Lecture 15 - Muscle PDF
Document Details
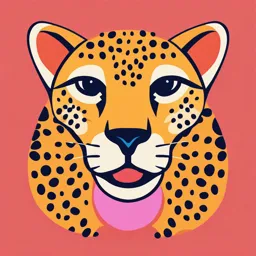
Uploaded by ElegantFantasy
Tags
Summary
This document provides an overview of muscle types, including skeletal, cardiac, and smooth muscle. It details the molecular structure and organization of these tissues, and explains the mechanisms of muscle contraction. The text covers aspects such as actin and myosin filaments, the role of ATP, and calcium regulation.
Full Transcript
LECTURE 15 – Muscle Three types of muscle cells can be recognised: skeletal muscle, cardiac muscle and smooth muscle. Although the two former types of cells have a common molecular organization, being constituted by sarcomeres and appearing at the microscope as striated muscle cells, the mechanisms...
LECTURE 15 – Muscle Three types of muscle cells can be recognised: skeletal muscle, cardiac muscle and smooth muscle. Although the two former types of cells have a common molecular organization, being constituted by sarcomeres and appearing at the microscope as striated muscle cells, the mechanisms that produce the contraction are different and specific for each of the three types of cells. In all three types of cells, the contraction is produced by the interaction of actin and myosin filaments, the energy is derived by cleavage of ATP, and myosin is the partner that possesses ATPase activity and produces the force for the contraction. However, in striated muscle myosin is always ready to do the work (provided ATP is available) and the regulation of contraction depends on the availability of binding sites for myosin on the actin filament, which in turn depends on the presence of calcium ions in the cytosol; vice versa, in smooth muscle myosin activity is modulated by phosphorylation, and therefore the force developed by the muscle (and contraction) can be finely graded. A further major difference among the three types of cells consists in the pattern of contraction they can produce. In striated muscle the contraction is triggered and sustained by the cytosolic level of Ca2+ that rises during the action potential; thus, the contraction consists in a very brief twitch in skeletal muscle, which displays a neuron-like action potential, lasting a few ms, whereas it can be maintained for tens or hundreds of ms in cardiac myocytes, which have a much more prolonged action potential; finally, contraction can be maintained indefinitely in smooth muscle either in a dynamic way, by sustaining the phosphorylation and ATPase activity of myosin, or in a static way by blocking myosin and locking it to actin. Striated muscle Striated muscle fibres are syncytia (multinucleated cells) of variable length, filled by contractile material which is organised in a very ordered way: elementary structural units, called sarcomeres, give rise to the striated (striped) appearance. Each sarcomere is constituted by the space between two septa, called Z bands – orthogonal to the major axis of he fibre and spaced about 1 micron apart – to which actin filaments are attached. The latter are directed toward the centre of the sarcomere and organized in a hexagonal pattern. Myosin filaments are in the middle of the sarcomere (M band), positioned at the centre of each hexagonal pattern of actin filaments and overlapping actin filaments attached to either Z bands. The alternating regions of overlap determine the darker bands. Myosin is organized in large filaments, constituted by bundles of myosin tails, from which myosin heads protrude in a helicoidal way, at 60° intervals, so to face the six filaments of actin that surround the myosin filament. At rest, myosin is bound to ADP and inorganic phosphate: binding of ATP has reduced its affinity for actin, but it has rapidly cleaved ATP, acquiring a strained (high free energy) conformation, the head extended on the tail, and regaining a high affinity for actin. In the resting muscle the binding sites for myosin on actin are masked by the protein complex of tropomyosin + troponin; the latter is an oligomer constituted by troponin I, the subunit that binds to actin and maintains the complex in a position that inhibits the interaction of the myosin head with actin, troponin T that binds to tropomyosin and troponin C that interacts with calcium ions. In the presence of the latter, troponin changes conformation and frees the myosin binding site. As soon as this occurs, the myosin head will bind to the binding site on actin that faces it and discharge the accumulated energy, by releasing ADP and P and producing the power stroke that pulls on actin. If ATP is available, myosin will bind it, lose affinity for actin, detach, cleave ATP, extend back to the strained conformation and, if the next binding site on actin is still not masked, bind and repeat the cycle. If ATP is not available, myosin will remain attached to actin (rigor mortis). Given the organisation of the actin and myosin filaments in the sarcomere, striated muscle cannot shorten by more than some 30% with respect to its maximum length. Excitation contraction coupling in skeletal muscle Calcium ions are the trigger for skeletal muscle contraction. Since skeletal muscle cells may have a diameter as large as 40 or more μm, a calcium signal cannot spread in the cytosol quickly enough to produce synchronous activation of the contractile material in the whole cell (Ca2+ has a coefficient of diffusion in the cytosol in the order of 10 μm2 /s). The organisation of the sarcoplasmic reticulum, T-tubules and “triads” overcomes this problem. The sarcoplasmic reticulum (SR) is organized in longitudinal tubules, around fascicules of contractile material, that end in cisternae associated to the two sides of the T-tubules; these are invaginations