Enzyme Mechanisms Lecture Notes PDF
Document Details
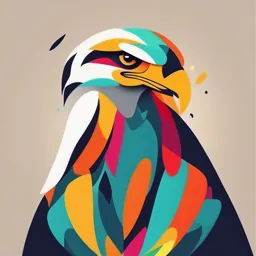
Uploaded by SimplerFoxglove
University of Minnesota
Robert Roon
Tags
Summary
These lecture notes cover the topic of enzyme mechanisms, detailing the chemical processes used for catalysis. The notes include explanations of different enzyme reaction mechanisms and their effects on reaction rates.
Full Transcript
BioC 3021 Notes Robert Roon Lecture 8: Enzyme Mechanisms Slide 1. Enzyme Mechanisms The topic of this lecture is enzyme mechanisms. This material gets at the heart of enzyme catalysis. We will take a detailed look at the chemical processes that an enzyme us...
BioC 3021 Notes Robert Roon Lecture 8: Enzyme Mechanisms Slide 1. Enzyme Mechanisms The topic of this lecture is enzyme mechanisms. This material gets at the heart of enzyme catalysis. We will take a detailed look at the chemical processes that an enzyme uses to effect catalysis. Slide 2. How Enzymes Increase Reaction Rates On an exam question asking how an enzyme can carry out catalysis, the typical answer is that, “Enzymes form an ES complex and provide an alternate reaction pathway with a lower energy of activation.” That answer is only partially correct, and it does not get to the heart of the question. So if you give me that answer on an exam you will get only partial (low) credit. The real answer is that there are a number of chemical mechanisms that an enzyme provides that collectively result in significant catalysis. We will look at those mechanisms in this lecture. Slide 3. Enzyme Reaction Mechanisms Here is a list of the most significant ways in which enzymes carry out catalysis. The mechanisms include: -Proximity and Orientation Effects -General Acid and General Base Catalysis -Electrostatic Effects -Nucleophilic and Electrophilic Catalysis -Structural Flexibility -Entropy Loss -Desolvation -Covalent Catalysis 1 BioC 3021 Notes Robert Roon Slide 4. Catalysis by Proximity and Orientation Enzymes bind substrates in close proximity to each other and in the correct orientation so that a reaction may occur without the need for a random collision between reactants. This reduces the entropy of the reactants, making ligation or addition reactions more favorable. It also reduces the overall loss of entropy when two reactants become a single product. This concentration of the substrates in the active site simulates the effect of increased substrate concentrations and gives the reactions a pseudo intramolecular character. Slide 5. Proximity Effects The chemical example of succinic anhydride formation shows how having two reacting groups in proximity to each other can facilitate a reaction. Instead of waiting for a random collision to bring two carboxylic acid groups together, the proximity of the two carboxylate groups in succinate facilitates their reaction. Slide 6. Rate Enhancements Due to Proximity and Orientation This figure shows the rate enhancements for proximity effects during the formation of carboxylic anhydrides in a chemical reaction using constrained substrates. By placing the reacting groups in the correct proximity and orientation, the chemical reaction can be enhanced by a factor of 108. Slide 7. General Acid Base Catalysis An enzyme can act as a proton donor (Acid) and a proton acceptor (Base) to promote rapid reaction. In fact, the same enzyme can simultaneously function as both an acid and a base. The substitution of enzyme functional groups for hydrogen ions and hydroxyl groups permits rapid enzyme catalysis at neutral pH. Acid Base catalysis often involves the histidine imidazole group because it has an appropriate pK that allows it to act as an acid or base at neutral pH. 2 BioC 3021 Notes Robert Roon Slide 8. Ester Hydrolysis Can Be Facilitated by Acid Base Catalysis Panel “a” shows the chemical hydrolysis of an ester at neutral pH. This reaction is slow for a number of reasons: -It depends of the availability of a hydroxyl ion, which at pH 7.0 is present at 10-7 M. -It involves an anionic transition state with a minus charge on an oxygen atom. Formation of this anionic intermediate involves a very high energy of activation. -It uses water as a source of a proton for the cleavage of an –OR group. This is much slower than the direct reaction with a free proton. Panel “b” shows the same reaction in the presence of a base (B-). The reaction is facilitated because the base strips a proton from water and thus provides a hydroxyl ion much faster. However, because the amount of hydroxyl ion is higher in a basic solution, the hydrogen ion (proton) concentration will be lower, so reaction components that require acid catalysis will be slowed. Panel “c” shows the same reaction in the presence of an acid (HA). The acid might help stabilize the anionic transition state by neutralizing the minus charge on the oxygen atom. It might also provide a proton for the cleavage of the –OR group. However, because the hydrogen ion is higher in an acid solution, the hydroxyl ion concentration will be lower, so reaction components that require base catalysis will be slowed. The genius of enzyme catalysis is that an enzyme can provide both high acid and high base equivalents at neutral pH. The enzyme provides both hydroxyl ions and hydrogen ions at the exact place that they are needed to facilitate catalysis. The simultaneous availability of both components results in a marked enhancement in the rate of ester hydrolysis. 