Lecture 1 Genomics and Proteomics PDF
Document Details
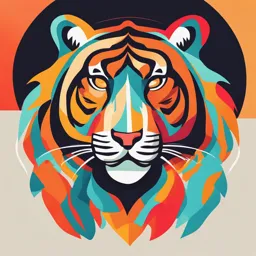
Uploaded by ComelyChrysanthemum5045
New Mansoura University
Tags
Summary
This document provides an introduction to genomics and proteomics, outlining the study of complete sets of molecules within biological systems. It details various techniques and methodologies used in the field, such as DNA sequencing and RNA sequencing. The information explores the roles of genomics, transcriptomics, proteomics, and metabolomics in understanding gene functions, metabolic pathways, and cellular processes.
Full Transcript
GENOMICS AND PROTEOMICS Lecture # 1 INTRODUCTION The suffex OMIC refers to the study of the complete set of molecules within a biological system, including genomics, transcriptomics, proteomics, and metabolomics to identify genetic factors and metabolic pathways that play...
GENOMICS AND PROTEOMICS Lecture # 1 INTRODUCTION The suffex OMIC refers to the study of the complete set of molecules within a biological system, including genomics, transcriptomics, proteomics, and metabolomics to identify genetic factors and metabolic pathways that play important roles in the growth and development of biological systems that would be affected by environmental conditions, diseases ------etc. By integrating multiple OMIC datasets, researchers can gain a more comprehensive understanding of the molecular mechanisms underlying living cells growth and development as well as responses to environmental stresses. OMIC approaches involve the comprehensive analysis of various biological molecules, including DNA, RNA, proteins, and metabolites, to gain insights into the molecular mechanisms underlying cell growth and development. These approaches have revolutionized the field of cell biology by enabling the simultaneous analysis of multiple molecules and providing a systems-level understanding of cell processes. - Genomics involves the study of the entire genome of an organism, including the identification of genes and their functions. The availability of genome sequences for several organisms enabled the identification of key genes and pathways associated with important traits. Studying the genome involves a range of techniques that allow researchers to analyze the DNA content of an organism. Some common techniques used in genome studies include: 1. DNA Sequencing: 1 o Sanger Sequencing: The traditional method for sequencing DNA. o Next-Generation Sequencing (NGS): High-throughput sequencing technologies that enable rapid sequencing of large amounts of DNA. 2. Genome Assembly: o De Novo Assembly: Building a genome sequence from scratch without a reference genome. o Reference-Based Assembly: Using a known reference genome to align and assemble sequenced reads. 3. Genome Annotation: o Identification of Genes and Functional Elements: Tools like GeneMark, AUGUSTUS, and BLAST are used to predict genes and annotate functional elements. 4. Comparative Genomics: o Whole-Genome Alignment: Comparing genomes to identify similarities and differences. o Synteny Analysis: Studying conserved genomic arrangements across species. 5. Metagenomics: o Analysis of Microbial Communities: Studying the genomes of microbial communities in environmental samples. 6. Functional Genomics: o Gene Knockout/Knockdown: Disrupting gene function to study its effects. 7. Phylogenomics: o Phylogenetic Analysis: Studying evolutionary relationships using genomic data. 2 These techniques, along with advancements in bioinformatics and computational biology, help researchers gain insights into the functions of genes, regulatory elements, and pathways within the genome. - Transcriptomics involves the analysis of the entire transcriptome of an organism, including the identification of all the expressed genes and their levels of expression. Transcriptomics has been used to identify genes and pathways associated with particular diseases and/or environmental conditions. Transcriptomics focuses on studying RNA expression patterns to understand gene expression levels, alternative splicing, and regulatory mechanisms within cells or tissues. Various techniques are employed in transcriptomic analysis, some of which include: 1. RNA Sequencing (RNA-Seq): o Quantification of Gene Expression: Measures the abundance of RNA transcripts in a sample. o Identification of Alternative Splicing Events: Detects different isoforms of genes. 2. Microarray Analysis: o Gene Expression Profiling: Measures the expression levels of a large number of genes simultaneously. o Comparative Expression Studies: Compares gene expression across different conditions or tissues. 