Drosophila Development Lecture Notes PDF
Document Details
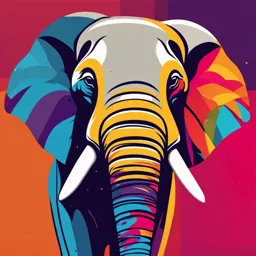
Uploaded by UsefulMoldavite3277
Concordia University
2020
Tags
Summary
These lecture notes cover the genetic mechanisms controlling Drosophila development. They detail the roles of maternal effect genes, gap genes, pair-rule genes, and homeotic selector genes in establishing the body plan. The notes also include diagrams and figures that illustrate various aspects of the discussed genes and their expression in the developing embryo.
Full Transcript
Lecture 16: Drosophila development Genetic mechanisms patterning the Drosophila body Recap Hierarchy: 1. Maternal effect genes (maternal mRNAs) Code for transcription factors and translational regulatory...
Lecture 16: Drosophila development Genetic mechanisms patterning the Drosophila body Recap Hierarchy: 1. Maternal effect genes (maternal mRNAs) Code for transcription factors and translational regulatory proteins that act as morphogens. 2. Gap genes The first zygotic genes to be expressed. Their expression is regulated by maternal effect genes. 3. Pair-rule genes Their expression is regulated by gap genes. 4. Segment polarity genes Their expression is regulated by pair-rule genes. 5. Homeotic selector genes The protein products of gap, pair-rule, and segment polarity genes interact to regulate expression of homeotic selector genes. Maternal gradients: anterior-posterior polarity Recap The AP axis is determined by two maternal mRNAs that act as morphogens: bicoid and nanos. Hunchback and caudal are another two other maternal mRNAs critical for anterior-posterior patterning. Bicoid and Nanos proteins are regulators of translation. Bicoid prevents translation of caudal mRNA. Nanos prevents translation of hunchback mRNA. Gap genes Gap genes are under control of the maternal effect genes (either activation or repression). Expressed in one or two broad domains along the anterior-posterior axis. The expression patterns of the gap genes correlates with the region of the embryo that is missing when that particular gap gene is missing or mutated. Each of the four major gap genes (Hunchback, Knirps, Giant, and Krüppel) encode DNA-binding proteins. Gap genes Gap genes mRNAs are initially expressed at low levels throughout the entire embryo. As nuclear divisions continue, their expression becomes more concentrated in specified areas. The maternal Hunchback, Bicoid and Caudal gradients are important in establishing the initial patterns of gap gene expression. Establishment of initial gap gene expression mRNA expression of gap genes High levels of Hunchback and Bicoid activate expression of giant (gt). Tailless inhibits expression of Giant → no expression at the extreme anterior end. Moderate levels of Hunchback activate expression of Krüppel (kr) Li et al. 2008. Transcription factors bind thousands of active and inactive regions in the Drosophila blastoderm. Establishment of initial gap gene expression Hunchback inhibits the transcription of knirps (kni) in the anterior end. mRNA expression of gap genes A gradient of Caudal is responsible for activating the abdominal gap genes knirps and giant. giant has two modes of activation: one for its anterior expression band (through Bicoid and Hunchback), and one for its posterior expression band (Caudal). Li et al. 2008. Transcription factors bind thousands of active and inactive regions in the Drosophila blastoderm. Gap gene expression networks After these initial gap gene expression patterns have been established by the maternal effect gradients, they are stabilized and maintained by repressive interactions between the different gap proteins themselves. There are three major mechanisms that control the network. 1. Mutual inhibition by Hunchback and Knirps Hunchback and Knirps proteins mutually inhibit each other’s mRNA expression. The mutual inhibition of Kni and Hb positions the Knirps protein domain at around 60%-80% along the anterior-posterior axis. 2. Concentration-dependent regulation of Krüppel by Hunchback The nature of the interaction between Hunchback and Krüppel is concentration-dependent. High concentration of Hunchback: Hb inhibits the transcription of Kr Moderate concentrations of Hunchback: Hb promotes the transcription of Kr 3. Mutual inhibition by Krüppel and Giant Krüppel and Giant mutually inhibit each other’s mRNA expression. Gap gene expression networks The end result of these repressive interactions is the creation of a precise system of overlapping mRNA expression patterns. Expression of the gap proteins occurs while the nuclei are still in a syncytium. Gap proteins diffuse into adjacent embryonic regions and this creates overlaps of protein expression (~8 nuclei wide). Hunchback