Thermodynamics Lecture Notes PDF (PHY 357) 2024-2025
Document Details
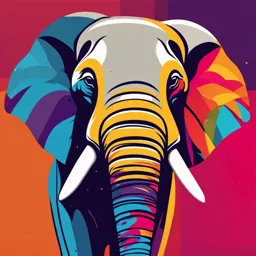
Uploaded by ComplementaryRosemary3891
2024
PHY
Dr. Essam Gamal
Tags
Summary
These lecture notes cover a university-level course in thermodynamics (PHY 357). They detail basic concepts, equations of state, kinetic theory of gases, work and the first law of thermodynamics, the second law, entropy, and thermodynamic functions. The course is for undergraduate students and the document appears to be from the year 2024-2025.
Full Transcript
THERMODYNAMICS PHY 357 املستوي الثالث شعبة علوم أساسي (إجنليزي) Dr. Essam Gamal 2024-2025 Course score distribution 1. Total Marks (100 Marks). 2. Final Exam (70 Marks). 3. Oral Exam (10 Marks). 3. Year Works (2...
THERMODYNAMICS PHY 357 املستوي الثالث شعبة علوم أساسي (إجنليزي) Dr. Essam Gamal 2024-2025 Course score distribution 1. Total Marks (100 Marks). 2. Final Exam (70 Marks). 3. Oral Exam (10 Marks). 3. Year Works (20 Marks). 3.1. Mid Term Exam (8 Marks). 3.2. Assignments (12 Marks): 3.2.1. Quizzes. 3.2.2. Homework. 3.2.3. Report. 3.2.4. Attendance. COURSE CONTENT املستوي الثالث شعبة علوم أساسي (إجنليزي) Dr. Essam Gamal 2024-2025 Chapter One: Basic Concepts and Principles o What is thermodynamics? o Definitions of some terms used in thermodynamics (system, medium, state, etc.). o Dimensions and units. o Thermodynamic systems. o Heat, temperature, heat quantity and internal energy of a system. o Thermodynamic equilibrium. o Zeroth law of thermodynamics. o Latent heat of fusion and vaporization. o Temperature scales and thermal scales. o Heat capacity and specific heat capacity. o Examples and problems. Chapter Two: Equations of State and Kinetic Theory of Gases o Gas laws. o Thermodynamic coordinates. o Equation of state. o Gas parameters. o Relation between gas parameters. o Examples and problems. o Equation of state for an ideal gas. o Equation of state for a real gas. o Examples and problems. o Kinetic theory of pressure and temperature of an ideal gas. o Examples and problems. Chapter Three: Work and the First Law of Thermodynamics o Reversible and irreversible processes. o Specific heat of an ideal gas. o Microscopic and macroscopic properties. o Relation between specific heats at constant volume and at constant pressure o Quasistatic processes. and the universal constant for gases. o Thermodynamic processes. o Ratio between specific heats of a gas at o Work done in the expansion or contraction constant pressure and at constant of an ideal gas in quasistatic processes. volume. o Work depends on the path. o Equations of state for an ideal gas in a o Examples and problems. state of adiabatic change. o Internal energy. o Work done by a gas expanding o Heat transfer. adiabatically from an initial volume to a final volume. o First law of thermodynamics. o Elasticity of isothermal and insulated o Enthalpy. gases. o Examples and problems. Chapter Four: The Second Law of Thermodynamics o Formulas of the second law of thermodynamics. o Conversion of work to heat and vice versa and thermal efficiency. o Thermal machine (heat engine). o Carnot cycle for the ideal heat machine. o Efficiency of the Carnot cycle as a function of temperature. o Inverse Carnot cycle. o Electric refrigerator. o Coefficient of performance for the inverse Carnot cycle. o Calculating