3. Quantitative Real-Time PCR (qRT-PCR): o Validation of Gene Expression: Confirms gene expression patterns observed in RNA-Seq or microarray studies. These techniques help researchers gain insights into the dynamic landscape of gene expression and regulation, providing valuable information about cellular processes, developmental pathways, and disease mechanisms. 3 - Proteomics involves the study of the entire proteome of an organism, including the identification of all the proteins and their functions. Proteomics has been used to identify proteins associated with stress response. Proteomics is the study of the entire set of proteins expressed by a cell, tissue, or organism. Proteomic analysis involves a variety of techniques to study protein structure, function, and interactions. Some common techniques used in proteomic analysis include: 1. Mass Spectrometry (MS): o Liquid Chromatography-Mass Spectrometry (LC-MS): Separates and identifies proteins based on mass-to-charge ratios. o Tandem Mass Spectrometry (MS/MS): Fragmentation of peptides for sequencing and identification. 2. Two-Dimensional Gel Electrophoresis (2D-PAGE): o Separation of Proteins: Separates proteins based on isoelectric point and molecular weight for analysis. 3. Protein Identification: o Database Search: Matches experimental mass spectra to known protein sequences for identification. o De Novo Sequencing: Determines protein sequences directly from mass spectra without a reference database. 4. Protein Structure Analysis: o X-Ray Crystallography and NMR Spectroscopy: Techniques used to determine protein structures at atomic resolution. 5. Bioinformatics Analysis: 4 o Database Search Tools: Analyze and interpret proteomic data for functional insights and pathway analysis. These techniques play a crucial role in understanding protein expression, structure, function, interactions, and post-translational modifications, providing valuable insights into cellular processes, diseases, and biological systems. - Metabolomics involves the study of the entire metabolome of an organism, including the identification of all the metabolites and their functions. Metabolomics has been used to identify metabolites associated with particular diseases and/or environmental conditions. Metabolomics is the study of small molecules, known as metabolites, within cells, tissues, or organisms. It aims to understand the metabolic processes and pathways in biological systems. Various techniques are used in metabolomic analysis to identify and quantify metabolites. Some common techniques include: 1. Mass Spectrometry (MS): o Gas Chromatography-Mass Spectrometry (GC-MS): Analyzes volatile and thermally stable compounds. o Liquid Chromatography-Mass Spectrometry (LC-MS): Separates and identifies metabolites based on mass-to-charge ratios. o Tandem Mass Spectrometry (MS/MS): Fragmentation of metabolites for structural elucidation. 2. Nuclear Magnetic Resonance (NMR) Spectroscopy: o High-Resolution Analysis: Provides information on the chemical structure and concentration of metabolites. 3. Targeted Metabolomics: 5 o Quantification of Specific Metabolites: Focuses on measuring a predefined set of metabolites with known biological relevance. 4. Isotope Tracing: o Stable Isotope Labeling: Tracks the fate of labeled metabolites through metabolic pathways to study fluxes and metabolic transformations. These techniques help researchers unravel the metabolic profiles of biological systems, providing insights into physiological conditions, disease mechanisms, and responses to external stimuli such as drugs or environmental factors. - The term epigenome is derived from the Greek word epi which literally means "above" the genome. The epigenome consists of chemical compounds that modify, or mark, the genome in a way that tells it what to do, where to do it, and when to do it. Different cells have different epigenetic marks. These epigenetic marks, which are not part of the DNA itself, can be passed on from cell to cell as cells divide, and from one generation to the next. The epigenome is the collection of all of the epigenetic marks on the DNA in a single cell. The epigenomic marks different between different cell types. So, a blood cell will have different marks or modifications than a liver cell. The epigenomic modifications, the whole collection of all of the epigenetic marks on my blood cell DNA should be more similar to all of the marks on your blood cell DNA than to the collection of all the marks on my liver cell DNA. So this is a way of defining a particular type of cell. Now due to individual differences my epigenome will differ from your epigenome even in the same tissue. It's those differences that make us all individuals, and we'll see even greater changes in a state of disease. So a comparison 6 of a normal cell and all of its epigenetic marks, or the epigenome of that cell, will differ from the diseased state of that same cell type. And we can use these differences to figure out mechanisms of disease. 