3 BioC 3021 Notes Robert Roon Slide 9. Covalent Catalysis Covalent catalysis involves the substrate forming a transient covalent bond with an amino acid R-group in the active site of an enzyme. The formation of a covalent intermediate can substitute for the nucleophilic attack by a hydroxyl group, and can also reduce the energy of later transition states of the reaction. The covalent bond must, at a later stage in the reaction, be broken to regenerate the enzyme. This type of covalent catalysis mechanism is found in proteolytic enzymes such as chymotrypsin and trypsin, where an acyl-enzyme intermediate is formed. Slide 10. Serine Proteases Form Covalent Acyl-Enzyme Intermediates In hydrolysis reactions, there is often a nucleophilic attack of a free hydroxyl group on a carbonyl carbon. In certain serine proteases such as chymotrypsin, an active site serine (hydroxyl) group substitutes for a free hydroxyl group, resulting in a covalent enzyme-substrate (acyl-enzyme ester) intermediate. Since the serine hydroxyl is always present at exactly the right place in the active site of the enzyme, the rate of reaction is increased. Slide 11. Covalent Schiff Base Formation in Acetoacetate Decarboxylase The formation of a positively charged covalent intermediate (Schiff Base) between enzyme and substrate promotes the movement of electrons that is needed for the decarboxylation of acetoacetate. That is, the enzyme provides an alternate transition state in which there is no minus charge on an anionic oxygen atom. This alternate pathway of reaction results in a lower energy of activation. 4 BioC 3021 Notes Robert Roon Slide 12. Covalent Catalysis with Cofactors Some enzymes utilize non-protein acid cofactors such as pyridoxal phosphate (PLP) or thiamine pyrophosphate (TPP) to form covalent intermediates with reactant molecules. Such covalent intermediates function to reduce the energy of the reaction transition state. This is similar to the use of covalent intermediates with active site amino acid residues. The ability of cofactors to delocalize electrons allows enzymes to carry out reactions that amino acid side residues alone could not accomplish. Enzymes utilizing covalent intermediates with cofactors include the PLP-dependent enzyme aspartate transaminase and the TPP- dependent enzyme pyruvate dehydrogenase. Slide 13. Covalent Catalysis with Pyridoxal Phosphate This reaction mechanism involving pyridoxal phosphate occurs during the transamination of amino acids. In this mechanism, a covalent intermediate is formed between the amino acid substrate and the pyridoxal cofactor. The association with the pyridoxal cofactor provides an alternate transition state intermediate in place of an anionic species that would form in the uncatalyzed reaction. Slide 14. Entropy Loss Binding to the enzyme reduces random tumbling and stretching of the substrate. This is a low-entropy state that favors reaction of the ES complex. Slide 15. Desolvation Substrates often have water tightly bound to their surface. Binding to the enzyme can release this water and make the substrate more reactive. Slide 16. Electrostatic Destabilization If repulsive charges occur between substrate and enzyme and these repulsive forces can be relieved during reaction, the reaction rate 5 BioC 3021 Notes Robert Roon may be accelerated forward. Slide 17. Enzyme Complementary to Substrate Enzymes generally bind their substrates fairly tightly, and it is sometimes suggested that this plays a major role in lowering the energy of activation of an enzymatic reaction. However, the occurrence of a very tight binding association between substrate and enzyme would actually place the ES complex in an energy well, making the energy of activation higher than for the uncatalyzed reaction. Slide 18. Enzyme Complementary to Transition State The current working hypothesis for enzymatic catalysis is that the tightest binding may occur between enzyme and transition state intermediates. This would effectively lower the energy of activation and speed the reaction rate. Slide 19. Energy of Activation for Catalyzed and Uncatalyzed Reactions The figure shows the reaction pathway for catalyzed and uncatalyzed reactions. The uncatalyzed reaction has a relatively high energy of activation. The uncatalyzed reaction has a slightly lowered energy for the ES complex, and it also has a significantly lower energy level for the transition state. The important feature is that