aa at a certain pH (characteristics) 1 7 14 Proton acceptor proton donor Isoelectric point Nett positive charge Nett negative charge isoelectric point at zero charge pI = [(pKa (COO-) + pKa (NH3+)] 2 Represents the pH of solution - glycine at net charge is zero Can also be used to calculate the charge of the molecule at physiological pH High pI - more acidic groups & lower pI - more basic groups in the protein Solvation & Hydration Shells Water - central role in proteins and nucleic acids thermodynamics & structure Biological activity - narrow temp, solvent potential & ionic conc. range Cellular functions are driven by solvent environment (incl. pH & ionisation, & solute concentrations) Solvation: propensity of water molecules to arranged around objects in water Solvation & Hydration Shells continued… Addition of ion in water – structure of water bond network changes Water molecules re-orientate Re-orientation to face ions break hydrogen bonds to their nearest water neighbours The group of water molecules oriented around an ion is called a hydration shell Solvation & Hydration Shells continued… Hydration shell results in a net charge on the outside = same charge as ion in the centre Charge on the outside of the hydration shell – orientates other water molecules - second hydration shell Hydration shells weaken hydrogen bond network Salt water has a lower freezing point than pure water: each salt ion uses water molecules & reduces those available for crystalline ice formation Solvation & Hydration Shells continued… Proteins exhibit a net positive or negative charge in solution (pH, charged functional (R) groups) Poly-electrolytes (e.g. DNA and RNA) and poly-ampholytes together in solution lead to electrostatic interaction Smaller charged ions (e.g. Na+, Cl-, Mg2+, Mn2+, K+) interact on multiple levels with larger macro-ions to create electrostatic shields between like-charged molecules Allows macro-ions to form larger associations than normal Therefore, smaller ions maintain solubility of macromolecules at their isoelectric pH High concentration of small ions diminishes solvation of proteins in solution (macro-molecules and water) because the water molecules now form hydrations shells around these small ions Renal Regulation of Acid-Base Balance The kidneys control pH by adjusting the amount of HCO3− that is excreted or reabsorbed All of the HCO3− in serum is filtered as it passes through the glomerulus Reabsorption of HCO3− is equivalent to removing free H+ Changes in renal acid-base handling may occur from hours to days after changes in acid-base status Sodium Bicarbonate Reabsorption Plasma acid-base balance occurs through renal control of HCO3- reabsorption and secretion of H+ HCO3− reabsorption occurs mostly in the proximal tubule and, to a lesser degree, in the collecting tubule The generation of HCO3- and H+ occurs by dissociation of carbonic acid (H2CO3), formed in the tubule cells from H2O and CO2, through the action of carbonic anhydrase. Secretion of H+ into the lumen occurs as ion exchange for Na+. Na+ reabsorption/intracellular reduction of Na+ is driven by a Na+/K+-ATPase pump which pumps the excess Na+ into the interstitial fluid The intracellular HCO3- then diffuses from the tubule cell into the interstitial fluid Acid gradients across the tubules allow secretion of H+, influenced by pH of the urine (4.5) Interstitial fluid buffers (HCO3-, HPO42- and NH3) allow for active secretion of H+ across the tubule H+ + HCO3- H2O + CO2 (H+ ions diffuse back into tubular cells) REABSORPTION / Proximal convoluted tubules H+ + HPO42- H2PO4- (H+ excreted, reducing acid content) ACID EXCRETION / distal conv. tubules & collecting ducts H+ + NH3 NH4+ (aids in acid excretion, no effect on pH, Na+ or K+) / AMMONIA SECRETION Refer to Textbook, Fig25.23/ p1044 RENAL ACID BASE BALANCE http://www.youtube.com/watch?v=FgQiN049jS0 Metabolic Acidosis (non-respiratory) Kidneys cause a drop in Headache bicarb ion concentration Decreased BP Hyperkalaemia Kidneys not removing Muscle twitching enough acid through Warm, flushed skin (vasodilation) urination Nausea, vomiting, diarrhoea Drop in alkalinity leads to Confusion, drowsiness increase in [H+] creating acidic environment Possible causes: DKA, severe diarrhoea, renal failure, shock pH goes below 7.35 Metabolic Alkalosis (non-respiratory) Kidneys cause an increase in Restlessness followed by lethargy Dysrhythmias (tachycardia)Compensatory [HCO3-] Hypoventilation Results in decrease in [H+] and Confusion increase in pH Nausea, vomiting, diarrhoea Muscle tremors, cramps, tingling of extremities Hypokalaemia Possible causes: severe vomiting, excessive GI suctioning, diuretics Fundamental laws and concepts of thermodynamics Work and heat are inter-related concepts Heat is the transfer of thermal energy between 2 bodies, unequal temp, not equal to thermal energy Work is the force used to transfer energy between a system and its surroundings, and is needed to create heat and the transfer of thermal energy Both work and heat together allows systems to exchange energy Thermodynamics - interaction of heat and other types of energy Third, linking factor: the change in internal energy Energy cannot be created nor destroyed, but it can be converted or transferred Internal energy : kinetic energy + chemical bonds heat, work and internal energy: energy transfers/ conversions upon changes occur no net energy is created or lost First Law of Thermodynamics Energy can be converted from one form to another with the interaction of heat, work and internal energy, but it cannot be created nor destroyed, under any circumstances The equation that supports the 1St Law of Thermodynamics mathematically is: DE = q – w DE: change in free energy/ Internal energy q: heat (negative if heat flowed out of system, positive if heat introduced to system) w: work done on system by surroundings (negative) or vice versa (positive) DE = q – w Rxn: constant volume, no work done; only heat is transferred: DE = q Rxn: constant pressure, system will work on environment: DE = q – w work = pressure x change in vol w=PDV Rxn: initial & final temp are equal: DV = Dn[RT/P] R: universal gas constant n: moles of gas P: absolute pressure w = DnRT Rxn: constant pressure, heat released: q = DE + w = DE + PDV = DE + DnRT Enthalpy Enthalpy is the amount of heat content used or released in a reacting system at constant pressure It reflects the number and kinds of chemical bonds in the reactants and products When a chemical reaction releases heat, it is said to be exothermic, and the heat content of the reaction product is less than that of the reactants Enthalpy (H) is usually expressed as the change in enthalpy. The change in enthalpy (DH) is related to a change in internal energy (DE) and a change in the volume (DV), which is multiplied by the constant pressure of the system (P) DH = DE + PDV Biological systems – reactions occur in usually large volume of fluid, with no gases - little/no change DV PDV is also small/ negligible Enthalpy is reflected by internal energy in biological systems DH = DE Reversible & Irreversible Reactions Refer to notes 2nd Law of Thermodynamics Entropy represents the extent of disorder or randomness of the system & increases as a system approaches towards equilibrium under constant condition of temperature and pressure The 2nd Law of Thermodynamics: the state of entropy of the entire universe, as a closed, isolated system, will always increase over time The second law also states that the changes in the entropy in the universe can never be negative What happens when molecules go into solution? Solute molecules usually undergo an increase in entropy Free to dissociate from one another Ionic solutes - the cations can separate from the anions Solvent molecules more organized in the vicinity of the solute molecules than prior to solute introduction Contribution to total change in entropy is negative The net effect is slightly negative: the solution has lower entropy than the separated components