Lecture 07 Microbiology PDF
Document Details
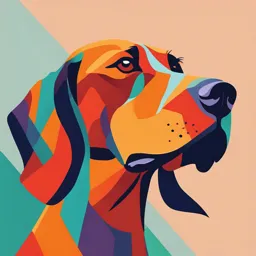
Uploaded by GoldenDeciduousForest
Tags
Summary
This document is a lecture on microbiology, specifically focusing on the genetics of bacteria and archaea, and how these drive microbial evolution. It covers topics like mutation, gene transfer, mobile DNA elements, and different types of mutations like missense and nonsense mutations.
Full Transcript
BIOL371: Microbiology Lecture 7 – Genetics of bacteria and archaea (driver of microbial evolution) 1 Topics of today 1. Mutation 2. Gene transfer 3. Mobile DNA elements Materials covered: Chapter 9.1-9.11 Figures 9.1, 9.3-9.6, 9.10-9.13, 9.18, 9.20, 9.22-28, 9.32-35 Tables 9.1, 9.2 2 Ov...
BIOL371: Microbiology Lecture 7 – Genetics of bacteria and archaea (driver of microbial evolution) 1 Topics of today 1. Mutation 2. Gene transfer 3. Mobile DNA elements Materials covered: Chapter 9.1-9.11 Figures 9.1, 9.3-9.6, 9.10-9.13, 9.18, 9.20, 9.22-28, 9.32-35 Tables 9.1, 9.2 2 Overview of bacterial and archaeal genetics Bacteria and archaea can exchange genes Mutation: heritable change in genome Can lead to a change in properties of an organism Some beneficial, some detrimental, most have no effects Spontaneous mutations at low rate; but exponential growth in prokaryotes accumulates mutations quickly Horizontal gene transfer (genetic exchange) generates much larger changes than mutation Mutation and genetic exchange drive evolution 3 Mutations and mutants Composition of genetic material Prokaryotic and eukaryotic cells: double-stranded DNA Viruses: double- or single-stranded DNA or RNA Wild-type strain: isolated from nature “wild-type” can also refer to a single gene Mutant: a cell derived from wild type that carries a nucleotide sequence (genotype) change Genotype in bacteria is designated by three lowercase letters followed by capital, all italicized (e.g., hisC involved in histidine biosynthesis) Mutations designated hisC1, hisC2, etc. or ΔhisC Observable properties (phenotype) may also be altered Phenotype in bacteria is designated by capital letter followed by two lowercase letters, then +/– (e.g., His+ makes histidine while His– strain cannot synthesize histidine) 4 Isolation of mutants: selection vs screening Selectable mutations confer an advantage Under certain environmental conditions, progeny cells outgrow and replace parent; e.g., antibiotic resistance Relatively easy to detect Powerful genetic tool Non-selectable mutations do not confer an advantage even though they may lead to a phenotypic change; e.g., color loss in a pigmented organism Requires laborious, time-consuming screening (examining large numbers and looking for differences) 5 Examples of common classes of mutants and detection Phenotype Nature of change Auxotroph Loss of enzyme in biosynthetic pathway Temperaturesensitive Cold-sensitive Drug-resistant Rough colony Pigmentless Sugar fermentation Virus-resistant Detection of mutant Inability to grow on medium lacking the nutrient Alteration of an essential protein so it is Inability to grow at a high temperature that more heat-sensitive normally supports growth Alteration of an essential protein so it is Inability to grow at a low temperature that inactivated at low temperature normally supports growth Detoxification of drug or alteration of Growth on medium containing a normally drug target or permeability to drug inhibitory concentration of the drug Loss or change in lipopolysaccharide Granular, irregular colonies instead of layer smooth, glistening colonies Loss of enzyme in biosynthetic pathway Presence of different color or lack of color leading to loss of one or more pigments Lack of color change on agar containing Loss of enzyme in degradative pathway sugar and a pH indicator Growth in presence of large amounts of Loss of virus receptor virus Method Screening Screening Screening Selection Screening Screening Screening Selection 6 Isolation for nutritional auxotrophs by screening Auxotroph has an additional nutritional requirement for growth compared with prototroph (wild type/parental strain); e.g., His– vs His+ Replica plating screens for nutritionally defective mutants Transfer colonies from master plate Inability of colony to grow medium lacking a nutrient indicates mutation Colony on master plate is picked, purified, and characterized 7 Mutations and mutation rates Mutation rates depend on frequency of DNA changes and