7 The first part of our sylbus, GENOMICS Genome nature in different types of cells Living organisms are divided according to their cellular structure into prokaryotes and eukaryotes. Prokaryotes include bacteria, archaea, and eukaryotes contain the rest of the groups of living organisms starting from animal protists such as amoebas, paramecium, algae, fungi, plants and various animals. The main difference between prokaryotes and eukaryotes is due to the presence of a true nucleus surrounded by a nuclear membrane in the case of eukaryotic organisms. As for prokaryotes, their genetic material spreads in the cytoplasm. Figure 2 shows the difference between prokaryotes and eukaryotes. At least 2 types of genomes are available in eukaryotic cells (nuclear and mitochondrial genomes). In case of photosynthetic eukaryotes, three types of Genomes might be available (nuclear, mitochondrial and chloroplast genomes). Both mitochondria and chloroplast have small genomes, like that of bacteria and archaea genome. Figure 1: Left shape: The differences between Eukaryotic and prokaryotic cells, Right shape: The types of DNA inside typical eukaryotic photosynthetic organism 8 Watson and Crick MODEL Although scientists have made some minor changes to the Watson and Crick model, or have elaborated upon it, since its inception in 1953, the model's four major features remain the same yet today. These features are as follows: DNA is a double-stranded helix, with the two strands connected by hydrogen bonds. A bases are always paired with Ts, and Cs are always paired with Gs, which is consistent with and accounts for Chargaff's rule. Most DNA double helices are right-handed; that is, if you were to hold your right hand out, with your thumb pointed up and your fingers curled around your thumb, your thumb would represent the axis of the helix and your fingers would represent the sugar-phosphate backbone. Only one type of DNA, called Z-DNA, is left-handed. The DNA double helix is anti-parallel, which means that the 5' end of one strand is paired with the 3' end of its complementary strand (and vice versa). As shown in Figure 4, nucleotides are linked to each other by their phosphate groups, which bind the 3' end of one sugar to the 5' end of the next sugar. Not only are the DNA base pairs connected via hydrogen bonding, but the outer edges of the nitrogen-containing bases are exposed and available for potential hydrogen bonding as well. These hydrogen bonds provide easy access to the DNA for other molecules, including the proteins that play vital roles in the replication and expression of DNA (Figure 2). 9 Figure 2: Base pairing in DNA. Two hydrogen bonds connect T to A; three hydrogen bonds connect G to C. The sugar-phosphate backbones (grey) run anti-parallel to each other, so that the 3’ and 5’ ends of the two strands are aligned. One of the ways that scientists have elaborated on Watson and Crick's model is through the identification of three different conformations of the DNA double helix. In other words, the precise geometries and dimensions of the double helix can vary. The most common conformation in most living cells (which is the one depicted in most diagrams of the double helix, and the one proposed by Watson and Crick) is known as B-DNA. There are also two other conformations: A-DNA, a shorter and wider form that has been found in dehydrated samples of DNA and rarely under normal physiological circumstances; and Z-DNA, a left-handed conformation. Z- DNA is a transient form of DNA, only occasionally existing in response to certain types of biological activity (Figure 3). Z-DNA was first discovered in 1979, but its existence was largely ignored until recently. Scientists have since discovered that 10 certain proteins bind very strongly to Z-DNA, suggesting that Z-DNA plays an important biological role in protection against viral disease (Rich & Zhang, 2003). Figure 3: Three different conformations of the DNA double helix. (A) A-DNA is a short, wide, right-handed helix. (B) B-DNA, the structure proposed by Watson and Crick, is the most common conformation in most living cells. (C) Z-DNA, unlike A- and B- DNA, is a left-handed helix. 11 12