the algebraic sum of the ratio between the amount of heat and the temperature through the isothermal curve in the Carnot cycle. o The relation between the efficiency of the heat machine and the coefficient of performance of the cooler. o Applications on the Carnot cycle. o Examples and problems. Chapter Five: Entropy o Clausius Theorem. o Entropy and formulating the second law of thermodynamics. o Physical meaning of entropy. o Principle of increasing entropy. o Work done during the Carnot cycle as a function of temperature difference and entropy difference. o Change in entropy of an ideal gas as a function of specific heat of gas at constant volume. o Change in entropy of an ideal gas as a function of specific heat of gas at constant pressure. o Entropy changes in reversible processes. o Entropy of ice and steam at different temperatures. o Examples and problems. Chapter 6: Thermodynamic Functions o Internal energy function. o Enthalpy (heat content) function. o Free energy (Helmholtz function). o Thermodynamic potential (Gibbs function). o Maxwell’s equations. o Derivation of 𝑻𝒅𝒔 equations. CHAPTER ONE BASIC CONCEPTS OF THERMODYNAMICS املستوي الثالث )شعبة علوم أساسي (إجنليزي Dr. Essam Gamal LECTURE ONE Thermodynamics: The name thermodynamics stems from the Greek words thermo (heat) and dynamis (power). Thermodynamics can be defined as the science of energy and its transformations, including power production, refrigeration, and relationships among the properties of matter. The system and the surrounding: Thermodynamic system: is defined as a quantity of matter (solid, liquid or gas) or a region in space chosen for study. Surrounding: is the mass or region outside the thermodynamic system. System boundary: is the imaginary envelope that encloses the system and separates it from the surrounding medium. Types of thermodynamics systems: Non-isolated system There is exchange of energy (heat), or matter, or both between the system and the surrounding medium. Open system: It is a system that allows the exchange of both matter and energy between it and the surrounding medium. Therefore, these systems do not verify the laws of conservation of matter and energy. Closed system: It is a system that allows the exchange of energy only between it and the surrounding medium in the form of heat or work while, no matter exchange can take place. Therefore, these systems do not verify the conservation law of energy. Isolated system It is a system that does not allow the exchange of both energy and matter between it and the surrounding medium. Therefore, these systems verify the law of conservation of matter and energy. Quiz Select any of the following systems (open, closed, or isolated) and mention the reason for your choice. Initial Final Initial mass Final mass system temperature temperature 𝒎𝒊 𝑚𝒇 𝑻𝒊 𝑇𝒇 A 𝟎. 𝟎𝟓 𝒌𝒈 𝟓𝟎 𝐠 𝟐𝟕 ℃ 𝟑𝟎𝟎 𝐊 B 𝟏𝟎𝟎 𝒈 𝟎. 𝟏 𝐤𝐠 𝟑𝟏𝟎 𝑲 𝟐𝟗𝟎 𝐊 C 𝟎. 𝟕 𝒌𝒈 𝟎. 