efficiency of DNA repair Spontaneous mutations: occur without external intervention Result mainly from errors during DNA replication Mutation rates of spontaneous mutations Prokaryotes: 10–6 to 10–7 per kilo base pairs (kbp) Eukaryotes: 10–8 per kbp; 10-fold lower than prokaryotes DNA viruses: 10–4 to 10–5 per kbp RNA viruses: higher mutation rates than DNA viruses, lack of RNA repair mechanisms and less proofreading Induced mutations: caused environmentally or deliberately Result from exposure to radiation or chemicals that modify DNA Mutation rates depend on exposure doses 8 Base-pair substitutions Not all mutations change polypeptides due to codon degeneracy Silent mutations: do not affect polypeptide sequence or phenotype Missense mutation: change sequence of amino acids in polypeptide Not all missense mutations lead to phenotypic changes (dysfunction or loss of function) If occurs at a critical site, could lead to changes in function (phenotypic change) Nonsense mutation (stop codon) Typically results in truncated (incomplete) protein that lacks normal activity 9 Frameshift, insertion, deletion mutations Frameshift mutations: single or two base-pair deletions or insertions leading to a shift in reading frame Scrambles entire polypeptide sequence downstream Deletion/insertion of three base pairs deletes/add a codon (amino acid); not as bad, often no phenotypic change Insertions/deletions: gain/loss of large segments of DNA Often complete loss of function of one or more genes May arise from errors in recombination Transposable elements may cause large insertions 10 Reversions and suppressors Revertant: strain in which original phenotype is restored True revertant (same-site revertant): restore original sequence Suppressor mutation (second-site revertant): mutation at a different site in the genome that restores the original phenotype Mutation somewhere else in the same gene that restores function Mutation in another gene that restores function Mutation in another gene that results in production of an enzyme to replace a nonfunctional one 11 Mutagenesis: chemical and physical mutagens Agent Action Base analogs 5-Bromouracil Incorporated like T; occasional faulty pairing with G 2-Aminopurine Incorporated like A; faulty pairing with C Chemicals that react with DNA Nitrous acid Deaminates A and C Hydroxylamine Reacts with C Alkylating agents Monofunctional (e.g., ethyl methanesulfonate) Puts methyl group on G; faulty pairing with T Bifunctional (e.g., mitomycin, nitrosoguanidine) Cross-links DNA strands; faulty region excised by DNase Intercalating agents Acridines, ethidium bromide Inserts between two base pairs Radiation Ultraviolet (U V) Pyrimidine dimer formation Ionizing radiation (for example, X-rays) Free-radical attack on DNA, breaking chain 12 DNA repair and the SOS system In Bacteria, stalled replication or major DNA damage activates the SOS repair system Initiates many DNA repair processes, some of which are error-free Also allows DNA repair without a template by random incorporation of dNTPs resulting I many mutations Regulators are LexA (repressor) and RecA (normally used for recombination) proteins Fluorescently labelled repair protein localized to nucleoid after DNA damage DNA repair proteins 13 Horizontal (lateral) gene transfer in bacteria Horizontal gene transfer: gene movement between cells that are not direct descendants Allows quick acquisition of new characters Fuels metabolic diversity Three mechanisms: Transformation Transduction Conjugation Three possible fates: Degradation Replication by itself Recombination with host genome 14 Recombination: physical exchange of DNA Homologous recombination: process that results in genetic exchange between homologous DNA from two different source Endonuclease nicks one strand of donor molecule Helicase separates nicked from other strand Single-stranded segment binds single-strand binding protein and RecA Strand invasion: Base pairing displaces other strand of recipient DNA Creates recombination intermediates with heteroduplex regions where each strand is from a different chromosome Strands are separated by enzymes that cut and rejoin the previously unbroken strands of both the original DNA 15 Effect of homologous recombination on genotype In transformation, transduction, or conjugation, only part of the donor chromosome enters the recipient cell Recombination must take place with recipient chromosome or donor DNA