the overall energy of activation is much lower for the catalyzed reaction. This would not be possible if the ES complex was at an extremely low energy level. Slide 20. Mechanism of Chymotrypsin We will now study the enzyme chymotrypsin, which uses some of the catalytic functions we have just discussed. Chymotrypsin is a digestive enzyme that catalyzes proteolysis of peptide bonds. The enzyme preferentially cleaves peptide bonds on the carboxyl side of tyrosine, tryptophan, or phenylalanine. These amino acids contain aromatic rings in their side chain. During catalysis, these 6 BioC 3021 Notes Robert Roon aromatic rings fit into a ‘hydrophobic pocket’ of the enzyme. Chymotrypsin cleaves peptide bonds by attacking the peptide carbonyl group with a powerful nucleophile, the serine 195 residue located in the active site of the enzyme. The active site serine residue briefly becomes covalently bonded to the substrate, forming an enzyme-substrate intermediate. Slide 21. Chemical cleavage of a Peptide Bond This figure shows the mechanism of peptide bond cleavage. This is a hydrolytic reaction that uses the elements of water to break a peptide bond and form a carboxyl group and an amino group. The reaction equilibrium is highly in favor of peptide bond cleavage. Slide 22. A Peptide Containing Phenylalanine This peptide contains the amino acid phenylalanine (Phe). Chymotrypsin would cleave this peptide to the right of the phenylalanine residue. It would cleave the bond shown in red between the carbonyl group of phenylalanine and the amino group of asparagine (Asn). Slide 23. Reaction of the Active Site Serine 195 with DIFP Chymotrypsin is a serine protease. Its active site has a uniquely active serine hydroxyl group (serine 195) that can lose a proton and become anionic during the course of catalysis. That property makes the active site serine residue highly susceptible to reaction with the reagent DIFP. This reaction produces a covalent product and inactivates the enzyme. Slide 24. Hydrolysis of Amides or Esters by Chymotrypsin This figure gives a generalized scheme for the enzymatic hydrolysis of amides or esters in reactions involving active site serine residues. There is an acylation phase in which the alcohol or amine is released and the active site serine is acylated. That is followed by a deacylation phase in which the carboxylic acid component is released from the enzyme. The intermediate in this 7 BioC 3021 Notes Robert Roon process is an acylenzyme intermediate in which the carboxylic acid portion of the substrate is covalently bound to the surface of the enzyme. Slide 25. Catalytic Triad in the Active Site of Chymotrypsin The active site of chymotrypsin contains a catalytic triad, consisting of the amino acids His 57, Asp 102, and Ser 195. These three amino acids are especially important in catalysis. Hydrogen ions are passed back and forth between these three amino acids. That sharing of protons has the net effect of stabilizing the serine hydroxyl group in the negative anionic state. Note that these active site amino acids come from amino acid residues that are spatially separated in the primary sequence of the enzyme. Slide 26. The Reaction Mechanism of Chymotrypsin This figure shows the overall mechanism of the reaction catalyzed by chymotrypsin. Hydrolysis of an amide substrate involves the participation of the catalytic triad, the formation of a covalent acylenzyme intermediate, and the transient existence of various tetrahedral transition state intermediates. Slide 27. Chymotrypsin Mechanism Step 1 In step 1 of the reaction, there is a nucleophylic attack by the active site serine on the carbonyl group of the amide substrate. Slide 28. Chymotrypsin Mechanism Step 2 In step 2, the first tetrahedral intermediate is formed. This intermediate is unstable because of its anionic character (negative charge on the oxygen). That anionic intermediate is stabilized by charged groups in the “anionic hole” of the enzyme’s active site. This stabilization lowers the energy of activation and speeds the rate of reaction. The enzyme is providing an alternate reaction mechanism that has a reduced energy of activation. 8 BioC 3021 Notes Robert Roon Slide 29. Chymotrypsin Mechanism Step 3 In step 3, the tetrahedral intermediate breaks down, cleaving the amine product from the rest of the substrate. The portion of the molecule that remains covalently bound to the active site serine corresponds to the carboxylic acid part of the substrate. This is the acylenzyme intermediate that is much more stable than the anionic intermediates. Under certain conditions, serine proteases can be isolated with the acylenzyme intermediate covalently attached to the enzyme’s active site. Slide 30. Chymotrypsin Mechanism Step 4 In step 4, the amine product picks up a proton from the active site histidine and diffuses away. In this reaction, the histidine is acting as an acid (proton donor). Slide 31. Chymotrypsin