Human Physiology Lecture 12 - Nervous System PDF
Document Details
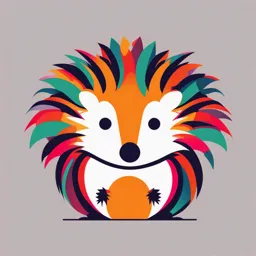
Uploaded by AdventurousNeumann
Tags
Summary
This document is a lecture on the nervous system, part of human physiology. It covers the structure and function of neurons and synapses, as well as the concepts of action potentials and neurotransmitters.
Full Transcript
Human Physiology Lecture:12 Nervous System If the body is to maintain homeostasis and function effectively, its trillions of cells must work together in a coordinated fashion. If each cell behaved without regard to what others were doing, the result would be physiological chaos and death. This is...
Human Physiology Lecture:12 Nervous System If the body is to maintain homeostasis and function effectively, its trillions of cells must work together in a coordinated fashion. If each cell behaved without regard to what others were doing, the result would be physiological chaos and death. This is prevented by two communication systems—the nervous system, which is specialized for the rapid transmission of signals from cell to cell, and the endocrine system, which is specialized for sending chemical messengers, the hormones, through the blood. The fundamental purpose of the nervous system is: (1) To receive information from receptors—cells and organs specialized to detect changes in the body and its external environment; (2) To process this information and determine the appropriate response, if any—a step called neural integration; (3) To tissue commands to effectors, cells and organs (mainly muscle and gland cells) that carry out the body’s responses. The Nervous System is comprised of two major subsystems: The Central Nervous System (CNS) The Peripheral Nervous System (PNS) 1. Central Nervous System (CNS) it consists of the brain and the spinal cord. It receives and sorts out information coming from the environment and from inside the body and determines the appropriate action. 2. Peripheral Nervous System (PNS) it is made up of nerves that extend throughout the body. It is through these nerve cells that communication between the central nervous system and the body tissues take place. The Peripheral Nervous System (PNS) is comprised of the: Somatic Nervous System Autonomic Nervous System Neuron Structure 1-Cell Body Nucleus – genetic information 2- Dendrites Receive information 3- Axon Carry information “long” distances Myelin (Multiple Sclerosis) 4-Axon Terminals Transmit information Universal Properties The communicative role of the nervous system is carried out by nerve cells, or neurons. These cells have three fundamental physiological properties that are necessary to this function: 1. Excitability (irritability). All cells possess excitability, the ability to respond to environmental changes called stimuli. Neurons have developed this property to the highest degree. 2. Conductivity. Neurons respond to stimuli by producing traveling electrical signals that quickly reach other cells at distant locations. 3. Secretion. When the electrical signal reaches the end of a nerve fiber, the neuron secretes a chemical neurotransmitter that “jumps the gap” and stimulates the next cell. Establishment of the Resting Membrane Potential Membranes are polarized or, in other words, exhibit a RESTING MEMBRANE POTENTIAL. This means that there is an unequal distribution of ions on the two sides of the nerve cell membrane. This POTENTIAL generally measures about 70 millivolts (with the INSIDE of the membrane negative with respect to the outside). So, the RESTING MEMBRANE POTENTIAL is expressed as -70 mV, and the minus means that the inside is negative relative to the outside. It is called a RESTING potential because it occurs when a membrane is not being stimulated or conducting impulses (in other words, it's resting). What factors contribute to this membrane potential? Two ions are responsible: sodium (Na+) and potassium (K+). An unequal distribution of these two ions occurs on the two sides of a nerve cell membrane because carriers actively transport these two ions: sodium from the inside to the outside and potassium from the outside to the inside. AS A RESULT of this active transport mechanism (commonly referred to as the SODIUM - POTASSIUM PUMP), there is a higher concentration of sodium on the outside than the inside and a higher concentration of potassium on the inside than the outside. Action potential An action potential is a very rapid change in membrane potential that occurs when a nerve cell membrane is stimulated. Specifically, the membrane potential goes from the resting potential (typically -70 mV) to some positive value (typically about +30 mV) in a very short period of time (just a few milliseconds). An action potential is a more dramatic change produced by voltage-regulated ion gates in the plasma membrane. Action potentials occur only where there is a high enough density of voltage-regulated gates. Most of the soma has only 50 to 75 gates per square micrometer and cannot generate action potentials. The trigger zone, however, has 350 to 500 gates per square micrometer. If an excitatory local potential spreads all the way to the trigger zone and is still strong enough when it arrives, it can open these gates and generate an action potential. Threshold stimulus & potential Action potentials occur only when the membrane in stimulated (depolarized) enough so that sodium channels open completely. The minimum stimulus needed to achieve an action potential is called the threshold stimulus. If the membrane potential reaches the threshold potential (generally 5 - 15 mV less negative than the resting potential), the voltage-regulated sodium channels all open. Sodium ions rapidly diffuse inward, & depolarization occurs. All-or-None Law - action potentials occur maximally or not at all. In other words, there's no such thing as a partial or weak action potential. Either the threshold potential is reached and an action potential occurs, or it isn't reached and no action potential occurs. The successive stages of the action potential are as follows: Resting Stage: This is the resting membrane potential before the action potential begins. Depolarization Stage: At this time, the membrane suddenly becomes very permeable to sodium ions, allowing tremendous numbers of positively