protein: red Krüppel protein: green Overlap: yellow Pair-rule genes Pair-rule genes begin to be expressed around nuclear division cycle 13, and their transcription patterns divide the embryo into regions that are the precursors of the segmental body plan. Pair-rule genes dictate parasegmental mRNA expression of two pair-rule genes: even-skipped (red) and fushi boundaries. tarazu (black) Pair-rule genes 8 known pair-rule genes, each expressed in 7 stripes. Pair-rule genes are expressed in alternating parasegments. E.g. even-skipped is expressed in every odd- numbered parasegment, and fushi tarazu is mRNA expression of two pair-rule expressed in every even-numbered genes: even-skipped (red) and fushi tarazu (black) parasegment. Pair-rule genes Each pair-rule gene is expressed in a specific part of the parasegment. Ftz is expressed at the anterior boundaries of all even-numbered parasegments. Eve is expressed at the anterior end of all odd-numbered parasegments. Figure modified from: Okochi et al. 2021 Pair-rule genes The pair-rule genes can overlap each other, this means each stripe of nuclei in every parasegment contains a specific combination of pair-rule genes. Figure modified from: Okochi et al. 2021 Pair-rule gene expression and regulation Primary pair-rule genes appear first, and their expression is regulated by the maternal effect genes and gap genes. hairy, even-skipped, runt Secondary pair-rule genes are downstream in the cascade and are regulated by the primary pair-rule genes. fushi tarazu, odd-paired, odd-skipped, sloppy-paired, paired Regulation of pair-rule expression Each pair-rule gene is controlled by multiple enhancers. Each enhancer controls the expression of a separate stripe or a pair of stripes. Even-skipped (eve) is the best studied primary pair-rule gene. The stripe 2 enhancer of even-skipped Every enhancer contains a different DNA sequence → every enhancer can be bound by different sets of transcription factors. The transcription factors that regulate primary pair-rule gene expression are the gap genes (and some maternal effect genes). The stripe 2 enhancer of even-skipped Stripe 2 enhancer contains binding sites for Bicoid, Hunchback, Giant, and Krüppel. Bicoid and Hunchback activate this enhancer. The stripe 2 enhancer of even-skipped Giant and Krüppel repress this enhancer. The stripe 2 enhancer of even-skipped In the row of nuclei where stripe 2 will be expressed, there is both Hunchback and Bicoid. There is no Giant or Krüppel. Eve will be expressed in this row of nuclei to generate stripe 2. Bicoid and Hunchback are required stripe 2 for expression of stripe 2, and Giant and Krüppel are responsible for setting the anterior and posterior borders of the stripe, respectively. How did scientists determine that each stripe was expressed independently with different enhancers? 1. Mutated/deleted enhancers affected only certain stripes and not others. 2. Placement of the stripes can be altered by deleting the gap genes that regulate them. 3. Reporter assays determined that enhancers will drive expression of the reporter gene in only one particular stripe. Reporter assays enhancer/promoter to be studied reporter gene (e.g. GFP, LacZ) DNA Report assays test if a particular reporter gene (e.g. GFP, LacZ) mRNA enhancer or promoter region is sufficient for gene expression. They also allow you to determine if a region of DNA contains a Reporter regulatory element. protein Reporter assays The posterior border stripe of stripe 5 is missing in the giant- deficient embryo. This means Giant is involved in maintaining the posterior border of stripe 5. Secondary pair-rule genes Once initiated by the gap gene proteins, the transcription pattern of the primary pair-rule genes becomes stabilized by interactions among their products. The primary pair-rule genes also regulate (activate or inhibit) the expression of the later-acting secondary pair-rule genes. Each row of nuclei within a parasegment contains its own array of pair-rule products that distinguishes it from any other row. *don’t need to know the patterns of these pair-rule genes. It is just to show you that each one is expressed in a different pattern, and they often overlap with each other. Clark et al. 2016. Odd-paired controls frequency doubling in Drosophila segmentation by altering the pair-rule gene regulatory network. eLife. Practice question You are studying the expression of even-skipped in hundreds of embryos and you notice that in one embryo, stripe 2 is thicker than the rest of the stripes. The anterior end begins where it is supposed to, but the posterior end is extended beyond its normal placement. All other stripes are normal. You confirm that there are no mutations in any protein coding