will be lost To generate new genotypes, the homologous sequences must be related but distinct To detect exchange of DNA, recombinant cells must phenotypically different from both parents Complementation occurs when a functional wild-type copy is supplied, restoring wild-type phenotype Recombinant can be detected by use of selective medium 16 Transformation competence: involvement of pili Transformation: process of genetic transfer by which free DNA (e.g., from cell lysis) is incorporated into a recipient cell and brings about genetic exchange Typical transformable size: <10 kbp or ~10 genes Competence in transformation: ability to take up DNA and be transformed In some bacteria, DNA binds to pili Retraction of pilus (depolymerisation of pilins) brings DNA to periplasmic space or cytoplasmic membrane Com system: competence system, a multi-protein complex that brings the DNA to the cytoplasm 17 Regulation of transformation Plasmid DNA must remain double-stranded for replication, naturally transformable bacteria transform plasmid poorly In naturally transformable bacteria, competence is regulated by factors including quorum sensing and catabolite repression High-efficiency natural transformation is rare in bacteria Transformation competence can be boosted by specific procedures ((e.g., heat/chill treatment of E. coli) Electroporation: high-voltage electrical pulses can force cells to take up DNA 18 Transduction Transduction: transfer of DNA from one cell to another by a bacteriophage Generalized transduction: DNA from any portion of the host genome can be packaged inside the virion Donor genes cannot replicate independently Will be lost without recombination Specialized transduction: DNA from a specific region of the host chromosome is integrated directly into the virus genome, typically replacing some viral genes Homologous recombination may occur or may be integrated during lysogeny Occurs in many Bacteria and at least one Archaea Examples: multiple-antibiotic-resistance genes in Salmonella, Shiga-like toxins in Escherichia coli, virulence factors in Vibrio cholerae, photosynthetic genes in cyanobacteria 19 Generalized transdcution Host DNA accidentally packaged into phage forming transducing particles that is defective and cannot lead to viral lytic infection Upon lysis, transducing particles and normal virions released Small number of cells receives transducing particles that may recombine DNA Low frequency: one in 106 to 108 cells transduced 20 Lysogeny and specialized transduction Extremely efficient transfer Selective and transfers only small part of bacterial chromosome Lysogen: phage genome is integrated at specific site (example in figure, Lambda in E. coli: next to galactose utilization genes) Viral replication is under control of bacterial host chromosome Upon induction, viral DNA separates via process that reverses integration Sometimes excises incorrectly and takes adjacent host genes along with it, which can be transferred to another cell Limit to amount of host DNA that can replace phage DNA, but helper phage can assist 21 Phage conversion Phage conversion: alteration of the phenotype of a host cell by lysogenization When a normal (non-defective) phage lysogenizes a cell and becomes a prophage, the cell becomes immune to further infection by the same phage Selective value for host because of resistance to further similar infection Many bacteria isolated from nature are lysogens, and their corresponding prophage encode an abundance of transposons, suggesting that they: Enhance the survival of the lysogens Contribute to the formation of new genes 22 Conjugation (mating) Conjugation (mating): Horizontal gene transfer that requires cell-to-cell contact Plasmid-encoded Occurs between closely related or distantly related cells Donor cell (F+ cell): contains conjugative plasmid Recipient cell (F– cell): does not contain plasmid Other genetic elements (e.g., other plasmids or host chromosome) may be transferred during conjugation Following conjugation, both donor and recipient cell are F+ 23 F (fertility) plasmid ~99 kbp circular DNA molecule Contains genes that regulate DNA replication Also contains transposable elements that allow the plasmid to integrate into the host chromosome Contains tra genes that encode transfer functions Synthesis of sex pilus and DNA transfer system Pili allow specific pairing through receptor contact, pulling cells together, and DNA transferred