Lecture 10 Cargo Packaging and Vesicular Fusion PDF
Document Details
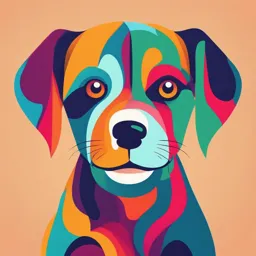
Uploaded by EnthusiasticTheremin
Tags
Summary
This lecture discusses cargo packaging and vesicular fusion, focusing on the Golgi apparatus and lysosomes. It also explores different methods for studying vesicular transport, including the isolation of yeast mutants and the use of fluorescent microscopy.
Full Transcript
Lecture 10 Cargo Packaging and Vesicular fusion Lysosomes The Golgi Apparatus conjugating amide bond Glycolipids and sphingomyelin are synthesized from Sphi...
Lecture 10 Cargo Packaging and Vesicular fusion Lysosomes The Golgi Apparatus conjugating amide bond Glycolipids and sphingomyelin are synthesized from Sphingosine ceramide in the Golgi. Ceramides are group of waxy lipids. They are composed of sphingosine and fatty acid tail. Sphingomyelin is synthesized by transfer of a phosphorylcholine group from phosphatidylcholine to ceramide. fatty acid tail Addition of carbohydrates to ceramide yields a variety of different glycolipids. Protein sorting and export in Golgi In the trans Golgi network, molecules are sorted and packaged into transport vesicles. Proteins that need to stay in the Golgi are associated with the membrane and contain signals that prevent packaging and transport. Transport from the Golgi to the cell surface can occur by three routes: Direct transport to the plasma membrane Recycling endosomes Regulated secretory pathways Regulated pathways include release of hormones from endocrine cells and neurotransmitters from nerve cells. These proteins aggregate in the trans-Golgi network and are packaged in secretory granules. The granules store their contents until signals direct their fusion with the plasma membrane. Example of membrane domains: In polarized cells of epithelial tissue, plasma membranes are divided into apical domains and basolateral domains, each with specific proteins. Proteins leaving the trans Golgi network must be selectively packaged and transported to the correct domain. How do we study vesicular transport? The selectivity of vesicular transport is key to maintaining the functional organization of a cell. Vesicles must recognize and fuse only with the appropriate target membrane. Understanding the mechanisms that control vesicular transport is a major area of research in cell biology. Three experimental approaches have been used: 1. Isolation of yeast mutants defective in protein transport and sorting 2. Reconstitution of vesicular transport in cell-free systems 3. Biochemical analysis of synaptic vesicles 1. Yeasts mutants can be defective at various stages of protein secretion (sec mutants), or are unable to transport proteins to the vacuole, or retain resident ER proteins. Isolation of mutants led to molecular cloning and analysis of corresponding genes and identification of proteins. 2. Reconstituted systems (in vitro): Enabled isolation of transport proteins, study of the transport process, and functional analysis of the proteins identified by mutations in yeasts. 3. Synaptic transmission in neurons is a specialized form of regulated secretion. Synapse: junction of a neuron with another cell. Chemical neurotransmitters are stored in the neuron in synaptic vesicles. Stimulation of the neuron triggers fusion of synaptic vesicles with the plasma membrane and neuro-transmitters are released into the synapse. Synaptic vesicles from brain tissue can be purified in large amounts and proteins isolated. Real time tracking of vesicular transport Fluorescent microscopy allow real time observation of vesicular transport in living cells. Cells are transfected with cDNA encoding secretory proteins tagged with green fluorescent protein (GFP). DNA is transcribed by cellular or viral polymerase. Transcription yields mRNA. Protein is produced by the cell and targeted to the organelle via studied pathway. GFP fusion proteins allow transport vesicles carrying specific proteins to be visualized by fluorescence as they move through the secretory pathway. This method have relatively low sensitivity and GFP might disturb protein function and localization. Alternatively fluorescent antibodies could be used to visualize intercellular protein location. The process is called immunostaining. It have higher sensitivity than GFP based tracking but limited to the fixed cells. The Mechanism of Vesicular Transport Transport vesicles from the ER are coated with cytosolic coat proteins. Coats assemble as the vesicle buds off and are removed in the cytosol before it reaches its target. The vesicles fuse with the target membrane, empty their cargo, and insert their membrane proteins into the target membrane. Three families of vesicle coat proteins: COPII-coated vesicles carry proteins from the ER to the ERGIC and on to the Golgi apparatus. COPII vesicles draw their name because they are coated by Coat Protein Complex II. This type of transport is called anterograde transport. COPI-coated vesicles bud from the ERGIC (ER-Golgi intermediate compartment) or Golgi and carry their cargo back, returning proteins to earlier compartments. COPI vesicles are coated by Coat Protein Complex I. This type of transport is called retrograde transport. Clathrin-coated vesicles transport in both directions between the trans Golgi network, endosomes, lysosomes, and plasma membrane. The Mechanism of Vesicular Transport Formation of coated vesicles is regulated by small GTP- binding proteins (ARF1 and Sar1), related to Ras and Ran GTPases. GTP-binding proteins recruit adaptor proteins that mediate vesicle assembly by interacting with cargo