sequences in the genome. What could be causing this phenotype in this embryo? Segment polarity genes Segment polarity genes are expressed in some region of every segment (e.g. the posterior end of every segment). Segment polarity gene mutants show defects (deletions, duplication, polarity reversals) in every segment. Their expression is induced by pair- rule genes. Segment polarity genes Expression is around the 13 th-14th nuclear division, after pair-rule genes. Reminder: cellularization occurs at between the 13th and 14th nuclear division. Once cells form, interactions take place between cells, not between molecules within a syncytium. Segment polarity genes have two functions: 1. Reinforce the segmental periodicity established by the earlier transcription factors. 2. Mediate cell-cell communication to establish cell fate within each parasegment. Segment polarity genes The segment polarity genes encode components of the Wnt and Hedgehog signaling pathways. Wingless - Drosophila Wnt protein Wnt and Hedgehog signal transduction pathways Wnt and Hedgehog are paracrine factors: protein is synthesized by one cell and released to induce a signal transduction cascade in a neighboring cell. Hedgehog and Wingless Normal development and segmentation relies on the fact that, initially, only one row of cells in each parasegment is permitted to express the Hedgehog protein, and only one row of cells in each parasegment is permitted to express the Wingless protein. Rows of cells in a parasegment Cells Cells expressing expressing Hedgehog Wingless The key to this pattern is the expression pattern of pair-rule genes that will dictate the expression pattern of a segment polarity gene called “engrailed”. Cells that express engrailed will express Hedgehog. engrailed (en) – a major player The expression of the segment polarity gene engrailed is key to establishing the appropriate pattern of Wingless (Wg) and Hedgehog (Hh) expression (one row of cells expressing Hh, and another expressing Wg). engrailed is expressed in the cells that will go on to express Hh. engrailed is expressed in 14 stripes across the anterior-posterior axis of the embryo. engrailed expression en expression is activated in cells that mRNA expression of have high levels of Even-skipped, Fushi two pair-rule genes: tarazu, or Paired transcription factors. even-skipped (red) and fushi tarazu en expression is repressed in cells with (black) high levels of Odd-skipped, Runt, or Sloppy-paired proteins. As a result, the Engrailed protein is found in 14 stripes across the AP axis. In fushi tarazu-deficient embryos, only 7 bands of engrailed are expressed. Protein expression of Engrailed seen at the extended germ band stage. Establishment + initiation of engrailed and wingless expression Expression of engrailed and wingless is initiated by pair-rule genes. en is expressed in bands of cells that have high concentrations of either Even- skipped or Fushi tarazu proteins and no Odd-skipped, Runt, or Sloppy-paired proteins → the result is expression of engrailed in the anterior band of cells in each parasegment (and the posterior of each segment). Establishment + initiation of engrailed and wingless expression Expression of engrailed and wingless is initiated by pair-rule genes. wg is expressed in bands of cells that receive little to no Even-skipped or Fushi tarazu but do contain Sloppy-paired → the result is expression of wg in the column of cells directly anterior to end (posterior margin of each parasegment). Maintenance of wingless and engrailed expression patterns Pair-rule mRNAs and proteins degrade. Patterns must be maintained after their initiators are no longer being synthesized. The maintenance of these patterns is regulated by reciprocal interactions between neighboring cells. Maintaining wingless and engrailed expression patterns The continued expression of wg and en is maintained by cell-cell interactions between cells expressing Engrailed and Wingless. 1. Wingless is secreted + diffuses to surrounding cells. 2. Wingless activates the Frizzled receptor on cells expressing engrailed (only cells competent to express engrailed have the Frizzled receptor). 3. Engrailed protein activates expression of hedgehog + its own (en) transcription. Maintaining wingless and engrailed expression patterns 4. Hedgehog is secreted + diffuses to neighboring cells. 5. Hedgehog binds the Patched receptor and activates transcription of wingless in neighboring cells. Hedgehog and Wingless maintain each other’s expression. Gradients of Wg and Hedgehog signaling are responsible for different cuticular structures in the dorsal epidermis Hedgehog and Wingless initiate complex patterns of gene expression that cause different structures to be formed on the surface of dorsal cells. 