𝟎𝟕 𝐤𝐠 𝟒𝟏𝟎 𝑲 𝟏𝟎𝟎 ℃ Thermal equilibrium: It means that the temperature is the same throughout the entire system. The State: It is the set of macroscopic properties associated with the system, such as volume 𝑽, pressure 𝑷, temperature 𝑻, internal energy 𝑼, and others. The Process: Any change in the thermodynamic coordinates of a system is called a process. Or it is any change that a system undergoes from one equilibrium state to another. Dimensions and Units: In this course, we will use the International System of Units (SI) and the following table shows the basic quantities: Dimensions and Units: We will use the International System of Units (SI) and the basic quantities are: Physical quantity symbol Measuring unit Length (distance) L Meter (m) Mass M Kilogram (kg) Time t Second (s) Absolute temperature T Kelvin (K) Current intensity I Ampere (A) Material quantity m gram molecule (mol) Illumination intensity J Candela (cd) We must mention that it is necessary to use absolute temperatures in thermodynamics. Amount of substance in the system: “It is the number of molecular grams (moles) in the system”. The molecular gram is represented by the symbol (mol), and therefore: mol: “It is the molecular mass of matter 𝑴 expressed in grams or kg”. 1 mol of oxygen gas (O2) = 0.032 kg. 1 mol of nitrogen gas (N2) = 0.028 kg. In the case of most gases at normal temperatures, 1 mol contains a constant number of atoms or molecules equal to 𝟔. 𝟎𝟐 × 𝟏𝟎𝟐𝟑 , called Avogadro’s number 𝑵𝑨. Avogadro’s number: “It is the number of atoms or molecules contained in one mole of the substance, and it is equal to 𝟔. 𝟎𝟐 × 𝟏𝟎𝟐𝟑 atom or molecule”. Molar volume: “It is the volume occupied by 1 mol of matter” and its unit is (𝒎𝟑 /𝒎𝒐𝒍). Molar density: "It is the reciprocal of the molecular volume" and its unit is (𝒎𝒐𝒍/𝒎𝟑). In addition to the basic quantities, there are many other derived quantities whose units are derived from the basic units. Speed, force, pressure, energy and power are examples of these derived dimensions that are used in thermodynamics. 𝑭𝒐𝒓𝒄𝒆 (𝑭) = 𝒎𝒂𝒔𝒔 (𝒎) × 𝒂𝒄𝒄𝒆𝒍𝒆𝒓𝒂𝒕𝒊𝒐𝒏 𝒂 = 𝒌𝒈. 𝒎/𝒔𝟐 (𝑵) Pressure (P): “It is the force acting perpendicularly on a unit area”. 𝑭 𝒌𝒈.𝒎 𝑷= = = 𝒌𝒈Τ𝒎. 𝒔𝟐 (𝑵/𝒎𝟐 𝒐𝒓 𝑷𝒂𝒔𝒄𝒂𝒍) 𝑨 𝒔𝟐.𝒎𝟐 𝟏 𝑷𝒂 = 𝟏 𝑵Τ𝒎𝟐 = 𝟏 𝒌𝒈Τ𝒎. 𝒔𝟐 The quantity of heat (𝑸): “It is the energy that flows automatically from a hot body to a cold body”. 𝑸 = 𝒎 𝒔 ∆𝑻 𝑱𝒐𝒖𝒍 𝒐𝒓 𝒄𝒂𝒍. where 𝒎 is the mass of the body, 𝒔 is the specific heat capacity of the body and, ∆𝑻 is the change in temperature. ∆𝑻 = 𝑻𝒇 − 𝑻𝒊 where𝑻𝒊 and 𝑻𝒇 are the initial and final temperature of the body, respectively. Calorie (cal): “It is the amount of heat needed to raise or lower the temperature of 1 gm of water by 𝟏 ℃ from 𝟏𝟒. 𝟓 ℃ to 𝟏𝟓. 𝟓 ℃”. 𝟏 𝒄𝒂𝒍 = 𝟒. 𝟏𝟖 𝑱 Heat, temperature and thermal equilibrium: Heat: “It is the energy that is transferred between two systems when there is a difference in their temperature, and it is always transferred from the body with the higher temperature to the other body with the lower temperature”. When two bodies (systems), one hot and the other cold, are placed in direct contact, the hot body loses heat, meaning its temperature decreases, while the other body gains heat, so its temperature rises. This process continues until the two bodies reach an equal temperature (the same temperature). Here, heat is transferred automatically without resorting to a means of pumping it, and it is said that the two bodies are in a state of thermal equilibrium. Temperature: “It is the property by which a system can be identified in a state of thermal equilibrium or thermal disequilibrium with the surrounding”. Zeroth Law of Thermodynamics (Thermal Equilibrium Law): States that “if two systems (A) and (B) are in thermal equilibrium with a third system (C), they are also in thermal equilibrium