charged sodium ions to diffuse to the interior of the axon. Repolarization Stage: Within a few 10,000ths of a second after the membrane becomes highly permeable to sodium ions, the sodium channels begin to close and the potassium channels open more than normal. Then, rapid diffusion of potassium ions to the exterior re-establishes the normal negative resting membrane potential. This is called repolarization of the membrane The Refractory Period During an action potential and for a few milliseconds after, it is difficult or impossible to stimulate that region of a neuron to fire again. This period of resistance to re -stimulation is called the refractory period Absolute refractory period is the period during which another action potential cannot be elicited. Explanation: The inactivation gates of the Na + channel are closed and will remain until repolarization occurs. No action potential can occur until the inactivation gates open. Relative refractory period -begins at the end of the absolute refractory period and continues until the membrane potential returns to the resting level. -An action potential can be elicited during this period if a stronger than usual current is provided. Explanation:The slow return of the K+ channels to the closed state explains the after-hyperpolarization and hence relative refractory period. During this time, only a very strong depolarization can overcome the repolarization effects of the open K+ channels and produce a second action potential Synapses and Neurotransmitters a nerve signal can go no further when it reaches the end of the axon – action potential must trigger the release of a neurotransmitter – stimulates a new wave of electrical activity in the next cell across the synapse synapse between two neurons: – 1st neuron in the signal path is the presynaptic neuron releases neurotransmitter. – 2nd neuron is postsynaptic neuron responds to neurotransmitter. presynaptic neuron – may synapse with Dendrite Soma Axon of postsynaptic neuron – form different types of synapses Axodendritic synapses Axosomatic synapses Axoaxonic synapses The Discovery of Neurotransmitters The synaptic cleft – gap between neurons was discovered by Ramón y Cajal through histological observations. Otto Loewi, in 1921, demonstrated that neurons communicate by releasing chemicals – the chemical synapses ,he flooded exposed hearts of two frogs with saline stimulated vagus nerve of the first frog and the heart slowed. – removed saline from that frog and found it slowed heart of second frog – named it Vagusstoffe (“vagus substance”) later renamed acetylcholine, the first known neurotransmitter. presynaptic neurons have synaptic vesicles with neurotransmitter and postsynaptic have receptors and ligand-regulated ion channels fall into four major categories according to chemical composition – acetylcholine in a class by itself formed from acetic acid and choline – amino acid neurotransmitters include glycine, glutamate, aspartate, and γ-aminobutyric acid (GABA) – monoamines synthesized from amino acids by removal of the –COOH group retaining the –NH2 (amino) group major monoamines are: – the catecholamines = epinephrine, norepinephrine, dopamine – histamine and serotonin – neuropeptides Synaptic Transmission neurotransmitters are diverse in their action – some excitatory – some inhibitory – some the effect depends on what kind of receptor the postsynaptic cell has. – some open ligand-regulated ion gates. – some act through second-messenger systems. synaptic delay – time from the arrival of a signal at the axon terminal of a presynaptic cell to the beginning of an action potential in the postsynaptic cell – 0.5 msec for all the complex sequence of events to occur Three kinds of synapses with different modes of action – excitatory cholinergic synapse – inhibitory GABA-ergic synapse – excitatory adrenergic synapse Excitatory Cholinergic Synapse cholinergic synapse – employs acetylcholine (ACh) as its neurotransmitter – ACh excites some (most!) postsynaptic cells – inhibits others describing excitatory action – nerve signal approaching the synapse, opens the voltage-regulated calcium gates in synaptic knob – Ca2+ enters the knob – triggers exocytosis of synaptic vesicles releasing ACh – empty vesicles drop back into the cytoplasm to be refilled with ACh – reserve pool of synaptic vesicles move to the active sites and release their ACh – ACh diffuses across the synaptic cleft – binds to ligand-regulated gates on the postsynaptic neuron – gates open – allowing Na+ to enter cell and K+ to leave pass in opposite directions through same gate – as Na+ enters the cell it spreads out along the inside of the plasma membrane and depolarizes it producing a local potential called the postsynaptic potential – if it is strong enough and persistent enough – it opens voltage-regulated ion gates in the trigger zone – causing the postsynaptic neuron to fire Inhibitory GABA-ergic Synapse GABA-ergic synapse employs γ-aminobutyric acid as its neurotransmitter.r nerve signal triggers release of GABA into synaptic cleft. GABA receptors are chloride channels. Cl- enters cell and makes the inside more negative than the resting membrane potential. postsynaptic neuron is inhibited less likely to fire. Excitatory Adrenergic Synapse adrenergic synapse employs the neurotransmitter norepinephrine (NE) also called noradrenaline Receptor for adrenergic synapses not an ion gate – a transmembrane protein associated with a G protein NE and other monoamines, and neuropeptides acts through second messenger systems such as cyclic AMP (cAMP) Action of Adrenergic Transmembrane Receptor and G Protein unstimulated NE receptor is bound to a G protein. binding of NE to the receptor causes the G protein to dissociate from it. G protein binds to adenylate cyclase, activates this enzyme. induces the conversion of ATP to cyclic AMP. cyclic AMP can induce several alternative effects in the cell. -causes the production of an internal chemical that binds to a ligand-regulated ion gate from inside of the membrane, opening the gate and depolarizing the cell. -can activate preexisting cytoplasmic enzymes that lead do diverse metabolic changes. -can induce genetic transcription, so that the cell produces new cytoplasmic enzymes that can lead to diverse metabolic effects.