through junction Presence of F plasmid results in three changes: Ability to synthesize F pilus Mobilization of DNA for transfer to another cell Alteration of surface receptors so that cell can no longer be a conjugation recipient 24 Transfer of plasmid DNA by conjugation DNA synthesized by rolling circle replication Transfer triggered by cell-cell contact Plasmid DNA nicked by TraI enzyme and DNA is replicated in both donor and recipient cell Transfer of F plasmid is fast, ~5 minutes 25 Process of conjugation – animation 26 Hfr (high frequency of recombination) strain F plasmid is an episome (plasmid that can integrate into host chromosome) When integrated, chromosomal genes can be transferred with plasmod F+: cells possessing a non-integrated F plasmid Hfr: cells possessing an integrated F plasmid High rates of genetic exchange between genes on the donor (Hfr) and recipient (F–) chromosomes Insertion sequences (ISs; mobile genetic elements) are present in both the F plasmid and E. coli chromosome Homologous recombination between ISs results in F plasmid integration 27 Transfer of chromosomal genes by an Hfr strain After integration, tra functions normally and Hfr strain synthesizes pili When recipient cell is encountered, part of plasmid and some chromosomal genes are transferred Recipient cell does not become Hfr or F+ because only part of F plasmid transferred 28 Hfr conjugation – animation 29 Formation of different Hfr strains and F’ strains F plasmid can be inserted into various insertion sequences on the bacterial chromosome, forming different Hfr strains Occasionally integrated F plasmids may be excised from chromosome, some carrying chromosomal genes F’ (F prime) plasmids are F plasmids containing chromosomal genes F’ plasmids transfer chromosomal genes to recipients at high frequency Establishes diploids for limited chromosomal region 30 Horizontal gene transfer in archaea Genetic manipulation in archaea is less developed than in bacteria Most antibiotics do not work on archaea, so limit the choice of selectable markers No single model organism in archaea in contrast to bacteria where E. coli is a model Transformation, transduction (rare), conjugation, and plasmids are found in archaea 31 Mobile DNA: transposable elements Mobile DNA: Discrete segments of DNA that move as a unit from one location to another within other DNA molecules Most mobile DNA are transposable elements (stretches without their own origin of replication) Move by transposition Extremely abundant and found in all three domains of life, many viruses, and plasmids Two main types of transposable elements in Bacteria are insertion sequences (ISs) and transposons Encode transposase (enzyme required for transposition) Have short inverted repeats at ends also required for transposition 32 Insertion sequences and transposons Insertion sequences: simple transposable elements ~1000 base pairs long Inverted repeats are 10-50 base pairs Carry only one gene, which encodes transposase (enzyme for transposition) Found in chromosomes and plasmids of bacteria and archaea and in bacteriophages Transposons: larger than ISs Similar to ISs, contain inverted repeats and transposase gene Genes inside vary widely; e.g., antibiotic resistance in Tn5 and Tn10 33 Mechanisms of transposition Transposase recognizes, cut, and ligates DNA When inserted, a short sequence in target DNA at integration site is duplicated Conservative transposition: transposon is excised from one location and reinserted at a second location Replicative transposition: a new copy of transposon is produced and inserted at a second location 34 Utility of transposon mutagenesis Transposon insertion alters DNA, resulting in mutation Powerful tool for creating mutants to study gene function Transposon carrying antibiotic-resistance genes are used Transposon is introduced by conjugation or transformation on a plasmid that cannot be replicated in the cell (forcing the plasmid to integrate into the chromosome) Since microbial genomes are compact with genes close together, most insertions will be in genes that encode protein 35 Example of transposon mutagenesis A transposon conferring kanamycin resistance is added to a culture of wildtype cells Selection of integration of the transposon using the antibiotic kanamycin Screen mutants for ability to form biofilm Using primers specific to the transposon to sequence the flanking region of the insertion site 36