proteins and with coat proteins. Interactions between adaptor protein and clathrin are responsible for clathring vesicles self-assembly. Human clathrin have two polypeptide chains. Heavy ~ 190kDa chain and light chain ~14 kDa chain. Heavy chains are associated by their C-termini forming triskelion. Light chains modulate heavy chain polimerization Natural curvature of clathrin lattices defines the shape of clathrin vesicles. Clathrin shells average 700–800 Å in diameter, contain about 35–40 triskelions, and enclose a spherical vesicle, ∼400 Å in diameter, somewhat eccentrically placed. See also 9e Fig 11.30 The Mechanism of Vesicular Transport light chains Triskelion is a flexible. heavy chains Assembles into pentagons and hexagons flat sheets different vesicle sizes triskelia – an ancient symbol Clathrin does not interact with cargo directly. Adaptor molecules recruit clathrin and cargo. Time Arriving to the target Fusion of a transport vesicle with its target: 1. The vesicle must recognize the correct target membrane. 2. Vesicle and target membrane must fuse, delivering the contents to the target organelle. Small GTP binding proteins called Rab proteins direct vesicles to the correct membrane. Rab proteins interact with the vesicle coat and reside on the cytoplasmic surface of the vesicle. Fusion between transport vesicles and target membranes is mediated by tethering factors and Rab proteins. Vesicle bound Rab proteins are in active GTP-bound. Rab-GTP capable to interact with a receptor protein called tethering factor. Notably, tethering factors also can bind coat proteins, and may stimulate formation of complexes between transmembrane proteins called SNAREs. see 9e 11.33 Vesicle docking and fusion SNARE are proteins that mediate vesicle fusion. They are embedded both in the target and vesicle membrane. Vesicle snares are called v-SNAREs, and target membrane SNAREs are called t-SNAREs. Formation of complexes between v-SNAREs and t-SNAREs is required for fusion. t-SNAREs guide v-SNAREs during the complex formation. SNARE proteins have a central alpha helical domain. This domain binds to other alpha helical domains with four SNARE molecules form four membered supercoil. Domain interactions zip SNAREs on vesicle and target membranes together. SNARE-SNARE pairing provides the energy to bring the two bilayers close enough to destabilize them and fuse. This brings the 2 membranes into direct contact and leads to fusion of the lipid bilayers. Lysosomes A lysosome is a membrane-bound cell organelle that contains digestive enzymes. Lysosomes are involved with various cell processes. They break down excess or worn-out cell parts. They may be used to destroy invading viruses and bacteria. They are the digestive system of the cell. They can vary in size and shape depending on the materials that have been taken up for digestion. While most eukaryotic cells carry lysosomes, yeasts (fungus) and plant cells lack lysosomes. Proteins are transported from the Golgi to the vacuole, which has the same functions as a lysosome, plus nutrient storage and maintaining turgor pressure. Proteins are directed to vacuoles by short peptide sequences. Lysosomes Lysosomes contain about 60 different degradative enzymes. Mutations in genes that encode these enzymes result in lysosomal storage diseases—undegraded material accumulates in the lysosomes of affected individuals. Gaucher disease is a genetic caused by deficiency of glucocerebrosidase, which catalyzes hydrolysis of glucosylceramide to glucose and ceramide. In the most common form of the disease, macrophages are the only cells affected. Their function is to eliminate aged and damaged cells by phagocytosis. Most lysosomal enzymes are acid hydrolases—active at pH 5 in lysosomes, but not in the cytoplasm (pH 7.2). This prevents uncontrolled digestion of cell contents if the lysosome membrane breaks down. To maintain the acidic pH, a proton pump in the lysosomal membrane actively transports protons into the lysosome. Lysosomes and Endosomes Lysosomes digest material taken up from outside the cell by endocytosis. Lysosomes are formed when transport vesicles from the trans-Golgi network fuse with a late endosome. Endosomes represent an intersection between the secretory pathway and the endocytic pathway. Three types of endosomes: Early endosomes Recycling endosomes Late endosomes Early endosomes fuse with endocytic vesicles from the plasma membrane. They separate molecules for recycling from molecules destined for degradation in lysosomes. Molecules to be recycled are passed to recycling endosomes and back to the plasma membrane. Molecules destined for degradation are transported to multivesicular bodies and then to late endosomes. Transport vesicles carrying acid hydrolases from the trans-Golgi network then fuse with late endosomes, which mature into lysosomes. See 9e 11-36 Phagocytosis and autophagy Phagocytosis: specialized cells such as macrophages take up and degrade large particles—bacteria, cell debris, aged cells. Particles are taken up in phagocytic vacuoles (phagosomes), which fuse with lysosomes to become phagolysosomes. Autophagy: turnover of the cell’s own components. It is important in embryonic development and programmed cell death. A small area of cytoplasm or organelle is enclosed in a vesicle (autophagosome) which fuses with a lysosome, and its contents are digested. Autophagy leads to continuous turnover of cellular constituents. It can also be regulated during development and in response to stress. Example: Insect metamorphosis involves extensive tissue remodeling and degradation of cellular components.