Mechanism Step 5 In step 5, there is a nucleophylic attack of a hydroxyl ion on the acylenzyme intermediate. The production of the hydroxyl ion from water in the active site is promoted by the active site histidine, which acts as a base (proton acceptor) to remove the proton from the water molecule. Slide 32. Chymotrypsin Mechanism Step 6 In step 6, a second tetrahedral intermediate is formed. Similar to the chemistry of step 2, this intermediate is unstable because of its anionic character (negative charge on the oxygen). That anionic intermediate is again stabilized by charged groups in the “anionic hole” in the enzyme’s active site. This is a second transition state intermediate whose energy of activation is reduced by interaction with the active site of the enzyme. Slide 33. Chymotrypsin Mechanism Step 7 In step 7, the second tetrahedral intermediate is attacked by a proton conveniently provided by the active site histidine residue. 9 BioC 3021 Notes Robert Roon The anionic oxygen is converted to a carbonyl, and the attachment of the intermediate to the active site serine is broken. Slide 34. Chymotrypsin Mechanism Step 8 In step 8, the carboxylate product diffuses away, and the enzyme reverts to its original state. Slide 35. The Oxyanion Hole The oxyanion hole is the place in the active site of chymotrypsin where a negatively charged oxyanion forms on the substrate. This oxyanion is a highly unstable intermediate in the mechanism of chymotrypsin, and it has a very high energy level. The figure shows how the tetrahedral anionic intermediate is stabilized by hydrogen bonding to two protons from peptide amino groups on the enzyme. This stabilization of the intermediate lowers the energy of the transition state. Slide 36. Occurrence of Other Serine Proteases There are many serine proteases that are homologs of chymotrypsin, including some of the enzymes involved in the blood clotting cascade. These enzymes have evolved from common evolutionary precursor proteins. These homologous proteases exhibit similarities in amino acid sequence, and have the same catalytic triad and oxyanion hole found in chymotrypsin. There are other serine proteases that are not homologs of chymotrypsin. That is, they have very different sequences and have evolved independently. The next two slides illustrate a catalytic triad and an oxyanion hole in the proteases, subtilisin and carboxypeptidase II. These enzymes are not homologs of chymotrypsin or of each other. Slide 37. Active Site of the Serine Protease Subtilisin This figure illustrates the occurrence of a catalytic triad and an oxyanion hole in the protease, Subtilisin. This enzyme is not a 10 BioC 3021 Notes Robert Roon homolog of chymotrypsin. Thus, the occurrence of these features in Subtilisin represents an interesting example of convergent evolution. Slide 38. Active Site of Carboxypeptidase II This figure shows that the same pattern of a catalytic triad and an oxyanion hole has also been observed in carboxypeptidase II. This enzyme is not significantly similar to either chymotrypsin or subtilisin. Slide 39. Inhibitor of the AIDS Protease This figure shows the structure of an important protease inhibitor, Indinavir, which is used in the treatment of AIDS. Indinavir inhibits the HIV protease produced by the AIDS virus. Indinavir resembles the peptide substrate for the HIV protease. Slide 40. Carbonic Anhydrase Reaction We will now turn our attention to a different enzyme, carbonic anhydrase. Carbonic anhydrase plays an important role in the removal of carbon dioxide from the human body. In red blood cells, the enzyme catalyzes the conversion of carbon dioxide to carbonic acid. This reaction proceeds at a moderate pace even in the absence of any enzyme, but the presence of carbonic anhydrase makes it an extremely fast reaction. Slide 41. Mechanism of Carbonic Anhydrase Carbonic Anhydrase catalyzes the condensation of carbon dioxide with water to form carbonic acid. The carbonic acid then dissociates to form bicarbonate ion plus a proton. Slide 42. The Active Site of Carbonic Anhydrase Contains a Zinc Ion Carbonic anhydrase contains a zinc ion that is essential for catalytic activity. The zinc ion is bound to the imidazole rings of three histidine residues. 11 BioC 3021 Notes Robert Roon Slide 43. The pH Activity Profile for Carbonic Anhydrase Carbonic anhydrase exhibits a pH optimum at about pH 8. The pH response for this enzyme is thought to reflect the interaction of the zinc ion with a water molecule in the active site. Slide 44. Water Binds to the Active Site Zinc Ion of Carbonic Anhydrase Interaction of water with the enzyme’s zinc ion lowers the pK of water from 15.7 to about 7.0. This results in the rapid production of hydroxyl ion. Slide 45. Mechanism of Carbonic Anhydrase The hydroxyl ion formed on the carbonic anhydrase zinc ion can act as a nucleophile. The hydroxyl group attacks carbon dioxide and converts it into a bicarbonate ion. 12