with each other”. The relationship between heat, internal energy and temperature ❑ When the system gains thermal energy as a result of a heat exchange between it and the surrounding (or from another system), the amplitude of vibration of the system’s molecules increases, so the kinetic energy increases and the internal energy of the system increases, and this appears in the form of an increase in the temperature of the system, and vice versa. ❑ When the system loses thermal energy as a result of a heat exchange between it and the surrounding environment (or from another system), the amplitude of vibration of the system’s molecules decreases, so the kinetic energy decreases and the internal energy of the system decreases, and this appears in the form of a decrease in the temperature of the system. The internal energy of the system (𝑼): “It is the sum of the energies stored in matter, such as kinetic and potential energy and other energies”. Latent heat (L): “It is the amount of heat required to convert 1 g of a substance from one state to another at a constant temperature”. 𝑸 𝑳= 𝒄𝒂𝒍/𝒈 𝒐𝒓 𝑱/𝒌𝒈 𝒎 Latent heat of fusion (Lf): “It is the amount of heat required to convert 1 g of a substance from the solid state to the liquid state at constant temperature”. 𝑸 𝑳𝒇 = 𝒄𝒂𝒍/𝒈 𝒐𝒓 𝑱/𝒌𝒈 𝒎 Latent heat of sublimation or vaporization (Lv): “It is the amount of heat required to convert 1 g of a substance from the liquid state to the gaseous (vapor) state at constant temperature”. 𝑸 𝑳𝒗 = 𝒄𝒂𝒍/𝒈 𝒐𝒓 𝑱/𝒌𝒈 𝒎 Measuring temperature - thermometric material and thermometric property: Thermometric Thermometric Thermometer The law material property It is a liquid placed in a Change in the length of 𝑳𝒕 − 𝑳𝒐 capillary tube, such as an ❶ Liquid Thermometer the liquid column (𝑳) with 𝒕 = 𝟏𝟎𝟎 alcohol or mercury 𝑳𝟏𝟎𝟎 − 𝑳𝒐 temperature (𝑻); (𝑳 ∝ 𝑻). thermometer. ❷ Gas Thermometer It is a gas placed in a Change in the pressure of 𝑷𝒕 − 𝑷𝒐 𝒕 = 𝟏𝟎𝟎 (a) Constant-Volume container at a constant the gas ( 𝑷 ) with 𝑷𝟏𝟎𝟎 − 𝑷𝒐 volume. temperature (𝑻); (𝑷 ∝ 𝑻). It is a gas placed in a Change in the volume of 𝑽𝒕 − 𝑽𝒐 (b) Constant-Pressure container at a constant the gas ( 𝑽 ) with 𝒕 = 𝟏𝟎𝟎 𝑽𝟏𝟎𝟎 − 𝑽𝒐 pressure. temperature (𝑻); (𝑽 ∝ 𝑻). Change in the resistance 𝑹𝒕 − 𝑹𝒐 It is a coil resistance made of ❸ Platinum Thermometer platinum wire. of the wire ( 𝑹 ) with 𝒕 = 𝟏𝟎𝟎 𝑹𝟏𝟎𝟎 − 𝑹𝒐 temperature (𝑻); (𝑹 ∝ 𝑻). General law of thermometers: 𝒕−0 𝑿𝒕 − 𝑿𝒐 = 100 − 0 𝑿100 − 𝑿𝒐 𝑿𝒕 − 𝑿𝒐 𝒕 = 100 𝑿100 − 𝑿𝒐 Where: 𝑿𝒐 is the thermometric property at 𝟎 ℃. 𝑿𝟏𝟎𝟎 is the thermometric property at 𝟏𝟎𝟎 ℃. 𝑿𝒕 is the thermometric property at 𝒕 ℃. ❶ Liquid Thermometer 𝑿𝒕 − 𝑿𝒐 𝒕 = 100 ❸ Platinum thermometer 𝑳𝒕 − 𝑳𝒐 𝑿100 − 𝑿𝒐 𝒕 = 100 𝑹𝒕 − 𝑹𝒐 𝑳100 − 𝑳𝒐 𝒕 = 100 𝑹100 − 𝑹𝒐 Where: 𝑳𝒐 is the length of the column of mercury at 𝟎 ℃. Where: 𝑳𝟏𝟎𝟎 is the length of the column of mercury at 𝟏𝟎𝟎 ℃. 𝑹𝒐 is the resistance of the coil at 𝟎 ℃. 𝑳𝒕 is the length of the column of mercury at 𝒕 ℃. 𝑹𝟏𝟎𝟎 is the resistance of the coil at 𝟏𝟎𝟎 ℃. 𝑹𝒕 is the resistance of the coil at 𝒕 ℃. ❷ Gas thermometer (a) Constant volume (b) Constant pressure 𝑷𝒕 − 𝑷𝒐 𝑽𝒕 − 𝑽𝒐 𝒕 = 100 𝒕 = 100 𝑷100 − 𝑷𝒐 𝑽100 − 𝑽𝒐 Where: Where: 𝑻𝑲 = 𝑻𝑪 + 𝟐𝟕𝟑 𝑷𝒐 is the gas pressure at 𝟎 ℃. 𝑽𝒐 is the gas volume at 𝟎 ℃. 𝑷𝟏𝟎𝟎 is the gas pressure at 𝟏𝟎𝟎 ℃. 𝑽𝟏𝟎𝟎 is the gas volume at 𝟏𝟎𝟎 ℃. 𝑷𝒕 is the gas pressure at 𝒕 ℃. 𝑽𝒕 is the gas volume at 𝒕 ℃.