1° cells - express thick denticles 2° cells – naked 3° cells – small thick hairs 4° cells – small fine hairs Hedgehog mutant Hedgehog mutants were named because in the absence of hedgehog the Drosophila larva cuticle was covered in the large spiked denticles. In the absence of Hedgehog, the Wg gradient is disrupted. Disruption of Hedgehog and Wg signaling causes denticles to be formed all over the dorsal side of the embryo. Homeotic selector genes After establishment of the segment polarity genes, the segment boundaries are set. Pair-rule genes, gap genes, and segment polarity genes interact to regulate the homeotic selector genes. Homeotic selector genes specify the characteristic structures of each segment. Mutations in homeotic selector genes lead to body segments taking on new identifies (like a segment growing wings instead of legs for example). Homeotic selector genes By the end of the cellular blastoderm stage, each segment primordium has been given a unique identity through its unique constellation of gap, pair-rule, and homeotic gene products. Homeotic selector genes Most homeotic selector genes in Drosophila are found on two regions in chromosome III. Together, these regions are called Hom-C (homeotic complex). Homeotic selector genes encode transcription factors. Homeotic selector genes Collinearity is found between the order of the genes on the chromosome and the other in which the genes are expressed along the anteroposterior axis of the embryo. lab is expressed on the 3’ position on the chromosome and is expressed in the most anterior head region of the embryo. Homeotic selector genes Antennapedia region: Contains the homeotic genes labial (lab), deformed (dfd), sex combs reduced (scr), Antennapedia (Antp), and proboscipedia (pb). labial and deformed: head segments sex combs reduced and Antennapedia: first and second thoracic segments, respectively proboscipedia acts only in adult flies, but in its absence, the labial palps of the mouth are transformed into legs. Homeotic selector genes Bithorax complex: Contains Ultrabithorax (Ubx), Abdominal A (AbdA), and Abdominal B (AbdB). Ultrabithorax: identity of the third thoracic segment AbdA and AbdB: segmental identities of the abdominal segments Homeotic selector genes Most genes are expressed at a high level (darker color) throughout one segment, and at a lower level in some adjacent segments (lighter color). In regions where the expression domains overlap, the more “posterior” gene determines the phenotype of the segment. E.g. in segment T3, both Ubx and Antp are expressed, but Ubx is the more posterior T3 gene, and Ubx will determine the identity of the segment (third thoracic segment) Homeotic gene regulation 1. Homeotic gene expression is regulated by gap genes and pair-rule genes: Example: Expression of abdA and AbdB genes are repressed by the gap proteins Hunchback and Krüppel. This inhibition prevents these abdomen- specifying genes from being expressed in the head and thorax. Homeotic gene regulation 2. Some homeotic genes are negatively regulated by the homeotic gene products expressed posteriorly to it. E.g. every gene in the bithorax complex represses the expression of Antennapedia. If Ubx is deleted or mutated, Antp activity extends through the region that would normally express Ubx and stops where the abdA region begins. E.g. Abd-B reduces the expression of Abd-A in the most posterior segments. Homeotic mutations Homeotic mutation: transformations of body regions or segments T1: legs (specified by scr) T2: legs + wings (specified by Antp) T3: legs + halteres (specified by Ubx) Ubx both suppresses wing formation and activates haltere formation. Homeotic mutations Wild-type: Ubx mutant: Homeotic mutations Antennapedia specifies the second thoracic segment (T2 – legs + wings) by: Promoting expression of T2 genes Repressing genes critical for antenna and eye formation in the T2 segment Loss of function antennapedia mutations: legs in T2 transform into antennae. Homeotic mutations Wild-type: Gain of function Antp mutant: In this mutant, Antp protein is overexpressed in the head segment where it is normally not expressed. Homeotic mutations in humans Synpolydactyly (SPD): mutations in the HOXD13 gene Extra fingers – polydactyly Webbed fingers – syndactyly Dominant mutation that affects the function of other Hox genes The same mutation in the homologous gene in mice also causes limb defects. Bruneau et al. 2001. The Mouse Hoxd13spdh Mutation, a Polyalanine Expansion Similar to Human Type II Synpolydactyly (SPD), Disrupts the Function but Not the Expression of Other Hoxd Genes Goodman and Scambler 2001. Human HOX gene mutations. Clinical Genetics. Midterm 2 material ends here.