of the sarcolemma that cross the muscle cell, transversally, in correspondence with the Z discs. When the action potential invades the sarcolemma, it also spreads to the T-tubules. Here, L-type voltage-gated calcium channels (also called di-hydropyridine receptors, DHPR) open, changing conformation. Where the terminal cisternae of the SR contact the T tubules, ryanodine receptors (RYR, Ca2+ channels) are in direct contact with DHPRs, so to open when the latter are activated. This way, Ca2+ is released from the SR into the cytosol; calcium-induced calcium release is triggered and Ca2+ concentration simultaneously rises all over the cell. The volume of the T-tubules is ridiculously small, as compared to cell volume; so, the possible inflow of Ca2+ through the DHPRs is irrelevant. Given the small surface to volume ratio of skeletal muscle fibres, also the amount of Ca2+ that enters across the sarcolemma is essentially irrelevant; most movements of Ca2+ occur between the cytosol and the SR, and the skeletal muscle can contract even in the absence of extracellular calcium. The contraction of a skeletal muscle fibre is very brief, because the action potential is over in about 1-2 ms and Ca2+ is quicly cleared by the activity of the SERCA. So, the skeletal muscle produces a brief twitch (30-100 ms). To produce a prolonged contraction, it must be stimulated repetitively. Cardiomyocytes The striated muscular cells of the myocardium do not constitute a syncytium, although they are reciprocally coupled through gap junctions. The surface to volume ratio is much higher here and dyads are present (connections of SR cysternae with the plasma-membrane); the movements of Ca2+ between cytosol and extracellular fluid are much more relevant. The action potential of cardiac myocytes is much longer than that of neurons and skeletal muscles, because the depolarization triggers a large calcium current through L-type channels, which builds up and wanes much more slowly than the sodium current. The action potential typically lasts several tens of ms in the atrial cells and hundreds of ms in ventricular cells. This way, calcium levels in the cytosol are maintained elevated and the contraction persists for a similar length of time (which is needed to effectively eject the blood from the ventricles into the aorta and pulmonary artery). The first difference, with respect to the skeletal muscle, is therefore that the contraction does not consist in a transient twitch, here, but can be sustained. A second difference is that the entrance of calcium through the plasma-membrane is relevant here, in determining the level of Ca2+ during the contraction, because the amount of Ca2+ that enters also influences the amount accumulated in the SR and released during the next cycle. Since a higher level of cytosolic Ca2+ during the contraction implies a higher probability for troponin to be activated, and for the myosin binding site on actin to be available, the force of contraction can be modulated in cardiomyocytes. In particular, the force of contraction (contractility) can be modulated by enhancing the activity of L-type channels (phosphorylation by PKA), by enhancing the activity of the SERCA (phosphorylation of phospholamban by PKA) or by impairing the activity of the Na-Ca exchanger, so that most of the Ca2+ present during the contraction is stored in the SR rather than extruded through the plasmalemma. The smooth muscle In the smooth muscle, excitation-contraction coupling and the contraction mechanism are quite different. Whereas in striated muscle the regulation is on actin (exposing binding sites for myosin when Ca2+ is present), myosin being always ready to bind and pull, in smooth muscle contraction is regulated on myosin, by modulating its ATPase activity. The enzymatic activity of Myosin depends on the state of phosphorylation of its light chains: myosin light chain kinase (MLCK) activates myosin whereas myosin light chain phosphatase (MLCP) reduces its activity. MLCK is activated by the Ca-calmodulin complex, and inhibited by cAMP (phosphorylation by PKA that interferes with Ca-CaM binding); MLCP is inhibited by Rho kinase and activated by cGMP. As a consequence, there are two main ways of producing smooth muscle contraction: depolarization and consequent entry of Ca2+ through voltage-gated calcium channels, or activation of Gq-coupled receptors, leading to release of Ca2+ from the ER. Conversely, there are two ways of producing smooth muscle relaxation, either by activating a Gs-coupled receptor, leading to elevation of cAMP, or by activating guanylyl cyclase (mainly through the action of NO). Notice that a Gq-coupled receptor on the smooth muscle of a vessel produces vasoconstriction, while a Gqcoupled receptor on the endothelial cell produces activation of NO-synthase, migration of NO to the surrounding muscle, where it favours the production of cGMP and relaxation (vasodilation). A second difference consists in the organization of the contractile material: actin filaments are not all parallel; they cross each-other and connect diagonally opposed points of the cell membrane; this way, the smooth muscle cell can reduce its length much more than the striated one. This is particularly important for the function of sphincters, where a massive decrease in fibre length is required. A third and quite relevant difference consists in the fact that the myosin cycle can be slowed down in the smooth muscle, so that the release of ADP and inorganic phosphate, that prevents binding of ATP and detachment from actin, are delayed; this way the myosin-actin bond persists longer and the fibre remains in a “locked” state, maintaining production of force without expenditure of ATP.